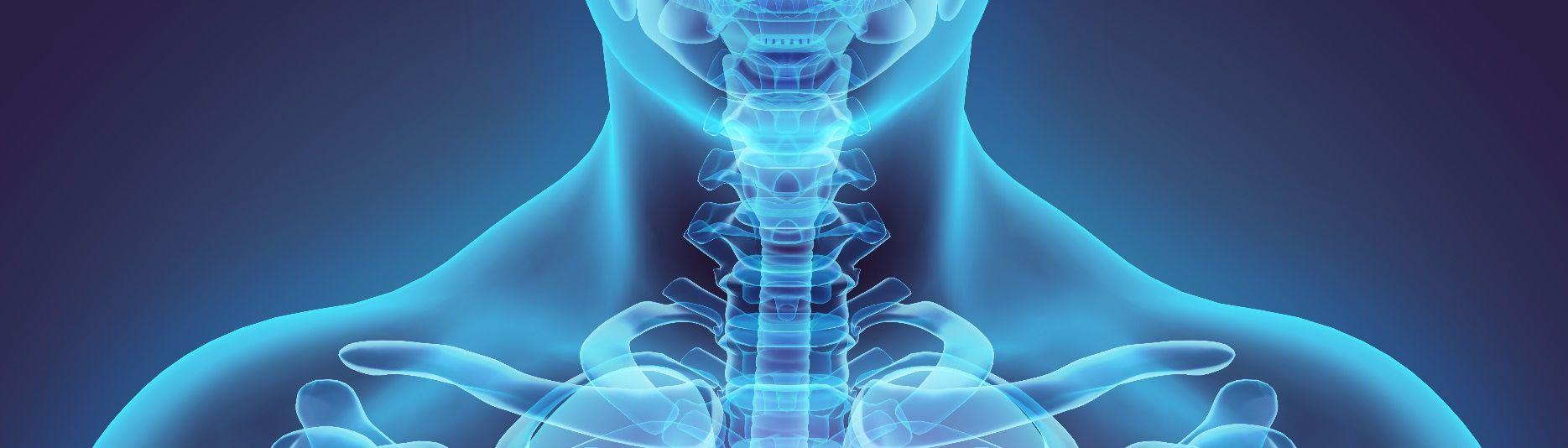
13 minute read
Cell Junctions
from Paramedic Exam
by AudioLearn
PEROXISOMES
These are vesicular organelles that break down lipids and other chemicals through the act of oxidation. They can self-replicate and are heavily present in both the kidneys and the liver, where detoxification is important. They contain various oxidation molecules.
Advertisement
CELL JUNCTIONS
These are the structures that connect cells to other cells so that tissues and organs can stay together. There are three major kinds of cell junctions:
A. Desmosomes—these are made from proteins and look like disks in the plasma membrane. There are protein fibers extending from the plasma membrane into the
cytoplasm. These act as connection between cells that undergo a great deal of stress, such as the heart muscle tissue and skin.
B. Tight junctions—these are highly connective between cells, forming a bond that completely encircles the cells they connect. They do not allow material to filter between cells. Tight junctions are found in the digestive tract so that nothing goes past the cells other than the material that goes through the cells themselves.
C. Gap junctions—these structures are actually slim tunnels that connect the cytoplasm of two cells next to each other. They consist of proteins known as connexons. The structures are so narrow that only small molecules and ions can pass from cell to cell. In addition, electrical impulses are allowed to go from one cell to another without interruption.
CELL METABOLISM
Cell metabolism represents the sum of all of the chemical reactions that occur within the cell. There are two major cell reactions that take place that can be defined as part of the metabolism. Catabolic reactions or “catabolism” are responsible for breaking down or
decomposing a molecule into its parts. Anabolism or anabolic reactions are the opposite of catabolism and represent the formation of a larger molecule from its component parts.
As mentioned, the major energy molecule of the cell is ATP or adenosine triphosphate. Each ATP molecule is recycled about 2-3 thousand times a day to make enough energy for the cell. Because ATP cannot be stored, it must be consumed shortly after it is made. This allows for about ten million ATP molecules to be made and consumed every second in a single cell.
