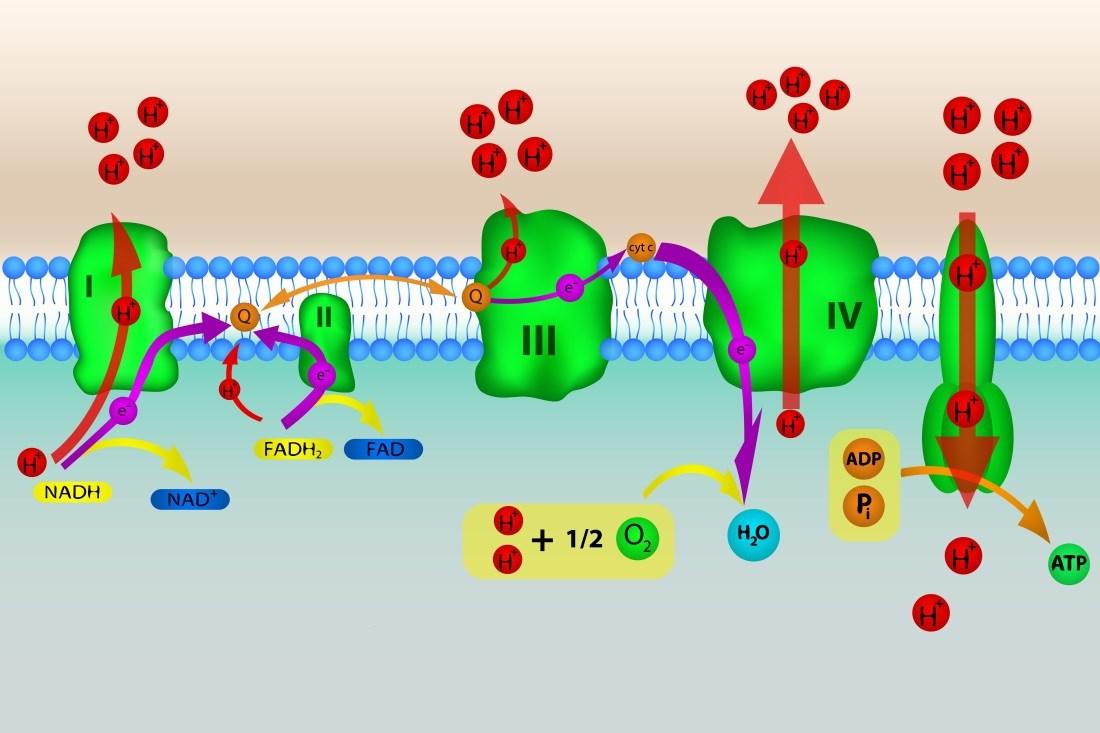
4 minute read
Oxidative Phosphorylation
by AudioLearn
There are eight steps to the citric acid cycle, which is a closed loop. This breaks down glucose further into CO2 and generates some important energy-producing molecules. It starts with acetyl CoA and oxaloacetate and forms citric acid or citrate (and thus the name). This is a six-carbon molecule. Through a series of reactions, 2 molecules of NAD become three NADH molecules and one FAD becomes an FADH2 molecule. There is a series of reactions inside the mitochondrial matrix that take the oxaloacetate and acetyl-CoA, removes two carbon dioxide molecules to turn the entire thing back into oxaloacetate, which is recycled for the next loop. Another energy molecule (GTP or ATP, depending on the organism) gets made in this cycle.
Because there are two acetyl CoA molecules per glucose molecule made, the net end is that there are six NADH molecules, 2 FADH2 molecules, and 2 ATP molecules (or GTP molecules) made. Because GTP can be used to make ADP without difficulty, most sources consider that ATP is essentially made in this cycle, at least in higher animals.
Advertisement
OXIDATIVE PHOSPHORYLATION
This is a highly important part of cellular respiration, taking place in the mitochondrial cristae. Remember that there are all of these high-energy molecules that aren’t ATP, which can be used to make ATP in the process. It involves a “proton gradient” or a positively-charged gradient (also referred to as a chemiosmotic potential) across the inner membrane of the mitochondria. ATP synthase is heavily involved in this process as many ATP molecules are made using this gradient to drive the biochemical process.
So far, there has been a lot of “reduction” or reductive chemical reactions going on. This is a time for oxidation to take place. This is why it’s called oxidative phosphorylation. It takes oxidative processes to “phosphorylate” the ADP molecule to make ATP. This is where oxygen is necessary because something has to be the final electron acceptor in this chain of electrons being transferred from one molecule to the next. Oxygen is a perfect electron acceptor because it can bind with hydrogen atoms to make water. Figure 22 describes what oxidative phosphorylation looks like:
This process occurs in the inner mitochondrial membrane—in the cristae. There is a pH gradient across the membrane with electrons being passed back and forth across the membrane until the final donation process takes place. ATP synthase is a rotary mechanical motor enzyme that needs to be rotated in order to do its job. The flow of hydrogen atoms across the pH gradient drives the rotation process so that ATP can be made from ADP. This ATP synthase is the whole purpose of this electron transport chain in that the chain is used to drive this enzyme.
It should be noted that, in prokaryotes, such as bacteria and archaea, this process can happen but there are different electron receptors, different enzymes, and no mitochondria involved. The process instead happens across the cell membrane itself and uses different chemical substrates. Bacteria and other prokaryotes must grow under a variety of environmental conditions with electron donors being things like lactate, hydrogen, or formate, and electron acceptors being DMSO, oxygen, or nitrate.
Because these are oxidative reactions, they can lead to the formation of reactive oxygen species, such as hydrogen peroxide and superoxide, which ultimately lead to free radical formation and cell damage. This is a natural part of the metabolic process and is why the body has antioxidants to take care of these normal metabolic end-products.
In the case of eukaryotes, the electron donors in the process are the high energy products that were made in glycolysis and in the Krebs cycle—namely, the NADH and FADH2 molecules that seemingly didn’t make sense before. Just to summarize this entire process, these are the ATP production quotas from a single molecule of glucose:
• Glycolysis—this uses up 2 ATP molecules but gives off 4 later for a net of 2 ATP molecules made. It also makes 2 NADH molecules that go to the oxidative phosphorylation process to make 1.5 net molecules of ATP (because it takes ATP energy to transport it across the mitochondrial membrane.
• Oxidative decarboxylation—this is the intermediary step between glycolysis and the Krebs cycle. It makes 2 NADH molecules that go on to make 5 ATP molecules in oxidative phosphorylation.
• Krebs cycle—this is the largest source of ATP molecules. Two are made as part of the Krebs cycle itself, while 15 are made from the 6 NADH molecules created by the cycle and used in oxidative phosphorylation. Three ATP molecules come from 2 FADH2 molecules created by the cycle and used in oxidative phosphorylation.
The total yield is 30 to 32 ATP molecules, most, as you can see by the tally, coming from high energy molecules in oxidative phosphorylation. Theoretically 38 ATP molecules are made; however, it takes energy to move ADP, phosphate, and pyruvate across the membranes, resulting in losses that offset the otherwise high yield. The result is the complete oxidation (or breakdown) of glucose into CO2 and water. The breakdown for ATP production is 2.5 ATP molecules per molecule of NADH and 1.5 ATP molecules per molecule of FADH2.
Interestingly, cellular respiration can, in some situations and in some cells, purposely be inefficient for the express purposes of generating heat. Newborn humans have brown fat, which contains an uncoupling protein called thermogenin that purposely short circuits the electron transport chain, generating heat energy instead of ATP. This protein is also expressed in hibernating animals that allows them to stay warm during the cold weather.