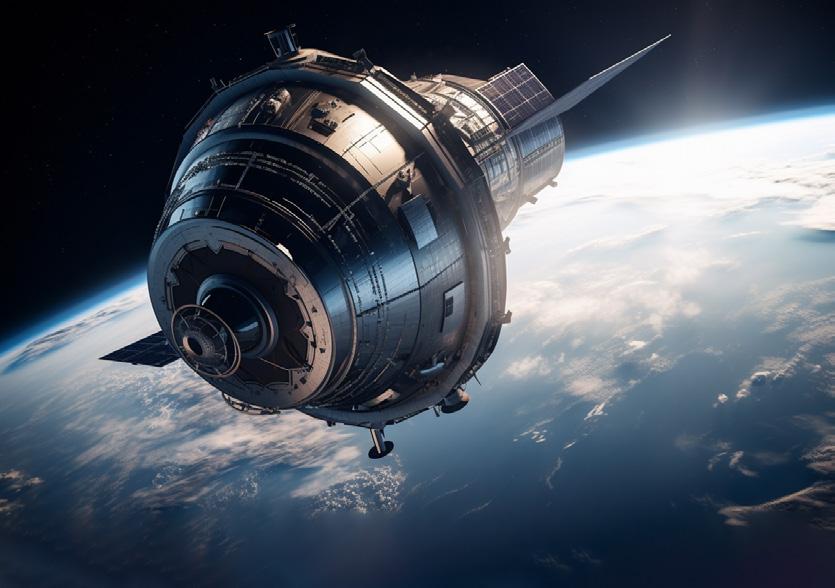
5 minute read
Building next generation autonomous space systems with AI
By Dr Carl Seubert SmartSat CRC and Professor Ryszard Kowalczyk, University of South Australia
Advancements in space technology continue to push the boundaries of possibilities on orbit, with spacecraft autonomy seen as the key to nextgeneration space systems. These new systems promise to enable new applications and increase the efficiency, responsiveness and continuity of space-based Earth and science observations, communication and connectivity, or Defence and National Security needs. With the aim of flying spacecraft smarter, with reduced burden on ground segment operators and users and built around a responsible approach in congested orbital regimes.
The potential of spacecraft autonomy for Australia
For Australia, the possibilities are particularly exciting. While the nation’s space sector is still relatively young, it is already producing game-changing technologies, including worldfirsts across many sectors, from defence to communications. Distributed architectures and constellations of small spacecraft are achievable prospects that will enable us to take advantage of these new space systems.
One key factor in realising this vision is spacecraft autonomy and onboard artificial intelligence (AI). Recognised as a key capability, this is an opportunity for Australia to build on its technology prowess, developing these innovative technologies and contributing to the international space ecosystem. This is a huge opportunity for Australia to develop AI-based spacecraft autonomy capabilities for the next-generation space systems to enable national missions and achieve home-grown benefits of remote sensing, communications, and other vital services from space.
Spacecraft autonomy can cover many spacecraft and payload aspects, from planning and executing missions on board to controlling the spacecraft in real-time, even when out of contact with ground stations. Key applications of spacecraft autonomy include:
• Continuous mission planning and real-time execution.
One of the primary benefits of spacecraft autonomy is the ability to perform continuous mission planning and execution onboard without relying on constant communication with ground stations. This allows the spacecraft to adapt to changing conditions and respond to unexpected events in real-time without needing to wait for commands from Earth. This can include an ability to navigate autonomously and adjust its trajectory based on scientific observations, avoid hazards or conjunctions or meet mission requirements.
Maximising mission objectives
Onboard decision-making enables the spacecraft or payload to maximise mission objectives by utilising the available onboard resources or even capabilities of nearby constellation spacecraft or edge processing. For example, if a spacecraft has limited power or fuel, it can prioritise its activities to ensure the most critical objectives are achieved before exhausting its resources. Additionally, spacecraft can work together in a coordinated manner to achieve shared goals, such as performing scientific observations or communicating with Earth.
• System robustness
Spacecraft autonomy also enhances the robustness of the spacecraft systems, which refers to its ability to continue functioning in the presence of onboard failures and external uncertainties. By implementing faulttolerance and self-healing mechanisms, spacecraft can detect and recover from failures autonomously, reducing the risk of mission failure or loss.
