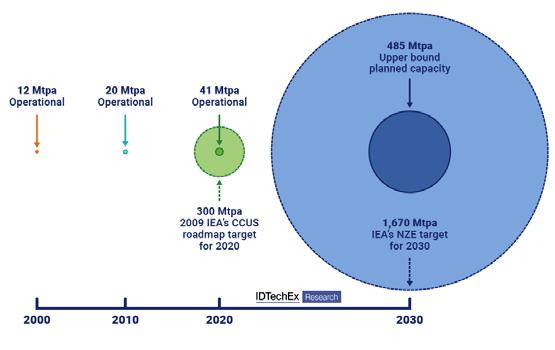
11 minute read
TECHNOLOGY
A NEW DAWN FOR CCUS
After five decades of ups and downs
Advertisement
Carbon Capture, Utilisation and Storage (CCUS) is recognised as one of the key technologies to achieve global climate goals, tackling emissions from energy and industrial sectors, as well as removing CO₂ from the atmosphere. But, despite the critical importance of the technology and substantial development to date, CCUS deployment has been slower than anticipated.
BY DR HYDRA RODRIGUES, IDTechEx
Regardless of the near fourfold growth in the past 20 years, the current deployment of CCUS is still limited. CCUS capacity growth has been around 2.3-million tons per annum (Mtpa) of added capacity since 2010, with annual capture capacity reaching 41Mtpa of CO₂ in 2020. This is 86% short of the ambition the International Energy Agency (IEA) set a decade prior. The current global CO₂ capture capacity is equivalent to 0.1% of overall emissions.
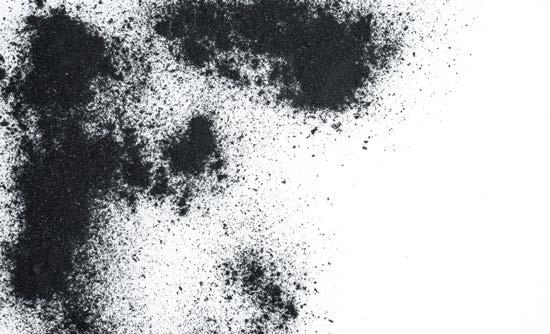
Global CO₂ capture capacity (in Mtpa) operational, announced and expected by year. Note: the radius of each circle has been scaled based on the capture capacity it represents. Dashed circles represent expected capacity.
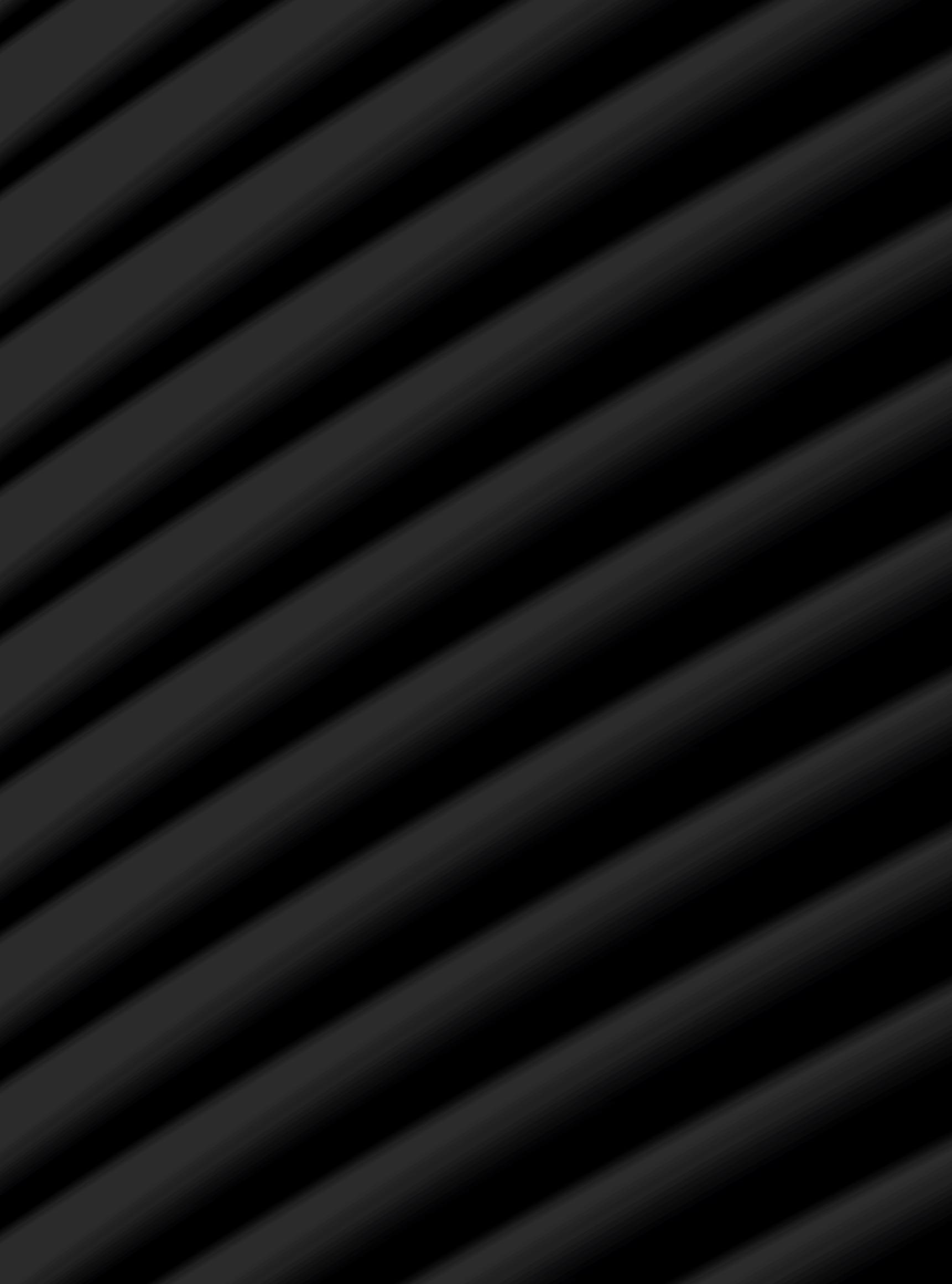
After years of a declining investment pipeline, there has been significant acceleration in the sector for the past two years, with multiple announcements of new integrated CCUS facilities. Most of these are in North America and Europe, but projects are also planned in Australia, China, Korea, the Middle East and Chile. Still, the industry needs to grow more than 35-fold by 2030 to reach the capture capacity required for net-zero emissions as per the IEA’s Net-Zero Emissions (NZE) by 2050 Scenario (IEA, 2021).
