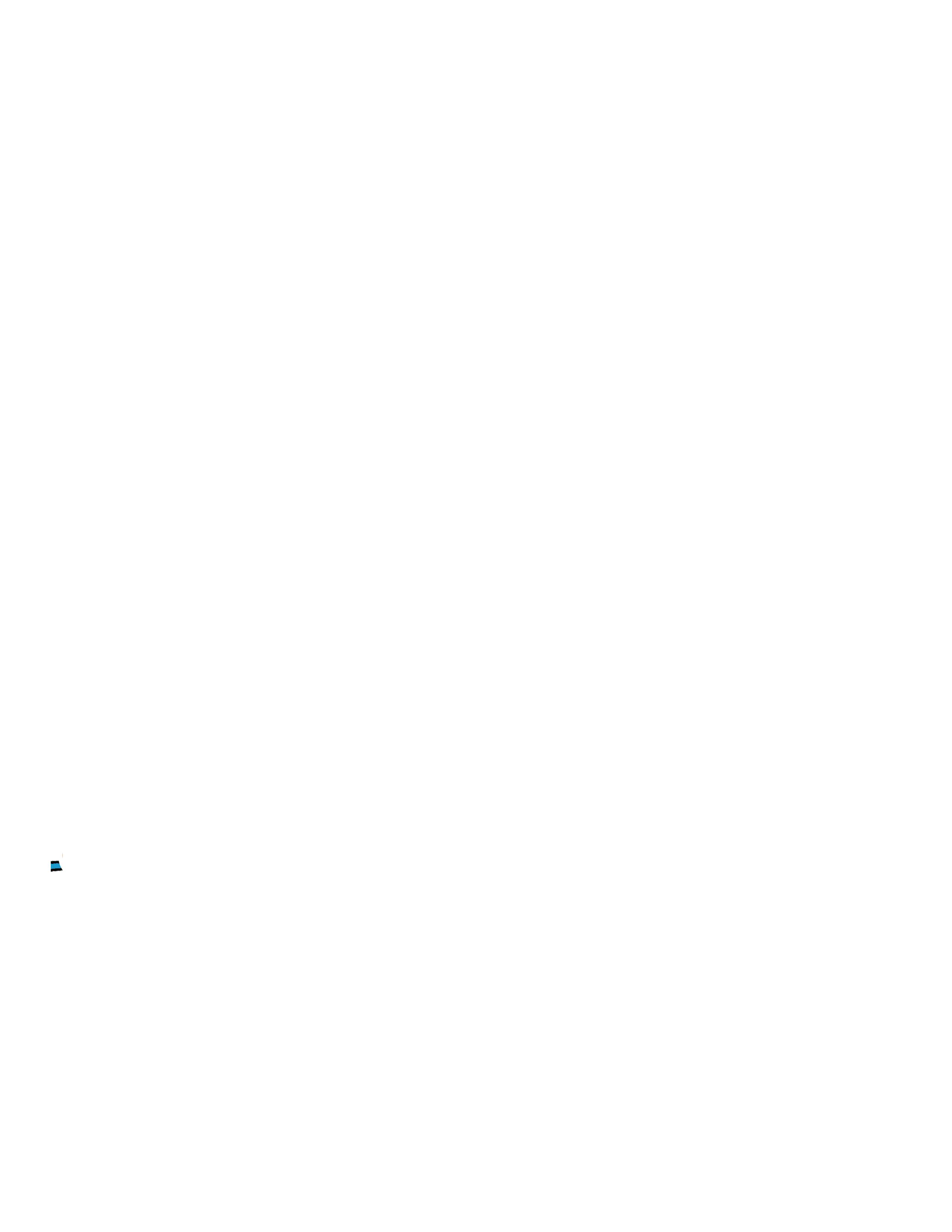
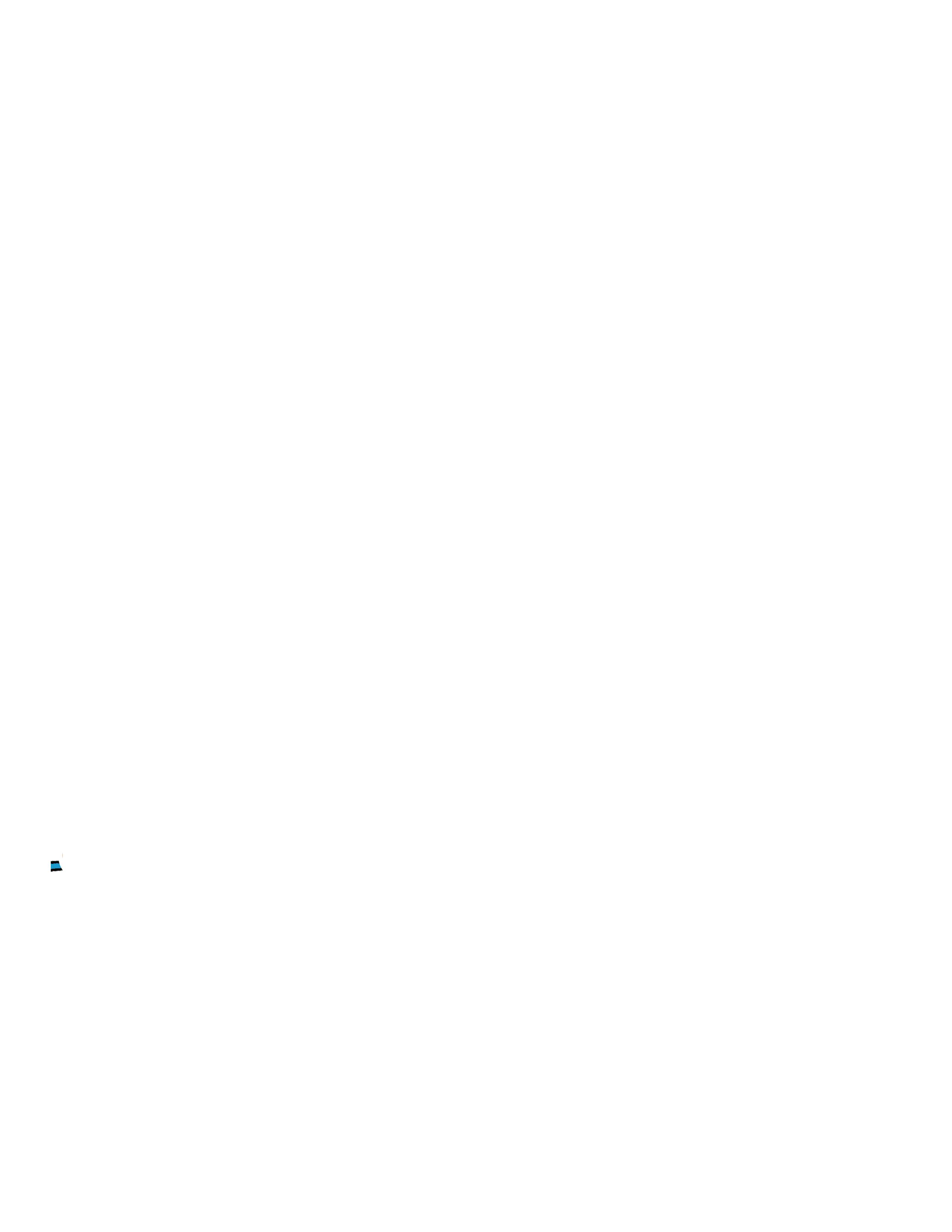
Genetics
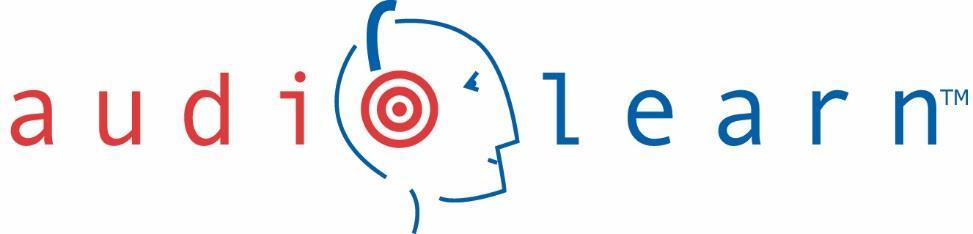
Quiz Answers ..........................................................................................303
Chapter One Answers ..................................................................................................303
Chapter Two Answers ..................................................................................................305
Chapter Three Answers................................................................................................306
Chapter Four Answers .................................................................................................307
Chapter Five Answers ..................................................................................................309
Chapter Six Answers .................................................................................................... 310
Chapter Seven Answers ................................................................................................311
Chapter Eight Answers ................................................................................................ 313
Chapter Nine Answers ................................................................................................. 314
Chapter Ten Answers................................................................................................... 316
Chapter Eleven Answers .............................................................................................. 318
Chapter Twelve Answers..............................................................................................320
Chapter Thirteen Answers ...........................................................................................322
Chapter Fourteen Answers ..........................................................................................324
Course Quiz Answers ...................................................................................................326
PREFACE
The purpose of this audio-course is to help you learn about the field of genetics at the college level of understanding. Genetics is the study of how traits are passed on from parent to offspring as well as how things like natural selection affect the genetics of a given population or species. The field of genetics has come a long way since the first research was done on how genetic traits are passed on from generation to generation.
We will also talk a great deal about what is known about genetics at the molecular level. After more than a century of research in this field, scientists who study genetics now know about what genetic material looks like and the molecular processes behind things like cell replication, sexual and asexual reproduction, and genetic mutations in all types of organisms. The knowledge of these things have greatly impacted our understanding of genetic diseases and has paved the way toward being able to manipulate the genetics of a species in everything from cloning to genetic engineering and the use of genetics to treat all types of diseases.
At the completion of this course, you should feel more confident about the large-scale and molecular aspects of genetics as they apply to prokaryotic species and to eukaryotic organisms, such as plants and animals, including humans and our many genetic disease states.
In chapter one in the course, we will talk about some of the basic issues in genetics and genetic research. The history of genetics and how it has been understood in the past is covered in this chapter as well as what we know about genes and chromosomes in prokaryotic organisms and in eukaryotes. Genetics is a broad field of study, with several branches we will touch upon. We will expand on this to talk about molecular gene expression, the genetic code, and how genes become traits, which will be further explained in later chapters.
Chapter two covers the seminal work of Gregor Mendel, partly because no discussion of genetics should not include his work. Mendel worked with pea plants in a systematic way to identify how traits get passed from parent plants to offspring. His work was
important to the modern study of genetics. The chapter also looks into the work of those who discovered the structure of DNA, which opened the door to genetic study even further by identifying what DNA looked like and why this was important to how it works.
