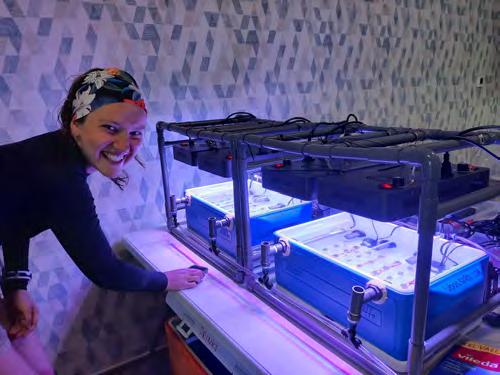
13 minute read
2020 ACRS Research Awards
Each year the ACRS supports the research of up to five students through provision of the ACRS Research Awards. The most outstanding proposals are awarded the Terry Walker Prize ($4,000) or the Danielle Simmons Prize ($4,000). Up to three additional ACRS Research Awards of $2,500 each are also awarded. Below we present you the recipients of the 2020 ACRS Research Awards. In the following pages, you can read articles contributed by each awardee.
Terry Walker Prize
Advertisement
RACHEL ALDERICE
Is low oxygen stress management key to photoprotection in reef-forming corals?
Unfortunately, due to the pandemic, Rachel was unable to conduct her experiments. However, data collection will be underway soon, and this research will build upon understanding of how corals manage deoxygenation stress and regulate their host pigments during bleaching – a critical step in developing genetic and optical biomarkers of enhanced stress-resilient corals.
Danielle Simmons Prize
AMELIA DESBIENS
The missing link: uncovering key predators of crown-of-thorns starfish juveniles
Resolving the impact of cryptic predation on crown-of-thorns starfish. CoTS research has focused on larvae and adult stages for decades, but juveniles could provide the missing link in our understanding of CoTS population cycles. Small predators, such as crabs, may be important controls of juvenile CoTS numbers and later outbreaks of adults.
ACRS Research Awards
MOLLY MOUSTAKA
Intertidal mangroves as fish nurseries: fact or fallacy?
Understanding how seascape configuration influences secondary productivity in coastal ecosystems.
MARGENA MARZONIE
Keeping pace with climate change with a little help from symbiotic algae
The influence of symbiont genetic diversity on thermally distinct coral reefs
Picture opposite page: Collecting coral spawn slicks ©Christina Langley
Danielle Simmons Prize
Predators | Crown-of-thorns- starfish | Coral rubble
Coral reefs across Australia, and the world, have been decimated by population outbreaks of the coral-eating crown-of-thorns starfish (also known as CoTS). However, there is now increasing recognition that natural CoTS predators may have the capacity to control and prevent outbreaks in certain locations. Despite this, relatively little is known about which predators are capable of consuming CoTS, especially during their small and vulnerable juvenile stage. CoTS research has focused on larvae and adult stages for decades, but juveniles could provide the missing link in our understanding of CoTS population cycles.
By Amelia Desbiens University of Queensland
“Key rubble-based predators have the potential to suppress juvenile populations and could therefore be a crucial bottleneck in the growth and progression of adult CoTS outbreaks.”
CoTS juveniles live for several months in coral rubble habitats alongside a plethora of other hidden cryptic organisms. This habitat is home to a wide variety of crustaceans, molluscs, echinoderms, polychaetes and other worms, as well as cryptobenthic fishes. My project aims to identify which of these organisms are capable of consuming CoTS juveniles. Key rubble-based predators have the potential to suppress juvenile populations and could therefore be a crucial bottleneck in the growth and progression of adult CoTs outbreaks.
With support from the Australian Coral Reef Society, I am conducting surveys of rubble communities across Heron Reef. This involves deploying 3Dprinted Rubble Biodiversity Samplers (RUBS) and active searches through
Above: Rubble habitats are a natural part of coral ecosystems, housing a huge diversity of cryptic organisms. © Kenny Wolfe 2021.