The first industrial large-scale CCUS project commenced operation about 50 years ago at the Val Verde natural gas processing plant in Texas, US. Instead of being vented, the CO₂ captured was compressed and transported through a pipeline and then injected into the SACROC field for Enhanced Oil Recovery (EOR). Natural gas processing plants supplying CO₂ for EOR became the standard of the CCUS industry, with some of the largest integrated CCUS projects in the world being a combination of these two Europe was the second continent to see CCUS projects appearing, with Norway’s Sleipner project for dedicated storage purposes in 1996.
THE IMPORTANCE OF CCUS
CCUS refers to the set of technologies that strip carbon dioxide (CO₂) from waste gases and directly from the atmosphere, before either storing it underground or using it for a range of industrial applications. The goal is to prevent CO₂ emissions from accumulating in the atmosphere, which is one of the main causes of global warming.
After a plateau during the 2000s, the number of CCUS projects in operation has taken off since 2010, but the CCUS industry saw highprofile project cancellations and government funding programs that failed to deliver. IDTechEx’s research indicates that more than 60% of proposed projects have been cancelled on average, notably in the power sector. The failure of the Kemper County carbon capture facility in 2017 and the mothballing of the CCS retrofit unit at the Petra Nova plant in Texas in 2020 have brought into question the CCUS industry’s ability to deliver large-scale projects that are profitable and low carbon. Delays have also been commonplace.
Several factors contributed to these obstructions, but they were mostly due to economic reasons (fluctuating markets, insufficient financial support), technical issues (flow assurance, solvent degradation, insufficient storage capacity), and lack of public acceptance. Additionally, as the western world has moved away from coal, many large-scale projects of coal power plants that were meant to be fitted with CCS capabilities never came to fruition.
In the last decade, the CCUS industry focus shifted from power towards industries considered “hard to decarbonise” (cement, iron and steel industries), the emerging hydrogen economy as well as new negative emission technologies (NETs).