The focus of chapter three in the course is molecular genetics, which is one of the major sub-categories of genetics. This deals mainly with DNA, RNA, and how these interact in order to make proteins. What happens to proteins after they are made is called post translational modification of proteins, which is how a protein structure gets finalized. How these processes happen is different in prokaryotes and eukaryotes, which will be covered in this chapter.
Chapter four is about gene regulation in prokaryotes and eukaryotes. More accurately, though, the chapter talks about trait regulation because it also focuses on how the entire process from gene regulation to epigenetics occurs. Much is known about how genes in prokaryotes are regulated directly; less is known about gene regulation happens in eukaryotes. As it turns out, there are other regulatory processes, such as translation regulation and epigenetic modification, which involve modifying traits in ways other than through genetic means.
The main focus of chapter five in the course is how cells divide and how organisms reproduce to create the next generation. With bacteria and other prokaryotes, the process is simpler and involves asexual reproduction, which mainly means they undergo binary fission. Mitosis is a method of cell division that is distinct from binary fission and occurs in eukaryotes. Sexual reproduction occurs in plants and animals. It involves a process called meiosis, which is discussed in this chapter. Finally, the chapter ends with the complex topic of sex determination and how this happens from a genetic perspective.
Chapter six is concerned with the different patterns of inheritance seen in genetics. There are dominant and recessive inheritance patterns as well as x-linked inheritance and codominant inheritance. The topics of pleiotropy, lethal alleles, and epistasis or gene to gene interactions are covered in this chapter as well. Many disorders have multigenic or polygenic inheritance patterns, which involve many different genes. Some
of the major genetic diseases in humans and their inheritance patterns are discussed at the end of this chapter.
Chapter seven in the course covers extranuclear genetics in eukaryotes, which mainly involves the genetics involved in the mitochondria and chloroplasts of these cells. The first topic of discussion is the theory of endosymbiosis, which is how it is believed that these structures first entered other cells to create organelles inside eukaryotes instead of being separate entities. The different genetic issues of chloroplast and mitochondrial DNA are covered in this chapter, including the epigenetics we know about so far that influence mitochondrial gene expression.
The focus of chapter eight is the different variations possible in chromosome structure and number, which is largely a phenomenon of eukaryotes. There are many different chromosome variations to consider in both plants and animals. In this chapter, we discuss polyploidy, which is having more than one set of chromosomes, aneuploidy, which involves any variation in chromosome number beyond what is normal for the species, and things like insertions, deletions, inversions, and translocations all of which affect part of a chromosome and affect how the chromosome behaves in the cell or organism.
Chapter nine in the course is about the different mechanisms of gene transfer, also called lateral gene transfer or horizontal gene transfer. There are different ways that genes can be transferred from the environment or between bacteria, which is a form of sexual reproduction among prokaryotes, even though it is not sexual in the traditional way. Lateral gene transfer has implications in medicine, largely because it is through these mechanisms that things like antibiotic resistance occurs. These issues are discussed as part of this chapter.
Chapter ten provides a discussion of viruses from a genetic perspective. You’ll learn the different types of viruses in terms of structure and the different aspects of their genetic makeup. The genetics of viruses, including how and why they mutate over time is covered as well as how they invade a host and ultimately replicate. Viruses have implications in industry, technology, and medicine, which are talked about in this
chapter. Finally, some viruses contain genes that promote cancer in their host. How they do this is part of this chapter.