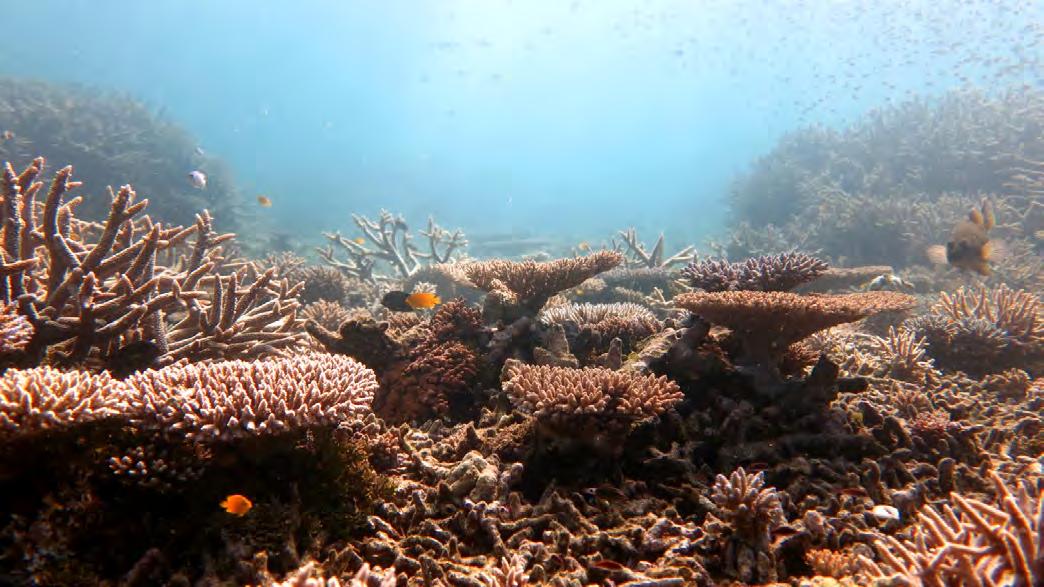
rubble beds on SCUBA. Many enjoyable dives are spent searching through rubble habitats, flipping rubble pieces to reveal the secret homes of these cryptic organisms. These surveys give us an indication of which species are common or rare in these habitats.
Each species collected from the rubble is offered CoTS juveniles as food in tanks in the lab. These predation tests are repeated across a range of CoTS sizes to understand how consumption may change as juveniles grow and transition to become large coral predators. Understanding which predators consume CoTS in rubble before they consume coral, and how abundant they are in natural environments, will help us to predict whether rubble-dwelling predators can suppress juvenile CoTS populations and thus reduce outbreaks of CoTS adults from developing.
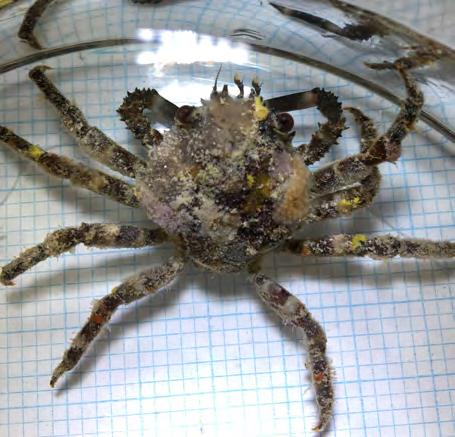
In a study conducted at Heron Island in September 2021, more than 100 species of cryptic animals were identified in coral rubble. Several of these species demonstrated capacity to prey on CoTS juveniles in the lab. Three different species of crabs attempted to eat CoTS juveniles that were ~10 months old. After just three days, juveniles were found with missing arms and severed bodies. Interestingly, these “old” juveniles were never eaten in full, and regenerated their lost limbs over several months when the predators were removed.
In 2022, more comprehensive rubble surveys and predation experiments will expand on these initial findings. I will conduct predation experiments with younger and smaller CoTS juveniles, which are more likely to be eaten than the 10-month old juveniles I used first. This will provide important information on size thresholds in predation. The outcomes of this research will have important implications for our understanding of predation as a mechanism of CoTS population control.
Above: Potential CoTS predator (Schizophrys sp.) Acknowledgments
This research would not be possible without support from grants including the Danielle Simmons Award from the Australian Coral Reef Society and the Great Barrier Reef Foundation’s CoTS Control and Innovation Program. Many thanks also go to my supervisors Prof Peter Mumby, Dr Kennedy Wolfe and Dr Eva Plaganyi, as well as colleagues Dr Sven Uthicke and Dr Symon Dworjanyn for their ongoing assistance in supplying CoTS juveniles without which none of this work would be possible.
Below: Healthy juvenile CoTS © Daniella Ceccarelli Below: Juvenile CoTs attacked by predator. Predators can cause extensive damage to vulnerable CoTS juveniles that may take several months to recover.