Another important molecule in the process of cell metabolism is flavin adenine dinucleotide or FAD, which forms FADH2 after the addition of two hydrogen molecules. It participates in the Krebs cycle (which is the main metabolic process occurring in aerobic respiration). NAD or nicotinamide adenine dinucleotide reduces to NADH. NAD is use in glycolysis (anaerobic respiration) and the Krebs cycle (aerobic respiration) as well as in the electron transport chain,
allowing for a lot of ATP to be made. NADP is use in fatty acid and nucleic acid synthesis, which are anabolic reactions.
CELLULAR RESPIRATION
This is the process of taking sugar molecules, turning them into glucose, and then metabolizing the glucose into CO2, water, and usable ATP. There are two major parts to cellular respiration.
The first is glycolysis and the second is the citric acid cycle or Krebs cycle. These are called cellular respiration because oxygen is used in the final step to take on the last electrons, forming the CO2 that leaves the cell and is ultimately expired from the body.
Glycolysis takes place anaerobically and happens in the cytoplasm. It takes glucose and converts it into two three-carbon molecules called pyruvate. It is pyruvate that is fed into the Krebs cycle in the mitochondria. Two molecules of ATP are necessary for this reaction to take place; however, four are created so there is a net gain of two ATP molecules. There are two NADH molecules made that participate in the electron transport chain (also located in the mitochondria). If glycolysis happens without the Krebs cycle, it is referred to as anaerobic
respiration, producing lactic acid that ultimately accumulates and leads to muscle cramping or soreness.
The Krebs cycle takes two molecules of pyruvate and breaks them down further. It is a cycle that participates in energy production in both animals and plants. It takes place inside the mitochondria and involves a series of reactions that generate ATP and creates CO2 and water. The Krebs cycle also makes NADH and FADH2, which go into the electron transport chain to make ATP. While the Krebs cycle makes energy itself, the most energy-producing aspect of cellular aerobic metabolism is the electron transport chain.
The electron transport chain is extremely complicated. It is a series of oxidation-reduction reactions or redox reactions that transfer electrons from one molecule to another, making ATP in the process. The redox state of the cell describes the balance of both NAD+/NADH and NADP+/NADPH in the cell or organ. There are several diseases that cause an abnormal redox state in the cell, including sepsis, shock, and hypoxia.
BUILDING BLOCKS OF THE CELL
There are three major classifications of molecules found within the cell. These include lipids, carbohydrates, and proteins. Lipids are considered fatty acids and the molecules that can be derived from fatty acids. These molecules will store energy and will become the structural components of the plasma membranes and other membranes within the cell. Lipids also act as signaling molecules in the cell.
Carbohydrates consist mainly of carbon, oxygen, and hydrogen. There are monosaccharides,
which consist of a single sugar molecule including glucose, fructose, and galactose. There are disaccharides, which consist of two sugar molecules attached to one another (such as sucrose and lactose). There are numerous polysaccharides that are made from more than two sugar molecules. In humans, the main polysaccharide is glycogen, which is made from many molecules of glucose strung together. Other polysaccharides are starch and cellulose.
Proteins are made from amino acids and composed mainly of nitrogen, carbon, hydrogen, and oxygen. The two other atoms found in some amino acids are phosphorus and sulfur. There are twenty different kinds of amino acids used by the human body. In various combinations, amino acids combine to make the basic protein structure. There are several structures associated with the protein molecule. There is the primary structure, which is just the polypeptide sequence in no particular shape. The secondary structure is formed by the hydrogen bonds between amino acids to create a semblance of a shape. The tertiary structure is the three-dimensional shape of the polypeptide.
Most enzymes in the cell are proteins that catalyze a chemical reaction. Enzymes allow for reactions to occur that wouldn’t have otherwise occurred in nature. There are some enzymes, however, that are made from RNA, called ribozymes or RNA enzymes. Like other enzymes, they catalyze certain biochemical reactions.