The focus of chapter eleven in the course is gene mutation and how DNA repairs itself on a regular basis in order to fix mutations that could otherwise damage or kill the cell. In this chapter, we talk about the different types of mutations, the various mutagens and how they cause DNA abnormalities, and how these mutations are ultimately fixed in the vast majority of cases. While DNA mutations lead to a wide variety of human genetic diseases, the ways in which mutagens lead to cancer is discussed as part of this chapter.
Chapter twelve looks at some ways that genes, DNA, and their products are studied and used in certain technologies. This is a new area of research that is continually evolving and looks at ways to clone genes, sequence genes and chromosomes, and separate DNA, RNA, or proteins using blotting techniques. As you’ll see, these technologies have been employed in medicine using things like the polymerase chain reaction. Also covered in this chapter is genomics, including structural and functional genomics and how these are used in DNA technologies.
The focus of chapter thirteen in the course is biotechnology, which is how modern science has taken earlier genetic research and applied it to technology so that all aspects of modern life are potentially infected. Issues like how microorganisms can be used in biotechnology and the creation of genetically modified animals and plants have been areas where this technology has most been applied to everyday life in modern societies. Gene therapy is just now becoming a reality and, while there is much more to do in this area, this will also be discussed in this chapter.
Chapter fourteen involves the common issues seen in understanding population genetics, including how population genetics interacts with principles of evolution to affect the genetic diversity of the world’s living things. In the chapter, we discuss genetic screening in human populations and why it is done. Other important aspects of population genetics we talk about in the chapter include genetic drift, migration, nonrandom mating, natural selection, and the Hardy Weinberg principle, which are all ways of understanding why we have genetic diversity in the different populations on earth.
CHAPTER ONE:
B
ASICS OF GENETICS
In this chapter, we will talk about some of the basic issues in genetics and genetic research. The history of genetics and how it has been understood in the past is covered in this chapter as well as what we know about genes and chromosomes in prokaryotic organisms and in eukaryotes. Genetics is a broad field of study, with several branches we will touch upon. We will expand on this to talk about molecular gene expression, the genetic code, and how genes become traits, which will be further explained in later chapters.
HISTORY OF GENETIC RESEARCH
To some extent, the study of genetics has been ongoing since the time of Pythagoras, Aristotle, and Hippocrates, when nothing was known about genes or chromosomes, which now know form the basis of what makes an organism of a certain species or have a certain trait. Modern genetics was first explored by Gregor Mendel, whose seminal work led researcher to understand the transmission of traits from parent organisms to offspring which was work he performed without an understanding of the molecular basis for gene transmission.
While Mendel’s work was very important to the understanding of genetics, it was first published in 1866 but was not discovered by scientists at large until 1900. Shortly after that, other groups of scientists and researchers uncovered how traits could be passed from parent to offspring in non plant species, such as the fruit fly. Still others applied what was known about genetics to populations of organisms, beginning the field we now call population genetics. How genetics influenced evolution was also uncovered.
Once the structure of the basis of the genetic code in nearly every organism type on earth was defined by researches in 1953, the field molecular genetics was born and scientists came to understand how DNA or deoxyribonucleic acid within each cell of most organisms becomes translated into proteins made by the cells, which further the phenotype of an organism. The genome of an organism is the collection of genes each
has, which lead to its genotype, while the phenotype usually relates to the organism’s physical appearance or biochemical functions. The field of study led to being able to identify the genome in a great many organisms, including humans.
Once the genome of humans was identified, doctors could then better understand the basis of genetic diseases and began understanding how to manipulate genes for the benefit of the host organism. In addition, treatments based on the genetics of an organism could be utilized to treat disease states in humans and other organism.
In what we call the pre Mendelian era, ancient thinkers like Aristotle and Hippocrates, formed opinions on how heredity worked. Hippocrates developed ideas related to the concept of pangenesis, which was that the entirety of the organism participated in passing on traits to offspring. Aristotle proposed the idea that male semen somehow interacted with female menstrual blood to create unique offspring.
Epicurus from Ancient Greece himself contributed to the understanding of genetics at the time by his observations of families and the proposal that both males and females contributed to the phenotype of offspring through sperm atoms in each gender. He also understood on some level what constituted a dominant trait and what was a recessive trait. Long before Mendel, he described the independent assortment and segregation of these sperm atoms.
Indian thinkers around 300 BCE felt that there were four determinants related to the appearance of a child. These were those gotten from the woman’s reproductive materials, things gotten from male sperm, the specific diet of the pregnant mother, and ill defined aspects of the soul of the fetus.
Writer Al Jahiz in the Middle East proposed that environment played a large role in the survival of an animal. As early as 1000 CE, at least one Arab physician described the hereditary basis behind the phenomenon of hemophilia, while HaLevi from this part of the world was able to define the difference between dominant and recessive traits as they applied to genetic inheritance patterns.
The preformation theory of genetics came from the works of Anaxagoras in Ancient Greece. This theory was maintained is some form until the mid nineteenth century. It was strongly related to evolutionary theory of the era. Anaxagoras believed that the
entirety of an organism was already preformed in either the egg or sperm and only needed to grow. This theory opposed that of epigenesis, which was that organs and structures in any organism came to be only when the organism developed. Of these two theories, epigenesis predated preformation. It was only later that epigenetic theories were felt to be more valid then those of preformist thinkers. The application of what was known about genetics was first applied to plants, particularly as scientists came to understand the vast diversity of plants. Researchers following Linnaeus and others did numerous experiments on plants using hybridization, particularly between species in order to make hybrid plants. This led to an increase in the numbers of stabile varieties of plants common today. The concept of dominance was introduced as was the idea of novel mutations. Those that bred these plants did not share with the rest of the world what they had learned from these processes with other researchers, however.
We will talk more about Gregor Mendel’s work in the field of genetics in the next chapter. Mendel worked from 1856 to 1865 in the area of crossbreeding pea plants and discovered that there were specific rules as to how different traits got passed on, essentially proving the concept of the existence of dominant and recessive traits. He discovered what we now call Mendelian inheritance which, as you’ll find out, does not represent all of how inheritance works. His work contradicted prevailing thought that traits represented blending inheritance, in which offspring carried blended traits they got from the parent organisms. He used the term “factors” because genes had not yet been discovered.
Charles Darwin himself contributed his own ideas on genetics through the theory of pangenesis. In this theory, it was believed that all parts of the body emit small particles called gemmules that migrate to the reproductive organs in order to contribute to the fertilized egg so that traits could be passed on. This was added onto genetic theory by proponents of Lamarckism, which involved the belief that changes in the body over a lifetime also contributed to what was passed onto offspring.
August Weismann did experiments in the 1880s on mice, which were bred after their tails were surgically removed. None of the offspring would later share this deformity.
This essentially refuted both Lamarckism and pangenesis and led to the idea that there was germ plasm in the egg and sperm, which were the only two cells that carried genetic information to the offspring.