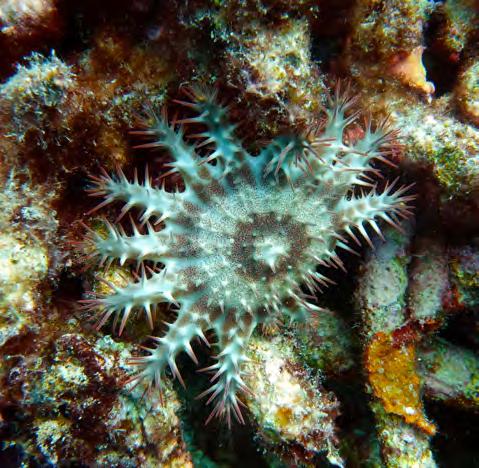
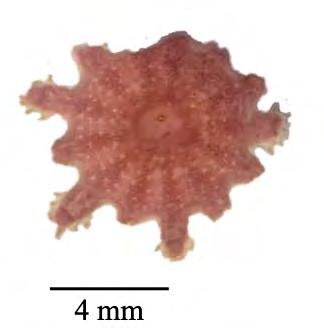
ACRS Research Award
Ontogenetic shift | Nursery habitats | Seascape connectivity
By Molly Moustaka University of Western Australia
Coastal habitats, such as macroalgae and mangroves, are often colloquially referred to as nurseries for fish. Juveniles of some fish species occupy these coastal habitats, exploiting the abundant food and shelter they offer, before migrating to coral reefs as adults.
The nursery value of mangroves has been the subject of considerable debate over the past two decades. The complex root systems of mangroves theoretically make ideal nurseries, and indeed much research from Caribbean systems supports this concept. Yet studies from the Indo-Pacific have produced mixed results, with these contradictions attributed to regional differences in tidal amplitude. In macrotidal systems, such as northwestern Australia, many mangrove habitats are unavailable during low tide, forcing fish into adjacent habitats.
Implementing ecosystem-based approaches to conservation and fisheries management depends on identifying, prioritising and preserving critical habitats and migration pathways used by fish. By exploiting the biolochronological properties of fish otoliths (earbones) this study aims to ascertain whether blackspot snapper (Lutjanus fulviflamma) recruiting to intertidal mangrove nurseries survive to adulthood, and whether the type of nursery habitat they occupy influences juvenile growth rates. This work forms a part of my broader PhD project which focuses on understanding how the configuration of habitats within a seascape influences the productivity of juvenile fish.
Tracking the migration of fish from one habitat to another (termed ‘ontogenetic shift’) is difficult due to the sheer size of the marine environment and the high mortality rates experienced by juveniles. Instead, with the support of the ACRS, I am using isotopic techniques to ‘look back in time’ at the habitats previously occupied by fish. Because fish otoliths grow incrementally over the life of a fish (like tree rings), their chemical composition at different points can yield information about the type of habitat that a fish lived in as a juvenile.
Using stable isotope analysis on the outer zone of otoliths (reflecting
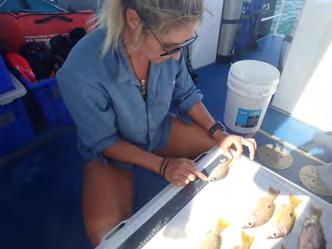
Above: Molly Moustaka measuring Lutjanus fulviflamma and Lutjanus carponotatus collected in the Dampier Archipelago, WA.
Below: Sectioned juvenile fish otolith (10x magnification). Each ring represents one day of life.