MOMENTUM BUILDING
There has been great momentum behind CCUS in 2022, with projects, new policies and funding announced. Building up to this year’s developments, the UN’s Climate Change Conference (COP26) in 2021 reinvigorated net-zero targets and fostered multi-country partnerships in CCUS. That same month, the US passed the Infrastructure Investment and Jobs Act, providing a combined US$15-billion to support CCUS and low-carbon hydrogen production.
TRANSITIONAL TECHNOLOGY
In many industries, CCUS is considered a transitional option to get carbon-intensive assets to their end of life or to serve as a steppingstone to more sustainable technologies that are still under development. Ultimately, CCUS can create a demand for captured CO₂ until carbon prices are sufficiently high. CO₂-EOR has been an on-ramp for CCS and, more recently, for DAC and may continue to do so alongside other emerging uses of CO₂ as a feedstock for chemicals, fuels and building materials.
Source: IDTechEx
KEY CCUS APPLICATIONS
• Hard-to-abate sectors with inherent process emissions such as cement, iron and steel, and chemicals. Renewable fuel switching and efficiency improvements will not be enough to decarbonise these industries. • Blue hydrogen production, where CCUS safely disposes of the
CO₂ produced during traditional steam methane reforming. Blue hydrogen is set to play a key role in creating new markets for hydrogen as a clean energy carrier, acting as a bridge for green hydrogen once electrolyser capacity catches up. • e-Fuels production, where CO₂ from emissions can be converted into hydrocarbons using renewable energy. e-Fuels can take care of legacy internal combustion engine vehicles while electric vehicles gain market share and of long-haul transportation (planes, ships, trucks), while battery technology advances and becomes lighter.
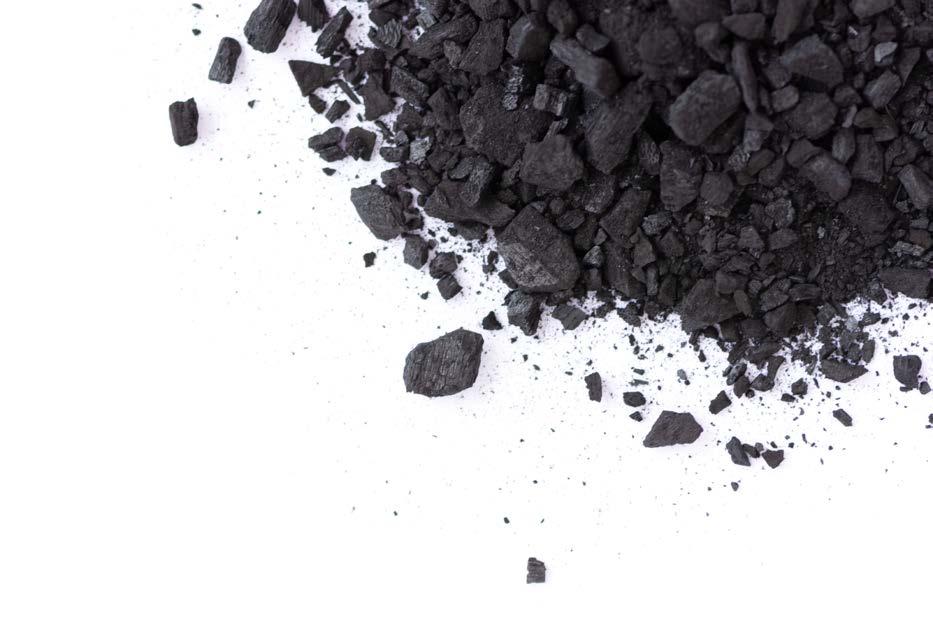
CARBON DIOXIDE REMOVAL
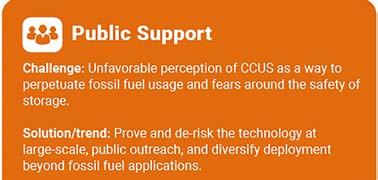
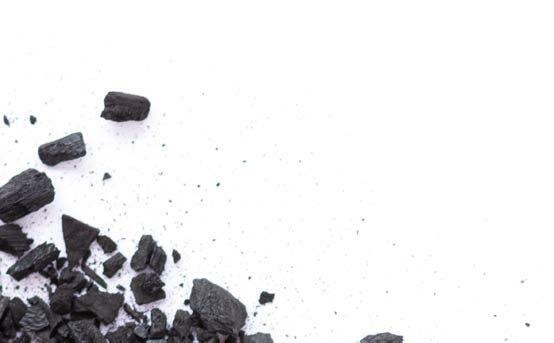
While carbon capture may play a crucial role in the energy transition, carbon dioxide removal (CDR) may be needed beyond that. CDR is present in every credible, long-term climate scenario to take and maintain the world at the net-zero level of emissions. Given its potential, there has never been more focus on removing CO₂ from the atmosphere using NETs such as direct air capture (DAC). Venture capitalists have poured more than US$1-billion into the nascent DAC industry in 2022, with the biggest slice of the pie going to Climeworks’ latest funding round worth US$650-million: more than the total amount invested in DAC in the past five years.