ACID BASE PHYSIOLOGY
Acid base balance is extremely important to the body as it controls the pH of the blood and the cells, where many reactions take place. Because there is a very narrow range of pH in which reactions can occur, the body has several buffering systems that maintain the pH at what’s called the physiological pH. Buffers prevent radical changes in fluid pH by dampening the effect of hydrogen ions on the system. Weak acids and weak bases will make the best buffers by taking up hydrogen ions or hydroxyl ions. It takes just seconds for the body’s buffering systems to adjust the pH when necessary.
The fastest buffering system is the respiratory tract. By exhaling CO2 from the body, the pH can
rise in cases of acidosis. The kidneys can also combat acidosis by increasing hydrogen ion excretion and by increasing the reabsorption of bicarbonate, which takes hours to days to have
an effect but which will also serve to increase the pH in cases of acidosis. Plasma proteins, phosphate, bicarbonate, and carbonic acid all act as buffer systems. Protein buffer systems are predominately intracellular, while the other buffering systems are extracellular.
Almost any protein can function as a buffer. Amino acids contain positively charged amino groups and negatively charged carboxyl groups. In this way, a protein can be a weak acid or a weak base. Almost all of the buffering in the cell comes from protein buffering, while twothirds of the blood’s buffering comes from proteins.
Hemoglobin is a protein that resides inside RBCs (red blood cells) and accounts for a full third of the cell’s mass. During the conversion of CO2 into bicarbonate, hydrogen ions are liberated and must be buffered. Oxygen is dissociated and hemoglobin is reduced, leading to the
maintenance of a normal pH. This happens at the tissue level. In the lungs, CO2 is reformed and diffuses out into the alveoli. Hemoglobin acts as the buffer there. Hemoglobin accounts for a major buffering system in the bloodstream.
The phosphate buffer also exists in the blood. Sodium dihydrogen phosphate is a weak acid, while sodium monohydrogen phosphate is a weak base. The two molecules move back and forth with each other, picking up a hydrogen ion (in the case of the weak basic form) or picking up a hydroxyl ion (in the case of the weak acid form). This buffers the blood from extremes in pH.
The bicarbonate-carbonic acid buffer works similarly to the phosphate buffering system. Bicarbonate will pick up a hydrogen ion forming salt and carbonic acid (which is a weak acid). In the same way, carbonic acid will pick up a hydroxyl ion to form a weak base and water, leading to a weaker base than would be seen with the hydroxyl ion alone. The bicarbonate system
protects primarily against acidic changes in the bloodstream versus an alkaline change (as there is much more bicarbonate than carbonic acid in the bloodstream).
Most of the waste products in the body, such as ketones and lactic acid, are acids, making it more important to protect the blood and the rest of the body from acidic changes. Carbonic acid levels are controlled by the amount of CO2 expired in the lungs, while the bicarbonate levels are controlled through the renal system. Bicarbonate can be more or less excreted by the kidneys, affecting its levels.
REGULATION OF THE ACID-BASE SYSTEM IN THE BODY
As mentioned, both the kidneys and the lungs contribute to the acid-base system of the body. The lungs regulate the blood levels of carbonic acid because CO2 plus water makes carbonic acid; CO2 and carbonic acid are in equilibrium in the bloodstream. When CO2 levels rise, the excess CO2 will react with water, forming carbonic acid to lower the pH. The individual will respond by increasing the rate or depth of the respirations to blow off CO2. This adjusts the pH to normal levels. Hyperventilation makes the blood too alkaline because CO2 is blown off and carbonic acid levels drop. Rebreathing air into a paper bag will increase the CO2/carbonic acid levels in the bloodstream.
Minor adjustments in the breathing rate and depth are usually sufficient to adjust the pH of the bloodstream in the pulmonary capillaries. If one doubled the respiratory rate, the CO2 difference would increase the blood pH by 0.2. This happens when a person exercises strenuously for a period of time. Exercise increases the lactic acid production and the production of CO2 at the cellular level and the breathing rate increases to counteract this and blow off the extra CO2 that is gained through the increase in cellular respiration.
There are chemoreceptors in the walls of the aorta and in the carotid arteries. They regulate the respiratory rate by sensing the CO2 level changes in the blood. Other sensors are located in the brain so that changes in the pH of the CSF affect the respiratory center in the medulla oblongata, changing the respiratory rate to normalize the pH level.
