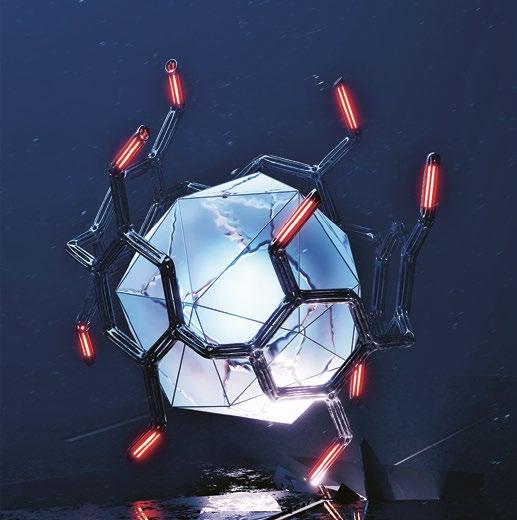
5 minute read
The path of least resistance
Christopher Jonkergouw is no stranger to languages. His native tongue is Dutch, we are talking in English, and most of his colleagues at Aalto University speak Finnish. Even in his spare time, he plays alongside several nationalities for Espoo rugby club.
However, as a biologist, it’s the ‘language’ and communication of bacteria that he’s particularly interested in.
Bacteria don’t talk, of course, but they do use a form of chemical signalling to pass messages to each other when infecting the human body. ‘Some of the most pathogenic, problematic bacteria utilise signalling molecules very heavily to establish successful infections,’ Jonkergouw explains. ‘A lot of pathogenic processes are directly related to this signalling.’
What if we could disrupt those bacterial communication lines?
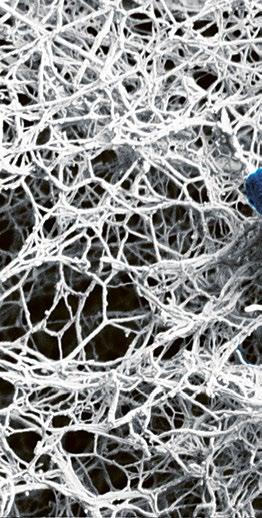
It’s a question that Jonkergouw and his colleagues are now exploring. Not only would it make infections less likely to spread, but it could help tackle one of the grandest challenges facing the world: antimicrobial resistance.
The dangers of antimicrobial resistance
In 1945, the Scottish physician Alexander Fleming delivered a lecture in Stockholm to accept his Nobel Prize for the discovery of penicillin. It was a celebratory speech, but towards the end, he made a prescient forecast about trouble ahead.
‘There may be a danger,’ he warned, ‘in underdosage. It is not difficult to make microbes resistant to penicillin in the laboratory by exposing them to concentrations not sufficient to kill them, and the same thing has occasionally happened in the body.’
In recent years, antimicrobial resistance has caused a ‘stealthy and silent’ pandemic which may not have led the news but has killed millions of people. According to research published in The Lancet in January 2022, it was directly responsible for the deaths of 1.27 million people in 2019, and by some estimates, this number could rise to 10 million deaths per year by 2050.
‘It's kind of an arms race between bacteria and the development of new treatments,’ explains Jonkergouw. But sadly, it’s a race we’re losing. As antibiotic-resistant encounters have risen rapidly, the development of novel therapies has fallen far behind. The number of new antibiotics in trials – as tracked by the World Health Organization – is worryingly small.
In part, this is because ‘it's expensive to make a new drug,’ explains Douglas Häggström of INCATE, the INCubator for Antibacterial Therapies in Europe, which helps universities commercialise research to tackle the antimicrobial resistance problem. But it's more complex than just the cost.
Companies know that even if a new antibiotic makes it through expensive clinical trials, nowadays doctors may use it only as a treatment of last resort. ‘Because resistance develops at a population level, you don't want to prescribe these drugs very often,’ Häggström explains. Ten years later, the patent expires, and the company’s hope of recouping their investment dries up.
In parallel, ‘the science got really hard,’ Häggström continues. The low-hanging fruit have gone. ‘Historically, there have been lots of natural products, but we’ve found most of the things in nature you can find.’
The good news is that alternative approaches are in the works. These include vaccines, immunotherapies, viruses called phages that can infect and kill the bacteria, and therapies that disarm the weapons they use to establish and spread their infection.
It’s this last strategy that Jonkergouw and his colleagues are exploring. What makes their approach novel and interesting is that it doesn’t target the bacteria themselves but instead focuses on their so-called virulence factors.
What are virulence factors?
To understand how the team’s approach works, you first need to know about how bacteria thrive during an infection. The term ‘virulence factors’ collectively includes both offensive and defensive bacterial weapons.