Hugo DeVries in the 1890s locked on to the germ plasm theory of genetics and wondered if what was passed onto the offspring were discrete things or simply followed blended inheritance patterns. His published paper in 1897 indicated the researcher had decided that discrete packets of information were passed to offspring rather than anything that was blended. He is credited with rediscovering Mendel’s work in 1900, although he essentially copied Mendel’s theory without initially crediting him.
After Mendel’s work was rediscovered, researchers began to study the same patterns of inheritance in fruit flies, which are members of the Drosophila genus. Thomas Hunt Morgan was one of these researchers who himself uncovered sex linked inheritance. Morgan also brought forth the idea that chromosomes in the sex cells existed and carried genes that were later passed onto offspring.
Out of Morgan’s work came the work of Alfred Sturtevant, who first mapped the genes of the chromosome of Drosophila melanogaster in the 1920s. By 1928, Fredrick Griffith demonstrated that genes could be transferred from one species to another by injecting mice with lethal bacteria that had been treated with heat to kill certain transferred genetic information. These were called Griffith’s experiments. This genetic information was first found to be carried as deoxyribonucleic acid or DNA in 1941. Prior to this, it was believed that proteins carried genetic material. The ideas of gene mutation were promoted by George Beadle and Edward Tatum, who specifically studied mutations that led to aberrations in cellular metabolic pathways. This also led to the concept of one gene leading to one enzyme.
In 1952, Rosalind Franklin and Raymond Gosling first demonstrated the helical shape of DNA, which was furthered by the work of James Watson and Francis Crick in 1952 so that DNA was finally identified as the double helix it is now known to be today. Soon afterward, the central dogma of molecular genetics was determined to be true, which was based on the idea that DNA is transcribed to make RNA that translated the information to proteins.
The study of genetics expanded greatly and quickly from that moment on. The first gene was sequenced in 1972 and both Richard Roberts and Phillip Sharp showed that genes could themselves be split in 1977. This furthered the concept that one gene could make several types of proteins. Genomes began to be sequenced and it was understood that certain genes could overlap with other genes.
The genomics era of genetics started in 1972 when the first gene was sequenced and continues today. Gene mapping was identified in 1980, which was also when the first US patent was awarded for gene cloning. The first genetically engineered insulin was approved in 1982 and the practice of polymerase chain reaction was invented in 1983, which is now used for DNA amplification in the diagnosis of infectious diseases.
In 1985, DNA fingerprinting techniques were created using chemical probes to examine DNA between different humans. By 1989, the CFTR gene that led to cystic fibrosis was first sequenced. Soon afterward, it became possible to use amniocentesis to identify hemophilia, cystic fibrosis, and other diseases in utero. The first breast cancer gene, called BRCA1 was discovered in 1994, followed closely by the identification of the second breast cancer gene, called BRCA2. The first cloned sheep was born in 1997. The Human Genome Project to sequence human DNA was completed in 2003.
CATEGORIES OF GENETIC STUDY
We have touched briefly already on the three major branches of genetics, which are classical genetics, molecular genetics, and population genetics. There are several areas of genetic study that are considered minor branches, such as behavioral genetics, developmental genetics, genetic engineering, genomics, human and medical genetics, microbial genetics, and psychiatric genetics. Let’s look at the three major branches studied today.
In classical genetics, research is based on the visible results of sexual reproduction. It dates back from the time of Gregor Mendel but has been expanded so it is understood now at a molecular level. At its heart is the concept of a gene being the basic unit of genetic transmission and on the idea that organisms are diploid, meaning they have two alleles of which one comes from the mother and one comes from the father.
Genetic linkage was identified through classical genetic study, showing that some genes do not separate independently at the time of meiosis in the creation of a gamete or sex cell, largely because the genes exist too close together on a chromosome. In classical genetic study, things like genes, heredity, and genetic variation are studied. Heredity is the ability of characteristics to be passed from parents to offspring. Genetic variation comes from the idea that related individuals are not identical to one another and the idea that traits are passed on through genes is part of this area of genetics as well. Each sex cell, defined as sperm or an egg cell, has just one allele or copy of a chromosome or chromosomes so these cannot themselves make an organism. When these unite, however, they form a diploid gamete that has two alleles per chromosome. DNA in eukaryotes is defined as being linear so that each line of DNA together is called a chromosome. As mentioned, the genotype is the arrangement of genes that lead to a specific trait, while the phenotype is the physical expression of the genotype or what the organism looks like. Sometimes the term genotype is called its genetic makeup. Some alleles are dominant over others and are preferentially expressed, while others are recessive so they are not seen if the organism also has a dominant allele.
Molecular genetics, on the other hand, is a field of genetics that came after classical genetics. With molecular genetics, there are several interrelated fields of study connected to it, such as cell biology, inheritance, biochemistry, biotechnology, and molecular biology.
This branch of genetics was probably started in 1952, when the structure of DNA was first identified. It was expanded further when researchers used restriction endonucleases to created techniques used in genetic engineering. Out of this came the practices of electrophoresis and Southern blotting, which will be discussed later in this course, in order to identify certain DNA segments using hybridization probes. Recombinant DNA technology, used to make many synthetic drugs, has come out of molecular genetic study. Another commonly used technique in medicine to identify organisms without culturing them is called polymerase chain reaction also a byproduct of molecular genetics. All of this culminated in the identification of the human genome, which created the field of genomics. Genomics takes the DNA molecule through the processes that make a functional protein.
The study of molecular genetics uses many techniques. Forward genetic techniques are specifically used to identify the genes or mutations that produce certain phenotypes in an organism. Genetic screening techniques will create random mutations in a genome to look for different phenotypes. Transformation techniques study antibiotic resistance in bacteria. Mutant genes can be identified as being dominant or recessive, while epistatic genes are mutant genes that mask another gene’s phenotype. DNA sequencing is then used to map where a certain mutation is located.
Reverse genetics is another aspect of molecular genetics that uses an intentional mutation in a gene to create and identify a different phenotype. This helps to identify what the function of the gene is supposed to be. It sometimes induces gene knockout, in which the entire gene is deleted. On the other hand, gene knock in techniques can be used to add genes into the genome. These added genes are called transgenes and they usually result in a gain in function of the host. These reverse genetic techniques are generally faster than forward techniques.
Population genetics is another major field of genetic research, itself being part of the study of evolutionary biology. It studies things like the organism’s adaptation to the environment, the development of new species, and population structure from a genetic perspective. It has been helpful in identifying modern evolutionary theories. Instead of using phenotypes to study different populations, it relies on the genotype of an organism.
Population genetics was first created by combining biostatistics with Mendelian inheritance to identify issues related to the natural selection of some organisms over others. It relies on mathematical principles used to describe how populations are able to maintain some level of variation. According to population genetics, the variation in a population of organism is maintained by the action of many genes acting together. Out of this came the idea that the frequency of certain alleles in a population could change, leading to evolution. This was furthered by areas of study in animal breeding that resulted in inbreeding and what’s called genetic drift. Population genetics was developed after classical genetics but before molecular genetics.