SmartSat is supporting the move towards resilience through a "smaller, smarter, many" spacecraft approach, focusing on technologies to enable these future distributed space systems and small satellite constellations. This hybrid space architecture is a shared vision with Defence allies, while also applying to future commercial and science-based constellation missions. Autonomy is a critical enabler for resilience and aids future Defence services from space remain resilient and secure during threats, faults, and unexpected situations.
There are five target outcomes defined that SmartSat researchers are focusing on developing:
Smart Sensing: Obtaining "live" information and reducedlatency actionable intelligence, including direct user tasking through onboard processing for real-time inferences
Smart resiliency: Protecting and operating assets in a contested and congested environment, with local awareness, fault and threat protection and responses for resilience
Smart spacecraft: Squeezing more capability out of small spacecraft and their payloads through management and optimisation of onboard and distributed resources
Smart constellations: Federated constellations and operations with minimal human interaction, providing services to the user (as opposed to operating a spacecraft for a service)
Smart assurance: Building autonomy around trust and ethical choices, so actions are predictable and confidence is quantified to the user/operator
Achieving true autonomy in space flight
The adoption of truly autonomous spacecraft has been limited due to the inherent risk-averse nature of operating a complex and expensive spacecraft in the remote and unforgiving environment of space. As a result, large operations teams and onboard state machines are still the norm. However, the space industry is adopting edge processing and payload smarts and overcoming these challenges to fully realise the potential of spacecraft autonomy.
One of the areas that needs to be addressed for true autonomy is small satellites' size and weight constraints. These also offer an ideal platform for training, running and maintaining large deep learning (DL) models on low-GPUs or single-board computers. Small satellites also offer the potential to optimise the design of DL models to minimise power usage and memory footprints, without impacting overall performance.
Another area to explore is how deployed AI/Machine Learning (ML) algorithms should operate in rare/edge scenarios when extra support from the ground station is needed. For example, considering how to recalibrate deployed DL models in orbit by detecting the data drift issue and re-developing models by adjusting the architecture and parameters. It is also vital to examine the inherent limitations or minimum DL building blocks needed to extract actionable intelligence onboard for specific enduser applications.
Achieving autonomy in space demands properly addressing all these fundamental and practical issues related to onboard processing and trusted AI.
Recent progress in the field
Spacecraft autonomy has been actively researched for some decades. The implementation depends on specific mission requirements and constraints, varying between a very low level of autonomy with a high level of ground control, to a high level of autonomy where most functions are performed onboard.
Most current AI-based solutions are typically deployed with the main processing performed on the ground, while onboard processing is often limited to data collection, filtering, and aggregation. Recently, there has been an increased focus on generating more actionable intelligence from academia and industry. Several companies are now providing commercial software for AI-based onboard processing, including the AIKO’s MiRAGE solution for autonomy (onboard data processing for perception) and downlink optimisation through onboard image processing.
Another area of progress is fault detection, isolation, and recovery (FDIR). Functionality has been gradually transferred from the ground to the spacecraft to ensure safe and high-performance operation with less intervention by human operators. This is particularly important for deep space missions or missions involving hundreds of small spacecrafts. However, FDIR uses pre-defined recovery strategies and cannot anticipate potential threats, unexpected errors or malicious attacks.
To this end, there is a need for Australia’s space sector to develop novel concepts, methods, and technologies to provide new AI-based spacecraft autonomy capabilities for next-generation space systems. Potential areas of interest could include onboard processing and actionable intelligence, small spacecraft and constellation resilience, dynamic optimisation of constellation resources and realtime tasking and resource allocation.
Australia is building skills, hardware, and capability in its burgeoning space industry and this must include autonomous algorithms in order to remain at the innovative front of space system development and implementation. As autonomous technologies advance and are embraced, their place in space will expand and present new opportunities for emerging Australian space technologies. The more we push the boundaries of what is possible, the more exciting the future of space exploration begins to look. With nextgeneration space systems on the horizon, the possibilities for science, discovery and innovation are endless.