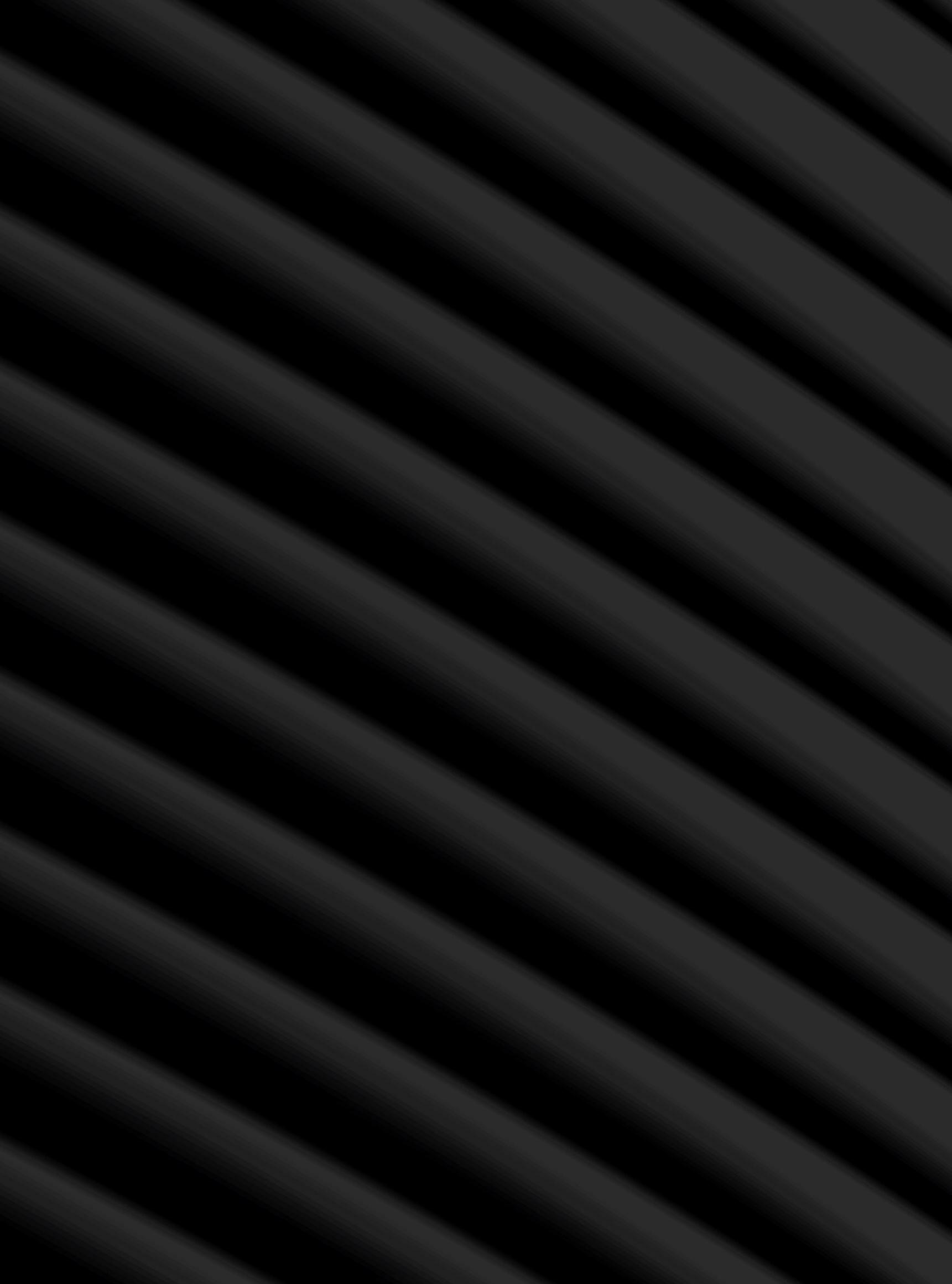
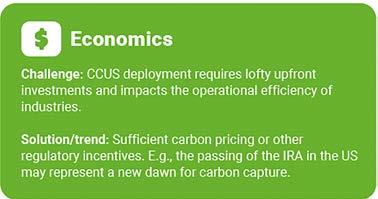
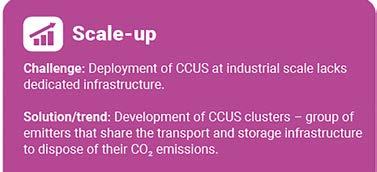
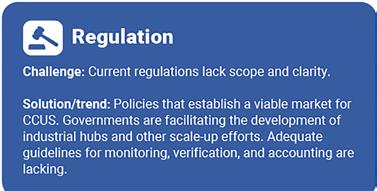
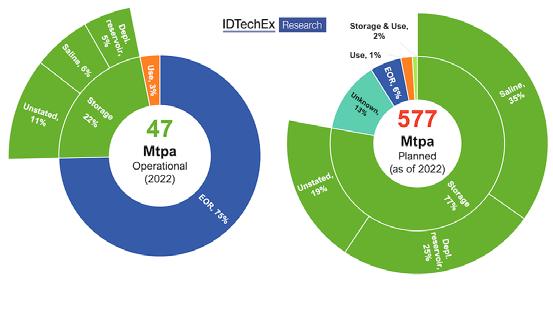
Global outlook of CCUS capacity by CO2 endpoint: operational in 2022 (left) and announced to date (right). Challenges in CCUS.
DAC players are racing to drive the cost down to US$100 per net ton of CO₂ removed and to reach the gigatonne scale of annual removals. Both milestones are complementary, as scale and cost will likely evolve in tandem. Standardising a robust methodology for monitoring, reporting, and verification (MRV) of carbon removals will be essential to achieve those goals.
FUTURE PROSPECTS
The fundamental role of CCUS is to provide an infrastructure capable of securely and permanently isolating millions of tons of CO₂ away from the atmosphere. CCUS will be critical in enabling the level of decarbonisation required to achieve the net-zero emissions targets stipulated in the Paris Agreement. But for this, it needs to scale.
Despite the fair level of maturity, carbon capture technologies remain costly, energy-intensive and underdeveloped. Questions remain around the willingness of governments to ensure a proper price for carbon through cap-and-trade or other incentive schemes and of investors to financially support large-scale CCUS projects. Meeting CCUS’ ambition to slow down climate change requires a step-change in policy and coordinated global action that is shaping up, but it is yet to be fully realised.