Through the study of population genetics, the ideas leading to modern synthesis concepts in evolution were created. Before population genetics, ideas like orthogenesis and Lamarckism were used to explain why there was such a great deal of genetic diversity in the world. These theories have been refuted through the study of population genetics. Now, mathematical models are used to explain things like the genetic diversity seen in small isolated populations, such as that which exists on the Galapagos Islands.
Another related theory coming out of the study of population genetics is called neutral theory. The idea behind this is that most mutations that can happen in a population are considered deleterious for those organisms who have them so they do not persist in the population. The rest are considered to be neutral, with only a few being advantageous to the organism. Neutral mutations may or may not get passed on but this is largely through chance of through the processes of genetic drift.
Other things that are studied in population genetics are things we will talk further about as you listen to this course. These include things like natural selection and sexual selection, which determine whether or not a specific trait survives in a population, mutation, which is how genetic variation in a population occurs in the first place, genetic drift, which is the change in the frequency of an allele caused by random events rather than environmental pressures, and gene flow, which involves the actual transfer of alleles between populations caused by immigration of some of the population’s individuals.
CHROMOSOMES AND GENES IN PROKARYOTES AND EUKARYOTES
We will talk more about the genetics of prokaryotes and eukaryotes later on but, in this section, we will talk about the chromosomes seen in these types of organisms. Prokaryotes are organisms that do not have membrane bound organelles inside each cell. This means they do not have separate, bound nuclei in the cell to house genetic material. Bacteria and Archaea organisms are both types of prokaryotes. Eukaryotes have membrane bound organelles in the cell so they do have nuclei where genetic material is stored. Fungi, plants, and animals of all types are eukaryotic organisms.
The genome of prokaryotes is much simpler than in eukaryotes. Remember that the genome of an organism is the sum total of genetic material in each cell. Prokaryotes usually have a single chromosome plus one or more small, circular plasmids, which contain just one or a few genes. Figure 1 shows you what a prokaryotic chromosome would look like:
Figure 1.
Chromosome studies certainly could be done on any prokaryotic organism but most have been performed through studying the chromosome of the E. coli bacterium. The chromosome is made from double stranded DNA that clustered together in an area called the nucleoid, which is not bound by a membrane.
Despite their small size, prokaryotes have rather large genomes. The chromosome is able to fit into such a small space by being rolled into coils in a process called supercoiling, which twists the fibers so they form a tight ball. Positive coiling happens
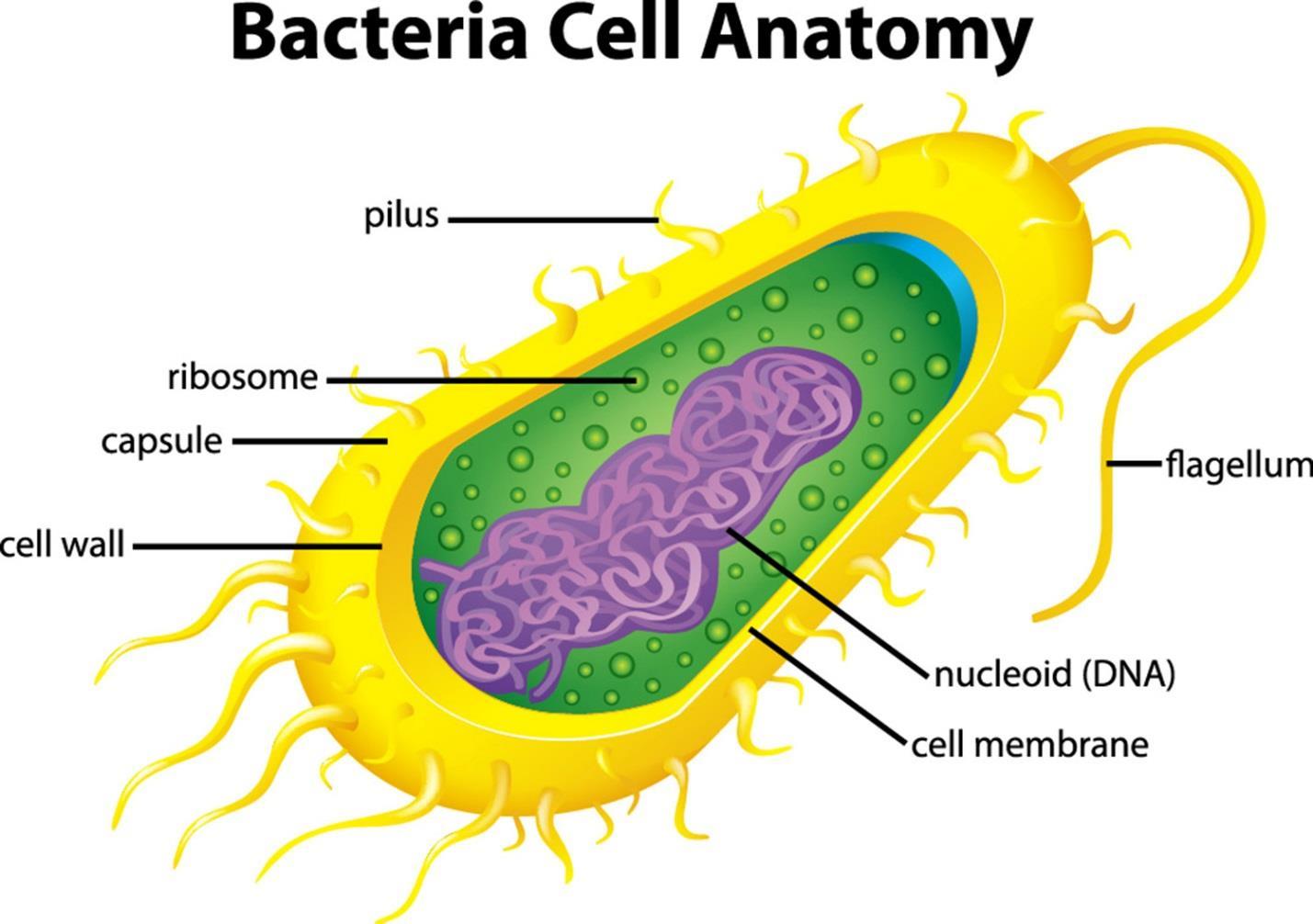
in the same direction as the double helix, while negative coiling is in the opposite direction of the coiling. Most of the time, bacterial DNA is negatively coiled. Prokaryotes reproduce through asexual means most of the time and the DNA is considered haploid, which means there is only one copy of any gene per cell. Plasmids, as mentioned, are also circular but can be linear. Most plasmid DNA isn’t necessary for the bacterial organism to survive but give it an added advantage, such as antibiotic resistance. Plasmids replicate themselves separately from the replication of the chromosome.
Chromosomes in prokaryotes need to be so efficient in order to be able to fit in the cell. Because of this, there isn’t very much of the bacterial chromosome that isn’t directly involved in coding for proteins. While eukaryotes have 98 percent of their DNA that doesn’t get coded into known proteins, prokaryotes have much more coding DNA with only 12 percent of the DNA not coding for proteins.