Hypercapnia happens with COPD, CHF, pneumonia (and anything else that impairs the release
of CO2 in the lungs). Certain drugs and holding one’s breath can do this as well. Hypocapnia (low CO2 levels) will occur with hyperventilation. This drives off CO2 levels, leading to respiratory alkalosis.
The kidneys have a more complex buffering system that requires more time to normalize blood pH. Diarrhea and carbonic anhydrase inhibition will leach bicarbonate from the bloodstream or
will otherwise decrease the bicarbonate level. Blood bicarbonate levels will also be decreased
bicarbonate conservation).
Hydrogen ions compete with potassium in the renal tubules. If there is excess potassium, the potassium takes the place of hydrogen ions in the renal filtrate and fewer hydrogen ions can participate in the conservation of bicarbonate, leading to a net loss of bicarbonate. Less potassium leads to conservation of bicarbonate. Remember that bicarbonate is not permeable in the tubules so it must be conserved rather than reabsorbed.
The ultimate goal of the buffering systems in the body are to keep the physiological pH, which is between 7.35 and 7.45. Buffers act to prevent sudden shifts in pH by absorbing or taking on hydrogen or hydroxyl ions. Most buffers are weak acids or weak bases (or, in the case of proteins, are a combination of the two). The primary buffering system of interstitial fluid is the bicarbonate buffering system, while the intracellular proteins primarily buffer the interior of the cells.
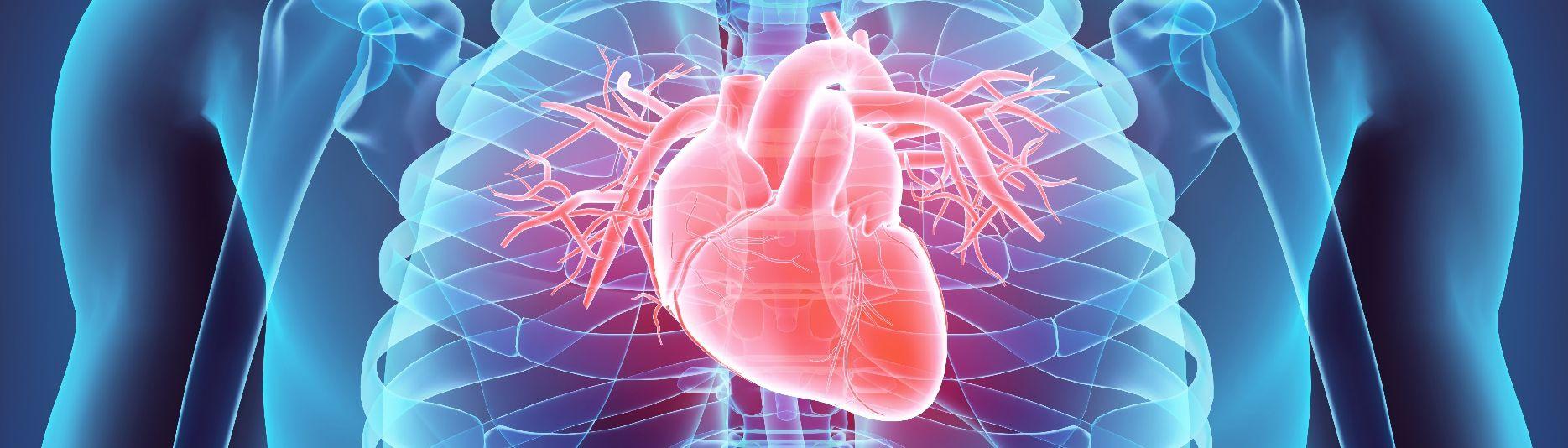
ACID BASE DISORDERS
Anyone with a blood pH of less than 7.35 is said to be acidotic or has acidosis. Anyone with a
blood pH of greater than 7.45 is said to be alkalotic or has alkalosis. A blood pH of less than 7.0 for a prolonged period of time is fatal. Typical symptoms of acidosis include confusion, headache, lethargy, and fatigue. Typical symptoms of alkalosis include nausea and vomiting, cognitive impairment, extremity and facial numbness, and muscle twitching. Both acidosis and alkalosis can be caused by metabolic or respiratory diseases.