Merits of ASSESSING CARBON IMPACTS early
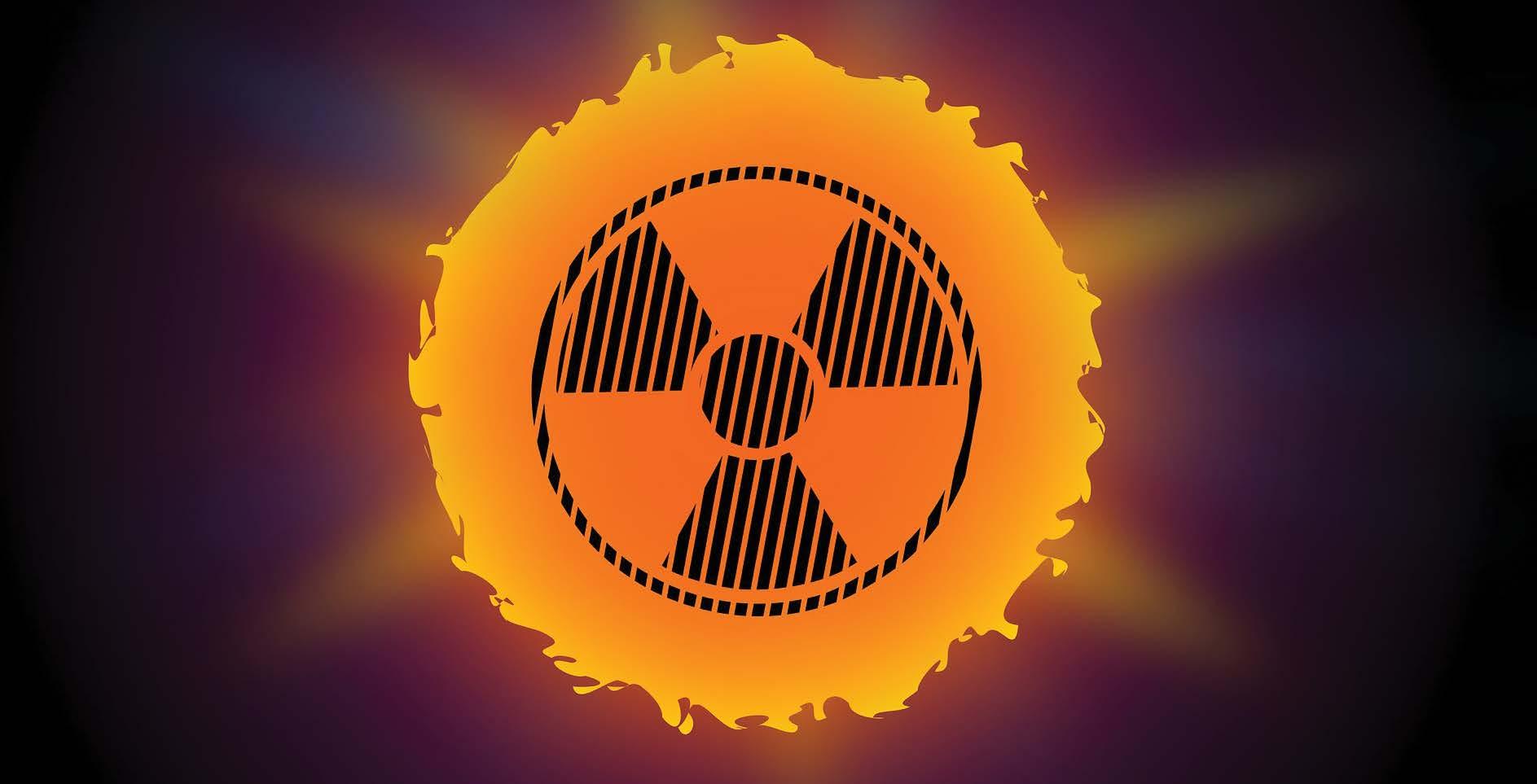
Under increasing pressure and incentives to reduce their carbon footprint, projects in the infrastructure and industrial space need to start early in their lifecycle to find optimal mitigation and adaptation strategies.
BY SRK CONSULTING
Philippa Burmeister, associate partner and principle scientist, SRK Consulting.
Speaking at the International Association for Impact Assessment (IAIA) symposium in Cape Town recently, SRK associate partner and principal scientist Philippa Burmeister highlighted that an options analysis at project concept stage is vital.
“This allows developers to consider alternative layout, equipment, processes and technologies,” Burmeister said. “These options could contribute significantly to reducing greenhouse gas (GHG) emissions.”
Choices could include more efficient or low energy technologies, specialised electric vehicles or reduced travel distances. There are also replacement options to consider, which open possibilities to consider various energy options – from traditional to renewable sources.
“Considering alternatives and designing for reductions upfront enable reductions with potential economic benefits,” she explained. “Once infrastructure is in place without such planning, retrofitting alternative and technology is substantially more costly and difficult.”
For waste management facilities such as landfill sites, for instance, methane created by waste degradation is a powerful GHG that can be captured. If this option is embraced at concept stage, with the appropriate design and budget, GHG emissions at a landfill can be reduced while also creating a valuable energy product.
The opportunities to apply decarbonisation strategies to a project decrease substantially once it evolves beyond concept and design stage. Once prefeasibility or feasibility stage is reached, the priority is to accurately quantify the alternative options for reducing GHG emissions. This includes developing an inventory of GHG emissions for the project and identifying their most significant sources.
“Alternatives can then be proposed for the most significant sources,” she said. “Using a GHG emissions inventory, project developers can demonstrate reductions in carbon intensity as a result of alternatives.” This is a vital step considering GHG risks such as carbon taxes and energy cost increases.
“By the time the construction phase is reached, most clients do not have control over related emissions,” added Burmeister. “All they can do is to give preference to carbon-conscious contractors and suppliers, during their selection. They can choose companies who have demonstrated commitment to reduce their own carbon footprints.”
In the construction tender documentation, for example, GHG reporting and continual improvement can be included. There is also some scope to further reduce emissions in selecting energy efficient equipment alternatives like air-conditioner models or LED light bulbs.