Eukaryotic chromosomes are much more complex that is true for prokaryotes. There is a great deal more DNA involved overall but the majority of it does not seem to code for any proteins yet discovered. Chromosomes in eukaryotes are linear and paired so that there are generally two copies of each gene, except for those stored on the sex chromosomes. The number of chromosomes in each species varies with the species and, interestingly, it is not true that more complex organisms have more chromosomes. Plant, in fact, often have many more chromosomes than is true for complex animals. Eukaryotes have the same problem as prokaryotes in that the DNA must fit in a small space. This is accomplished by wrapping pieces of the DNA around histone proteins. The combination of DNA plus its histone proteins is called chromatin. Figure 2 shows what chromatin looks like. Each bead on the string represents an area that is wrapped around a histone in a structure called a nucleosome.
Chromosomes in eukaryotes look differently depending on what part of the cell cycle you look at. During mitosis or the cell division stage, for example, the chromosomes are much more visible under the microscope. In between those times, however, the chromatin is thin and the chromosomes cannot be individually seen.
When the chromatin is visualized in eukaryotes, you can see three main aspects to it. There is a central centromere near the middle that is particularly important in cell division. It is this part that must separate during cell division. There are two types of arms. The short arm is called the p arm and the long arm is called the q arm. Figure 2 shows what the different types of chromosomes might look like, although you do not have to know the different types:
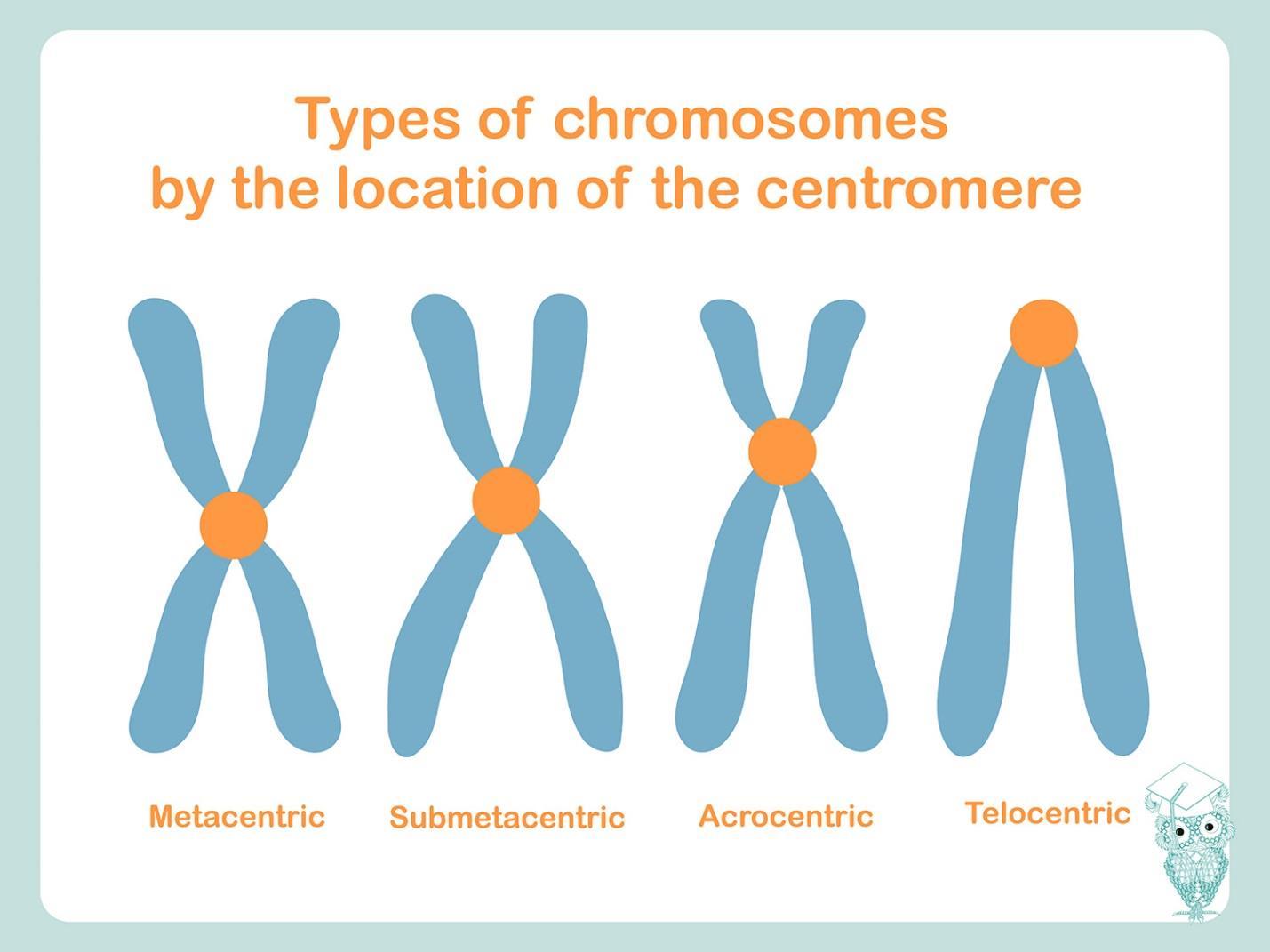
Figure 2.
The ends of each arm is a section called the telomere. Telomeres do not code for any specific gene but serve to protect the end of the DNA arm of the chromosome. Chromosomes do not have any color themselves but can be dyed with Giemsa dye. When you do this, you will see striped chromosomes. The stripes do not necessarily mean anything except for the fact that certain base pairs that make up the long DNA
chain will take up more dye than others. Scientists do use these stripes to look for areas where the chromosome is missing a piece or has an extra piece associated with it. In other situations, fluorescent dyes are used to light up the chromosome using certain microscopes that detect fluorescence. Figure 3 shows the human karyotype using Giemsa dye staining:
Figure 3.
The karyotype of a cell is a picture of what each of their paired chromosomes look like. A picture can be taken and the chromosomes sorted out and ordered, resulting in what you see in figure 4.