Because the levels of carbonic acid and CO2 are in sync with one another, the carbonic acid
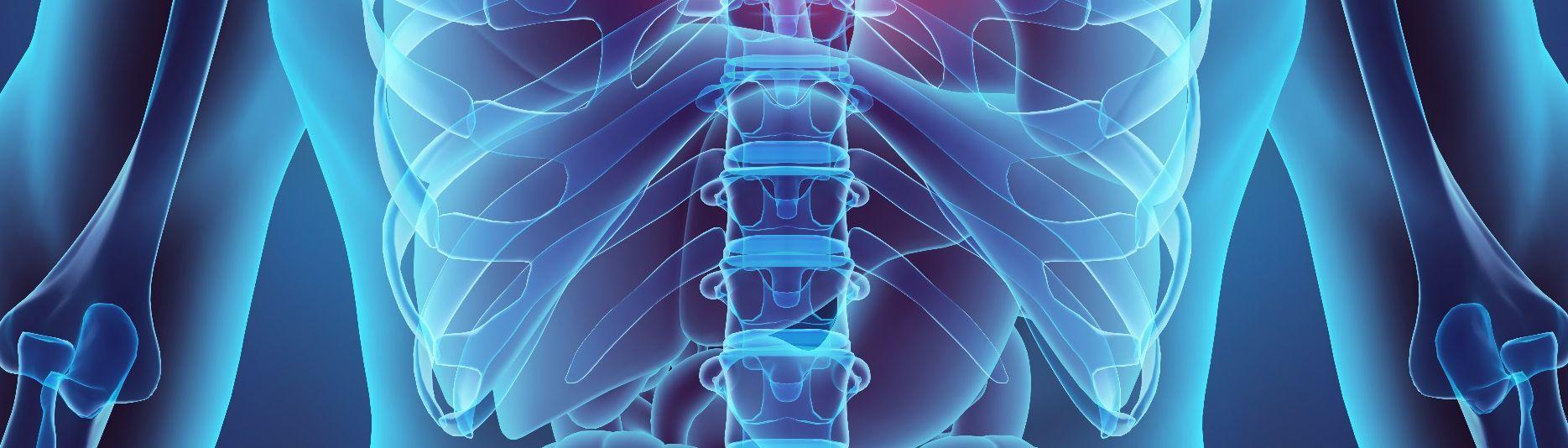
There are four major classifications of acid base disorers: metabolic acidosis, metabolic
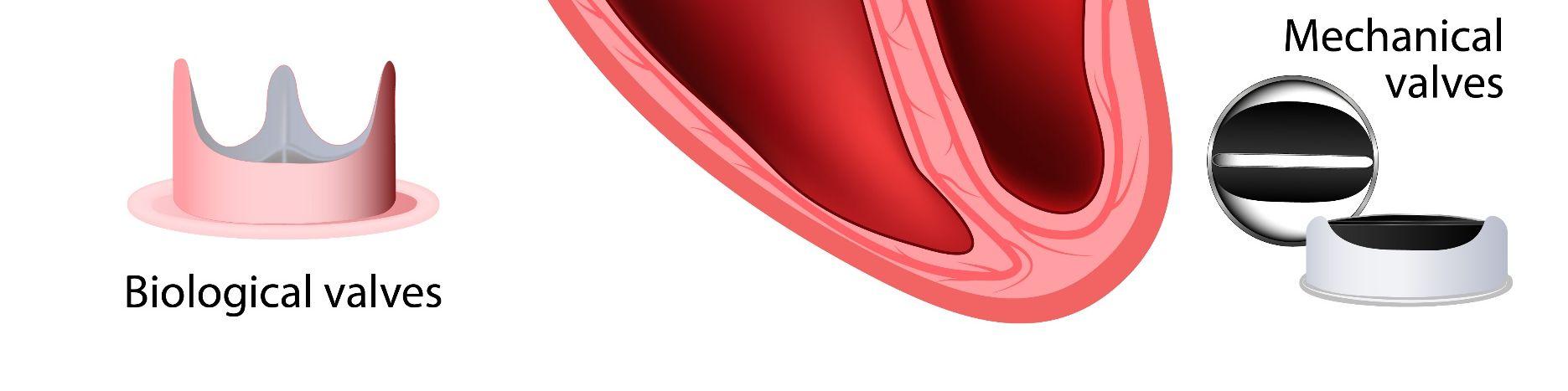
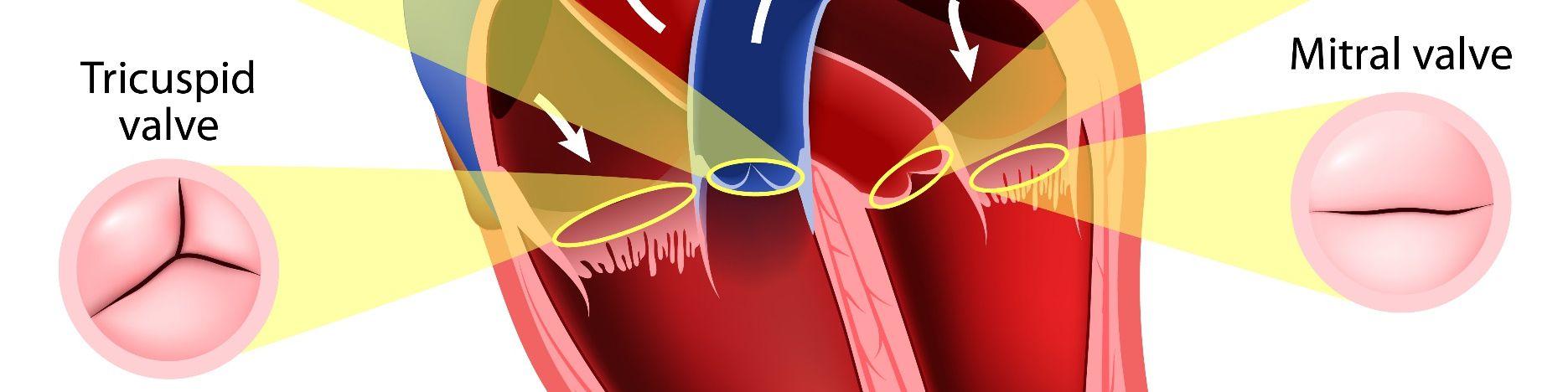
Metabolic acidosis is related to the bicarbonate level, which is too low. The most common cause of metabolic acidosis is the presence of organic acids or ketones in the bloodstream.
Other causes include diarrhea (with a loss of bicarbonate in the stool), uremia (with an excess of lactic acid, sulfuric acid, and phosphoric acid), diabetic ketoacidosis (with increase ketones), strenuous exercise (with an increase in lactic acid), methanol toxicity (with an increase in formic acid), paraldehyde toxicity (with an increase in beta-hydroxybutyric acid), isopropanol toxicity (with an increase in propionic acid), ethylene glycol toxicity, and aspirin/salicylate toxicity. As you can see, the first three causes are medical, while the last five are toxicology-related. Hyperkalemia will cause bicarbonate to be less conserved in the kidneys as well.
Metabolic alkalosis happens when the pH is above 7.45 from too much bicarbonate in the body or a relative lack of hydrogen ions. An influx of bicarbonate or other antacids for things like
reflux disease can lead to metabolic alkalosis. Cushing’s disease can cause chronic metabolic alkalosis by increasing aldosterone levels. This causes an increased loss of potassium, which increases the bicarbonate level. Potassium depletion from diuretics, laxative abuse, and hydrochloric acid losses from the stomach during vomiting can all lead to metabolic alkalosis.
Respiratory acidosis stems from too much CO2 in the body. COPD, pneumonia, and congestive
heart failure can cause respiratory acidosis. Respiratory alkalosis results from a CO2 deficiency; the most common cause of this is hyperventilation (which can be caused by many things).
Salicylate toxicity will cause a compensatory respiratory alkalosis because the lungs are compensating for the initial acidosis.