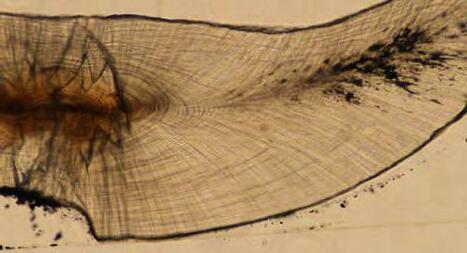
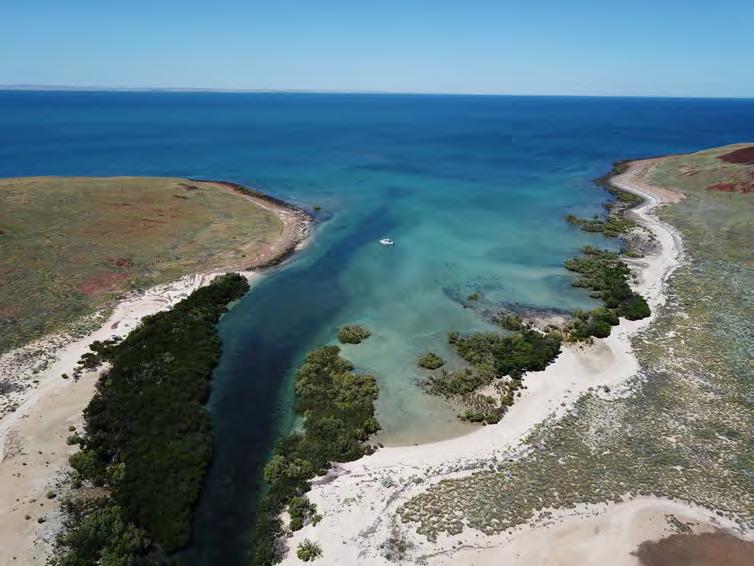
Above: Intertidal mangroves of Enderby Island in the Dampier Archipelago, WA © Dr William Robbins.
current habitat), I will obtain ‘chemical fingerprints’ for juvenile fish from mangroves and macroalgae habitats. For adult fish from the reef, both the inner zone (reflecting juvenile habitat) and the outer zone will be analysed, allowing me to match these ‘habitat fingerprints’ and ascertain the type of nursery habitat each fish previously occupied.
Additionally, the other otolith will used to determine the age of each fish by taking a thin section through the core and counting the number of rings present to investigate differences in juvenile growth rates between nursery habitat types.
In early 2021, we completed several field trips to the Dampier Archipelago during which we conducted underwater surveys of juvenile and adult fish communities in different habitats. We observed that juvenile L. fulviflamma were only found in mangrove and macroalgae habitats, and adults only on coral reefs; and subsequently collected fish from each of these habitat types.
Back in the lab, I extracted the otoliths and took tissue samples
from each fish. I embedded one otolith from each fish in epoxy resin and cut a thin section through the core of the otolith. In the coming weeks I will use these sections to age each fish by counting the number of rings in each otolith, allowing me to determine how fast L. fulviflamma from different habitats are growing. My next step is submitting tissue samples and otolith powder for stable isotope analysis, the results of which will reveal whether L. fulviflamma recruiting to intertidal mangroves are surviving to adulthood.
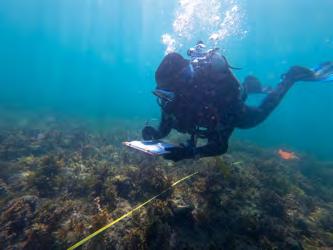
Above: Molly Moustaka conducting surveys of juvenile fish in the Dampier Archipelago, WA. Acknowledgements
My research would not be possible without the generous support provided by the Australian Coral Reef Society, the Australian Society for Fish Biology, the Holsworth Wildlife Research Endowment & the Ecological Society of Australia, the Jock Clough Marine Foundation, the Kieran McNamara World Heritage PhD Top-Up Scholarship, and the Department of Biodiversity, Conservation and Attractions. I am also grateful for the support and guidance provided by my supervisors Prof. Gary Kendrick, Dr Richard Evans, Dr Shaun Wilson, and Prof. Glenn Hyndes. Finally, I would like to thank my incredible volunteers, collaborators, and colleagues for their assistance in the field and invaluable advice.
ACRS Research Award
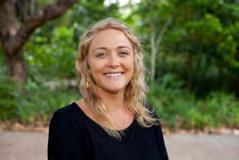
Heat Stress | Coral bleaching | Symbiosis
Keeping pace with climate change with a little help from symbiotic algae.
By Margena Marzonie James Cook University/AIMS
Marine heat waves have emerged as the predominant threat to coral reefs, causing widespread coral bleaching. Bleaching occurs when tiny symbiotic algae (i.e. symbionts) that live inside corals are stressed and expelled from the tissue. After the algae leave, the coral tissue is pale and its chalky skeleton exposed, hence the term ‘bleaching’. However, certain symbionts can withstand higher temperatures than others, so who the coral chooses as its symbionts can determine if a coral bleaches.