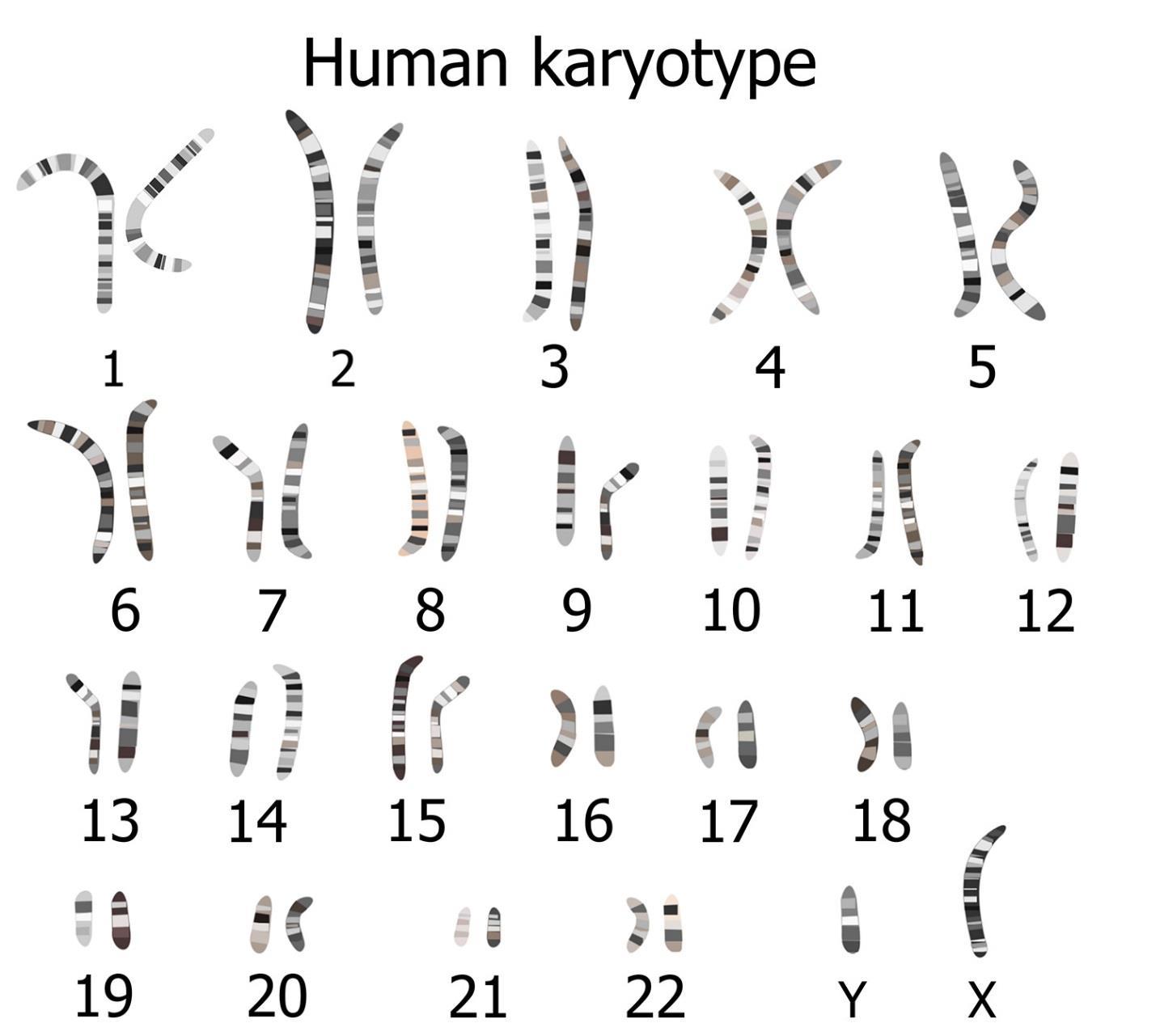
M
OLECULAR
EXPRESSION OF GENES
There is a great deal about DNA, genes, and gene products you will learn about in this audio course. In this section, however, we will provide a brief overview of genes and their expression. DNA and, in particular, the genes in our DNA determine the traits each person has. How this happens used to be a mystery but now, scientists know much more about how a particular piece of DNA becomes a specific trait. Each string of DNA in the cell has a number of genes on it along with varying lengths or stretches of DNA that do not code for anything. Almost all genes code for proteins rather than for fat or carbohydrates. It is through structural proteins that some of the cell is made and through a vast array of functional or “working” proteins called enzymes that other aspects of the cell are made and organized.
DNA, as you will find out later in this course, is made from four different types of nucleotides, which are unique molecules that stretch out in different order along the chromosome. The nucleotides can be read by molecules called RNA or ribonucleic acid. These RNA molecules exit the nucleus in eukaryotic cells and go to another part of the cell nearby called a ribosome. In the ribosome, proteins are made according to the message delivered to the ribosome by the RNA molecule. These proteins are processed further to make final protein structures. Some of these make enzyme proteins that help run every aspect of cell machinery.
In any organism that is multicellular, such as is the case in humans, each cell does not have a different set of chromosomes or different DNA inside them. All of the genetic material available to the whole organism is available in each cell but, of course, as each cell is unique and does different things in the body, mechanisms must be in place to make sure that the cells are unique. How organisms do this is that, depending on the cell type, some genes get turned on to make proteins, while others are turned off. This is how every cell is able to be unique in the proteins made by the cell.
There are a few products of genes or DNA that do not make for proteins. These will make one of two types of RNA molecules, transfer RNA and ribosomal RNA. These are type of RNA that play a major role in the process of making proteins in the ribosomes.
Genes basically provide instructions, usually for polypeptides, which are stretches of protein molecules. There are several processes that go into this, which will be further discussed later in this course. First a gene must be exposed and so it is available to be read. As you have learned, not all genes get turned on in every cell.
When a gene is exposed to be read, it is read through the process of transcription that happens inside the nucleus of a eukaryotic cell. When transcription happens, the double helical shape, which means that the DNA molecule has two strands, separates and a messenger RNA molecule is made that is an opposite match to the DNA strand. As each strand of DNA is different, so is the messenger RNA or mRNA molecule made from it.
In eukaryotes, the mRNA made is not complete as soon as it is made but must be further processed before it can leave the nucleus.
One the mRNA is processed, it leaves the nucleus to where there are nearby ribosomes. These ribosomes are the site for translation. Translation us the process that takes the mRNA message and turns it into a polypeptide or protein chain. It makes use of a language the translates the nucleotide message within the RNA molecule to a chain of amino acids in a polypeptide chain.
The polypeptide is not usually complete once it is translated. It often undergoes post transcriptional modification, which adds or subtracts different molecules to the polypeptide chain in order to allow the polypeptide to make its final shape. When it does this, the final product is often referred to as a protein. This process is called the central dogma in the study of molecular biology. We now know that, during this process, the genetic material in the nucleus of the cell found in DNA becomes the final protein message. This process starts with what is known as gene expression.
In prokaryotes, the process is similar but is unique to these types of organisms. The DNA is essentially the same but the methods that exist to express each gene are different than is the case with eukaryotes. Messenger RNA is also essentially the same but gets processed differently. They do not need to be processed in order to leave the nucleus because these organisms do not have them. The ribosomes are also located in the main cytoplasm component of the cell, where the mRNA is already there to be translated.
Often translation and transcription happen at the same time so that the mRNA does not have a chance to be degraded in the cell cytoplasm.
Part of the processing of mRNA involves a couple of different steps. The first piece of mRNA made is called the primary transcript or pre mRNA. It is made of exons, which are segments of RNA that actually get kept to participate in translation. It is also made of introns, which are segments of RNA that are not translated. Through the process of mRNA splicing, the right length or mRNA is made with the right nucleotides in it. There are caps put on both ends of the mRNA molecule, which isn’t something that happens in prokaryotes.