At operations stage, the annual reporting of the GHG emissions inventory is a positive way to reflect the achievements of emission reduction targets and identify opportunities for future reductions.
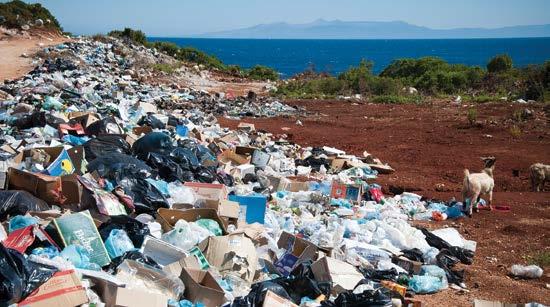
Methane created by waste degradation is a powerful GHG that can be captured in waste management facilities.
An options analysis at project concept stage is vital to reduce carbon from the outset of a project.
“As technology evolves and creates options for decarbonising, projects can consider retrofitting energy efficient or low emission equipment,” she said. “In most infrastructure or industrial processes, however, it is often impractical to completely replace key elements before the end of life. For existing infrastructure, the focus therefore is usually on the replacement of energy sources (from traditional to renewable) rather than on reduction strategies.”
At the closure stages – and even post-closure – there remain some opportunities to reduce carbon emissions. Carbon sequestration can also be considered, such as revegetating options that would capture the most carbon.
Burmeister pointed out that carbon taxes are driving many of the corporate efforts towards decarbonisation, but there are also growing ESG awareness and sustainability reporting requirements.
“Investors are commonly asking companies for GHG reporting and reduction planning,” she said. “The carbon intensity of products is also becoming a consideration by customers as it impacts the customers’ Scope 3 emissions reporting.” Increasingly, end-use consumers are also becoming aware of their carbon footprint and the carbon footprint of their preferred brands.
Projects need to consider not only their impact on the environment, but how the changing climate will have direct effects on the project itself. These will range from extreme weather conditions – causing flooding, drought, heat waves, heavy winds and fires – to socioeconomic impacts on nutrition and livelihood opportunities. Companies need to adapt by making the necessary preparations for how their staff, communities and supply chains will be affected.
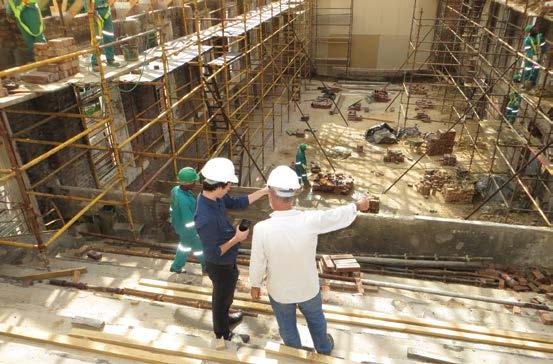
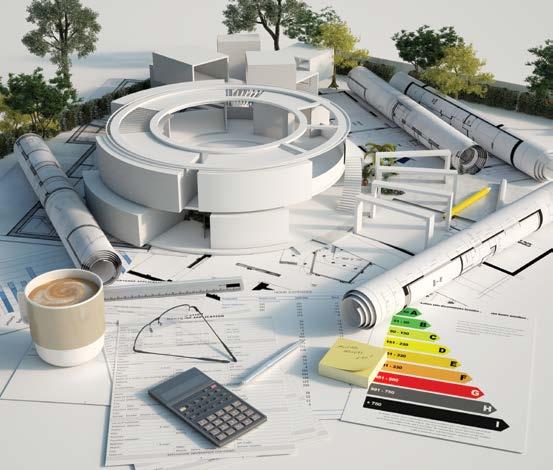
MITIGATION AND ADAPTION
Addressing the critical climate change issues of both mitigation and adaptation, SRK associate partner Philippa Burmeister made two presentations at the recent international of the International Association for Impact Assessment symposium. Under the theme Celsius 1.5, the event referenced the negative impacts forecast in relation to global temperatures rising 1.5 degrees above preindustrial levels.
In one presentation, authored jointly with SRK principal scientist Ashleigh Maritz, Burmeister addressed Decarbonisation through the project lifecycle. Initiatives to decarbonise operations are an important aspect of mitigating impact. At a preceding workshop on Climate Change and Urban Planning, she presented on the tools available to anticipate risk – as part of organisations’ efforts to adapt to climate change.