Over the last 6 years, severe marine heatwaves have affected Australia’s coral reefs, but not all individuals, species or reefs have responded equally to these events. Differences in bleaching are due to changing environmental pressures, including temperature, light or currents. However, even for two identical corals in the same environment, one can be bleached, and the other not. So, there are other mechanisms at play that the human eye cannot observe.
To understand why only some corals bleach and to assess impacts of further bleaching events, we must investigate bleaching at the scale of the coral host and the scale of whole ocean basins. One clue amidst these large-scale patterns, although tinier than the human eye can see, are the symbionts. If we learn how symbionts vary between reefs of high and low tolerance, we will better understand bleaching mechanisms.
As part of a team of scientists I went to the Coral Sea Marine Park (CSMP) in early 2020 during a bleaching event. The reefs there are some of the most isolated in the world, and well-removed from inshore impacts, making an ideal setting to look at bleaching tolerance. We collected three coral species and exposed them to shortterm heat stress experiments to identify how distinct reefs respond to temperature change. We used a portable aquarium system that fits on the back of a boat, using flowthrough seawater from the ocean. Over the month-long voyage, we collected over 700 samples from 13 reefs and measured coral health (a proxy for bleaching).
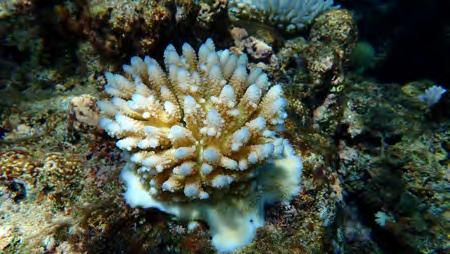
Above: One of the Acropora coral species that was abundant across the Coral Sea Marine Park. We collected 5 branches of each coral colony for experiments and genetic work. © Magena Marzonie
With ACRS funding, I extracted DNA from field-collected coral samples. I then sequenced a small region of DNA that helps identify different types of symbiont species. From there, I was able to input this sequencing information into a database, SymPortal, to discern which symbionts are abundant inside each coral.
We first found that some reefs were much more heat tolerant to experiments than others in the