Translation takes place in both prokaryotes and eukaryotes in roughly the same way. Ribosomes are protein making factories in all cells. In the prokaryote, these are found in the cytoplasm. In the eukaryote, they are found attached to a lipid structure called the endoplasmic reticulum. Ribosomes dot the endoplasmic reticulum, making it look rough in texture under the microscope. For this reason, these areas of endoplasmic reticulum are called rough endoplasmic reticulum.
In the ribosome, there is a site on the mRNA molecule containing what’s called a start codon. This is the signal for the translation process to occur. One section at a time, the mRNA molecule is made. Each section, called a codon, is a secret code for a specific amino acid. As we will talk more about, the process also involves transfer RNA or tRNA, which is a small segment of RNA that has a specific amino acid attached to it. The tRNA is a helper molecule that guides the amino acid from the beginning of the polypeptide chain to the end of the chain. There are similar stop codons that tell the whole process to stop and so that the polypeptide chain can be released from the ribosome. It is after this that post-transcription modification of the polypeptide chain happens.
GENETIC CODE
The genetic code is how the DNA molecule gets read. It is the same for every organism on earth, regardless of the type. As you will learn, there are four different nucleotides that make up the DNA structure. These are called adenosine, thymine, guanine, and cytosine. These are called A, T, G, and C. In RNA, the thymine is replaced by uracil.
These nucleotides are not read or transcribed individually but are arranged in sets of three called codons or triplet codon. This allows for enough different codons to account for the 20 amino acids that go into making a protein plus certain codons such as AUG, which is the RNA codon called the start codon. Some triplets code for the same amino acid. The different codons are collectively called the genetic code. The whole idea behind what constituted a codon, which is the basis of the genetic code, is called the triplet hypothesis, first uncovered by George Gamow in the 1950s. Gamow deduced that triplets must be involved based on the idea that a codon made of two nucleotides did not give the diversity necessary for all of the available amino acids. More nucleotides per codon seemed excessively complicated. Three, however, left a possible 64 different choices, which was at least in the ballpark of what was necessary. This was later proven in the 1960s, when researchers finally cracked the code.
When the genetic code works the way it’s supposed to, there is a start codon called AUG that usually codes for the methionine amino acid. This methionine might later get trimmed off. Each triplet gets read in turn until a stop codon is read. There are three stop codons, which are UAA, UGA, or UAG. None of these code for an amino acid but instead are simply signals for the polypeptide to drop off. If there are nucleotides after the stop codon is reached, they don’t get translated into anything. One thing that’s important to remember is that all of this must be read properly. If the reading frame is off in anyway, nothing about the resulting protein will make any sense. There are no breaks between codons, such as you would see when you read the words on a page. Instead, they appear as an unending chain that must be read in the proper groups of three. The start codon sets the reading frame properly.
Essentially the genetic code is the same for all organisms on earth but there are rare exceptions. A few bacteria have minor differences in a single or a few codons that instead code for different amino acids than all other organisms but this is not at all a common thing. All of this points back to the idea that every organism on earth started with a common evolutionary organism at some point in earth’s very distant past.
HOW GENES BECOME TRAITS
Sometimes, it is easy to see how a gene becomes a trait, while it is harder to see in other circumstances. In some cases, a single gene codes for a single enzyme in a biochemical pathway. If the enzyme isn’t present, nothing happens in the entire pathway and the end product of the pathway does not get made. In other cases, a single gene codes for a receptor protein on the cell surface. The blood types A, B, AB, or O, are determined by the proteins labeled as such on the red blood cell.
Remember, too, that in eukaryotes there are two copies of each gene. One copy by itself is called an allele. Some alleles are dominant so they get expressed no matter what the other allele is. Other alleles are recessive, so they don’t get expressed unless it’s corresponding allele is also the same recessive allele. Still others are called codominant because both alleles get expressed at the same time. Finally, most traits in an organism are polygenic, meaning that more than one gene goes into making the phenotype. There isn’t, for example, just one tall gene and one short gene that determines who will be tall and who will be short.
An example of a dominant trait is that for brown eyes, which is dominant over the blue eyed gene. Blood groups represent an example of codominance, where each gene is as important as the other. Recessive genes are best understood in terms of the disorders we often see that are recessive in nature. Diseases like phenylketonuria and cystic fibrosis are examples of diseases where two recessive genes need to be present. There are a great many other traits that depend on multiple genes, such as the risk a person has for heart disease or diabetes.
• The study of genetics in some form has existed since ancient times, when philosophers thought about dominant and recessive traits, and speculated on how these might get passed to one’s offspring.
• The first uses of genetics happened when individuals worked with plants to create plant hybrids.
• Mendel’s work on pea plants was done without his knowing about the existence of genes.
• There are many branches of genetics, including classical, molecular, and population genetics.
• The basic unit of genetics is the gene. Genes are arranged on chromosomes. The chromosomes are circular in prokaryotes and linear in eukaryotes.
• Chromosomes must pack themselves into small spaces. This happens through supercoiling in prokaryotes and through the use of histone proteins in eukaryotes.
• The molecular expression of genes starts with transcription, proceeds with translation, and ends with post translational modification of proteins.
• The genetic code is based on codons that themselves are made from triplets of nucleotides that together determine what amino acid gets made from a specific segment of DNA or RNA.
• There are some traits based on single genes, while most traits in complex organisms depend on the interaction of several genes at once.
1. Which pre Mendelian contributor to the understanding of genetics defined the differences between dominant and recessive traits?
a. Aristotle b. Hippocrates c. HaLevi d. Al Jahiz
2. What did Anaxagoras believe in his contribute to genetic theory?
a. The concept that the entire organism contributed to the traits in its offspring.
b. The idea that the organs of an organism was pre-formed in the sperm or egg.
c. The idea that the organs of an organism developed as the organism itself developed.
d. The idea that there are dominant and recessive traits.
3. According to classical genetics, what forms the basic unit of trait transmission from parents to offspring?
a. Chromosomes b. Genomes c. Nucleotides d. Genes
4. According to classical genetics, what is the word that defines the fact that traits can be passed from parents to offspring?
a. Heredity b. Gene variation c. Gene mutation d. Phenotype blending
5. Which field of genetics has contributed most to modern evolutionary theories?
a. Molecular genetics b. Classical genetics c. Population genetics d. Biotechnology
6. Which aspect of population genetics ultimately causes variation in a population?
a. Genetic drift b. Mutation c. Natural selection d. Gene flow
7. In eukaryotic multicellular organisms, how is it that every cell is unique?
a. Every cell in the body has different DNA instructions in them. b. Each cell in the body has a unique karyotype.
c. Each cell makes the same proteins from their DNA but the proteins get degraded in not necessary for the cell to function. d. Each cell has the same DNA but differ in which genes get turned on and which do not.
8. What is not a product of a segment of DNA or gene?
a. Carbohydrate b. Transfer RNA c. Ribosomal RNA d. Protein
9. Which amino acid gets made every time the messenger RNA molecule gets read first in the ribosomes?
a. Histidine b. Glycine c. Valine d. Methionine
10. How are most genetic traits determined?
a. By several different genes acting together b. By the presence of an autosomal dominant gene c. By the presence of two autosomal recessive genes d. By the presence of codominant genes