There are compensatory mechanisms in place that attempt to keep the pH within a very normal range, including respiratory, renal, and buffering mechanisms. Most of the time, these work effectively but, when they fail, the body is unable to compensate.
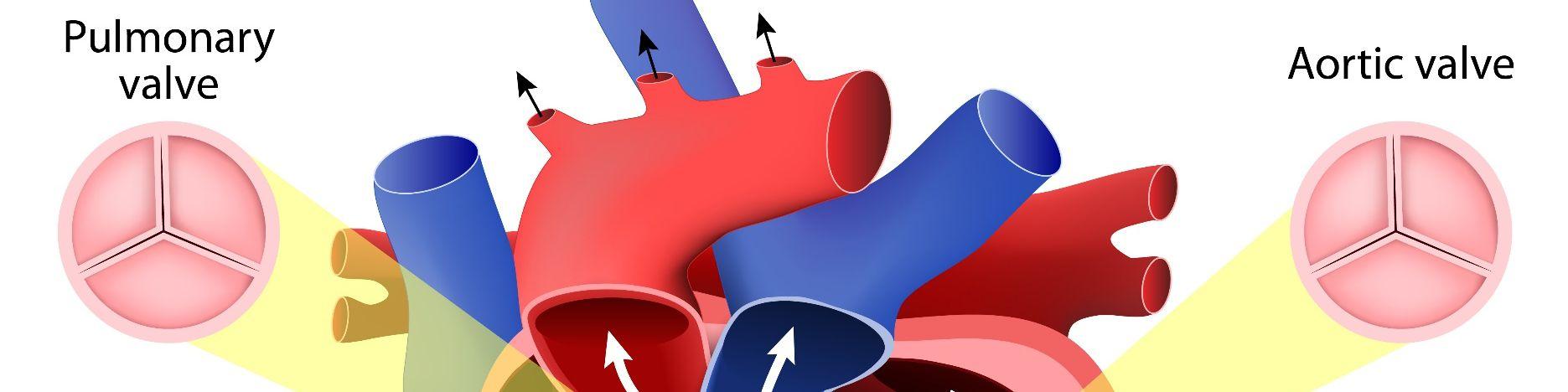
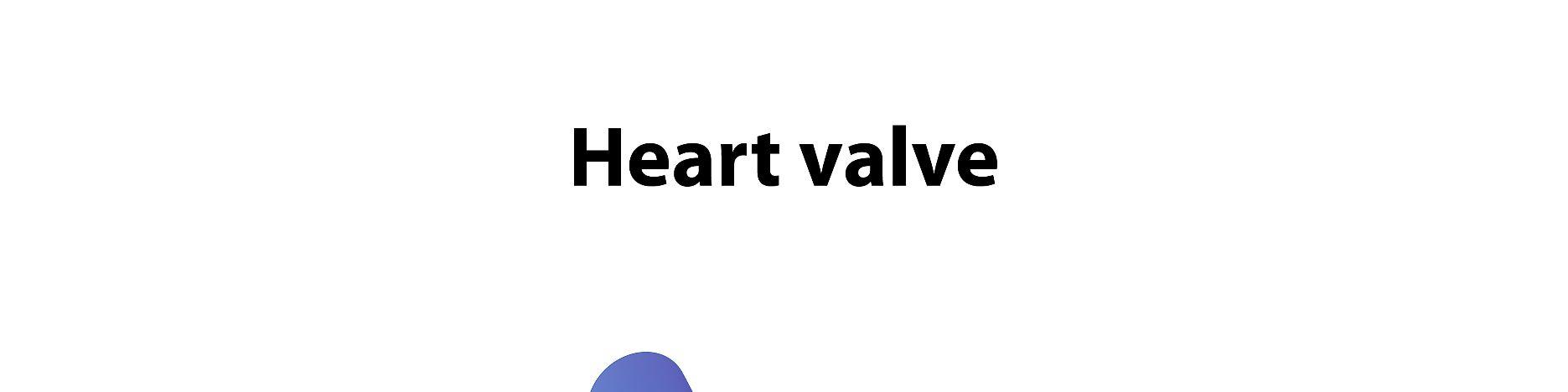