Defensive weapons include materials like biofilm, which is a protective matrix that bacteria produce so they can clump together in communities. ‘In practice, what it looks like is just a slimy mess: a whole range of complex polymers from bacterial pathogens,’ explains Jonkergouw. Biofilm helps shield the community from threats, such as our immune defences or antibiotics.
Offensive weapons include bacterial toxins, such as lipopolysaccharides. ‘Bacteria use these toxins to weaken a patient’s defences – your immune system – and create this competitive advantage for themselves,’ says Jonkergouw. ‘In those conditions, they can survive just fine, but you cannot, and this creates huge inflammatory responses and cell death.’
If a therapy could disrupt these weapons, it would pave the way for the immune system or antibiotics to completely wipe out the microbes before they can develop resistance.
Disrupting communication
To deploy virulence factors, bacteria need to communicate.
They do this by producing signalling molecules which can be sensed by other bacteria. If a large enough quorum is reached, the community can collectively spend resources on the energy-intensive production of toxins and biofilms. This group behaviour increases the chances of a successful infection. Bacteria may be unicellular, but they find strength in numbers.
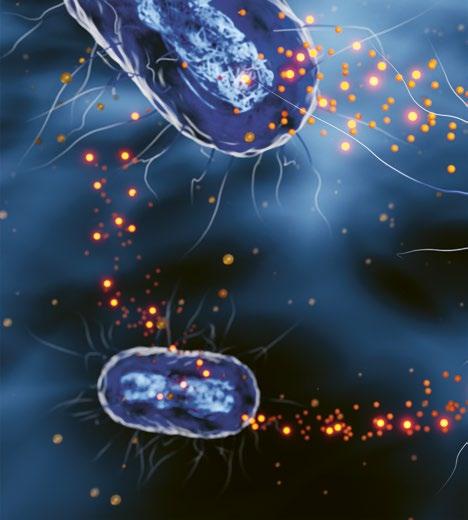
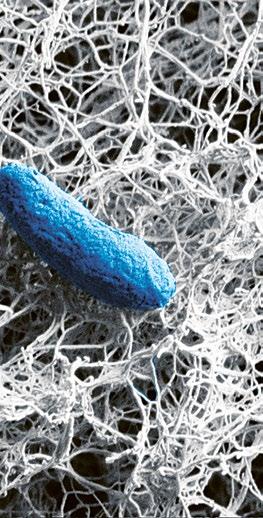
The way in which bacteria communicate forms the core of Jonkergouw’s approach. ‘A couple of years ago, we explored molecules in the lab that can interfere with this communication,’ he explains. The team realised that, hypothetically, interfering with communication could also help against the World Health Organization's ‘top priority’ resistant pathogens Acinetobacter baumannii and Pseudomonas aeruginosa. ‘However, when you try something like that in research, usually it never ever works,’ he laughs. ‘But here we found that it did work very effectively, and it works against the most problematic pathogens.’
Since then, the research team has been further exploring ways to disrupt bacterial communication and thus mitigate virulence factors. Along with his Aalto colleague Ekaterina Osmekhina, Katarzyna Leskinen of the University of Helsinki, and drug development veteran Tuula Heinonen, Jonkergouw is now developing the findings into a potential therapy through a spin-off company called Arivin Therapeutics.
Arivin is focusing on therapies for cystic fibrosis, chronic obstructive pulmonary disease (COPD) and other respiratory diseases. The team expects that bacteria won’t rapidly develop resistance to the new treatment, unlike antibiotics. Since disrupting the bacteria’s communication doesn’t kill them, there isn’t strong pressure on the pathogens to evolve.
The treatment is also effective against superbugs – bacteria that are already highly resistant to antibiotics –which are becoming increasingly prevalent due to our heavy dependence on the drugs.
Jonkergouw and the team acknowledge that the company has some way to go before the therapy will be ready as a treatment – but it’s been a strong start, and the science looks promising. Arivin was among the first companies selected by the INCATE incubator, alongside a small group of other promising European companies working on antimicrobial therapies.
Getting clever
In the coming years, the problem of antimicrobial resistance is only going to get more severe, so we’re going to need every new approach we can find.
However, if Alexander Fleming were alive today, the Nobel-winner would no doubt applaud the myriad new ideas and techniques that are emerging to tackle the issue, including efforts to cut off how they communicate.
If Arivin’s therapy can disrupt bacterial virulence, it won't stop the problem of resistance altogether, but it could certainly slow it down. We're in a race with bacteria – a race in which we're falling behind – and techniques like these could help us finally begin to catch up.