Above: The author geared up to extract DNA from coral samples. You can see the small white coral branch collected from the field experiments. © Taylor Whitman
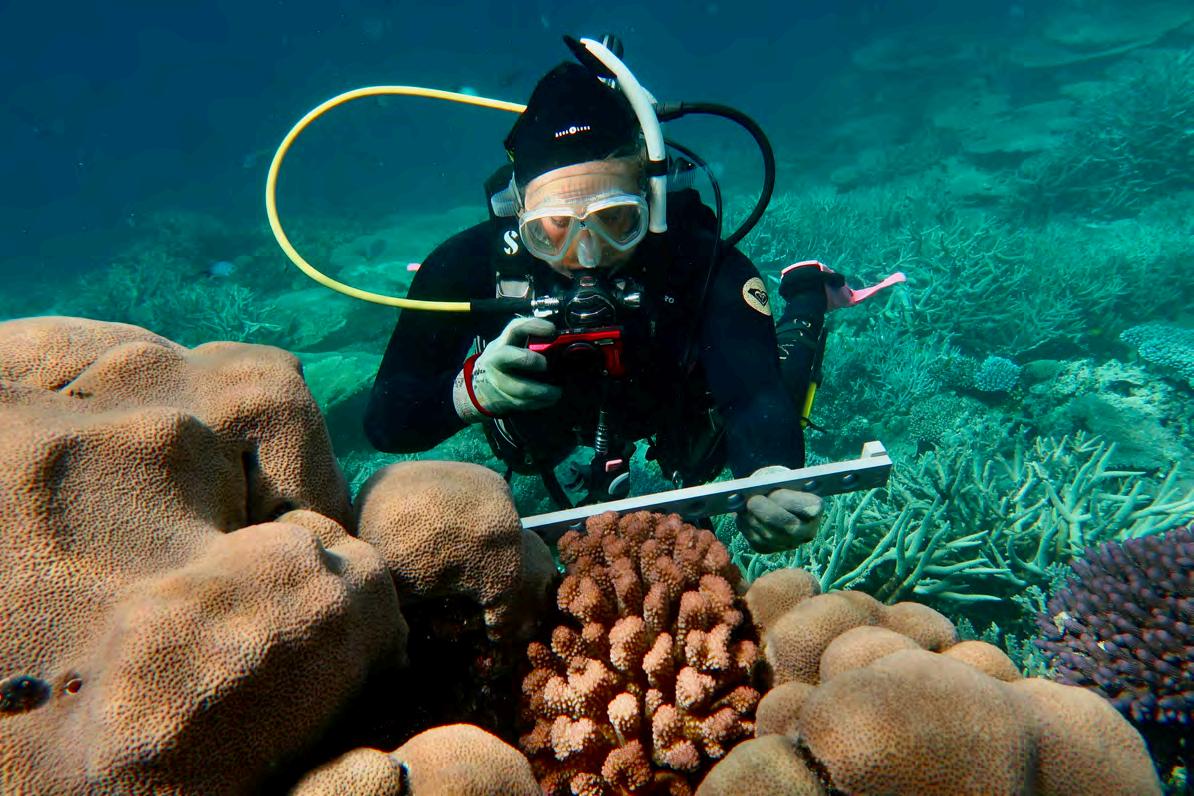
Right: The author taking photos and recording data for a Pocillopora coral prior to collecting a few branches to put into the heat stress experiment. © Johnston Davidson
CSMP. We also found that species had different responses to heat stress. The branching Acropora species was more susceptible to moderate temperatures above normal conditions (+5ºC). In contrast, the weedy Pocillopora species was more tolerant to moderate temperatures but hit a point of no return at extreme exposure (+7ºC).
Because we found differences in tolerance among reefs and species, we then looked to see if symbiont community structure explained this variation. Symbionts were highly specific to their host species and to individual reefs. Essentially, you can expect that the tiny algae inside Pocillopora, a weedy coral species, are very different to those inside a branching Acropora. You could also expect that corals collected in the northern reefs have different symbionts to southern reefs. This is evidence for why distinct reefs and species have different tolerances to marine heat waves and will help us better understand the mechanisms of coral bleaching in response to future heat waves.
Acknowledgments
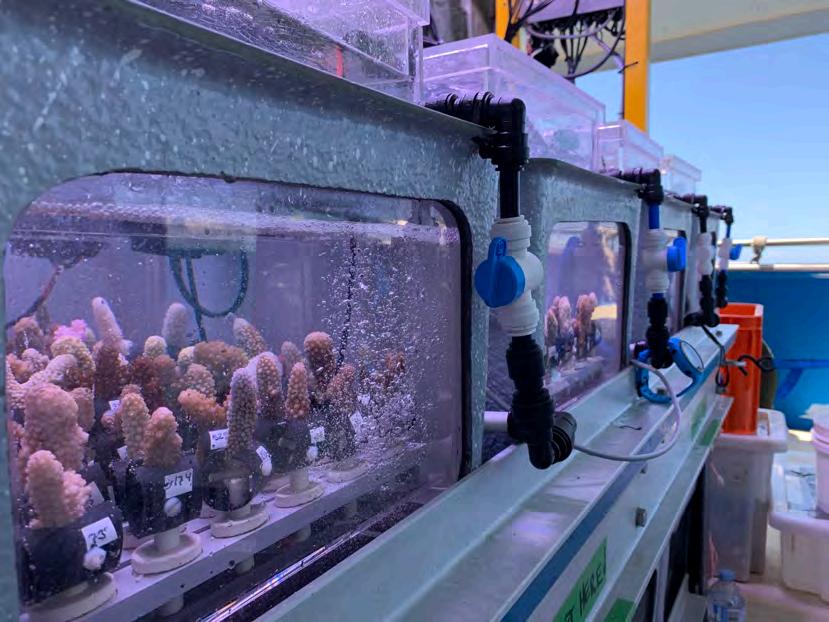
This project received funding from Parks Australia to Dr Hugo Harrison, Dr Andrew Hoey and Dr Morgan Pratchett and from the ARC Centre of Excellence for Coral Reef Studies and the Australian Institute of Marine Science. I am grateful to ACRS, the American Australian Association and AIMS@JCU for sequencing funding. Thank you to supervisors, Dr Hugo Harrison, Dr Line Bay and Dr David Bourne and collaborators Dr Matthew Nitchske and Luke Morris. I thank Dr Benjamin Hume (SymPortal) for bioinformatic support. I pay respects to the Marian Mer, Bindal and Wulgurukaba peoples as the Traditional Owners of land and sea country where I work.