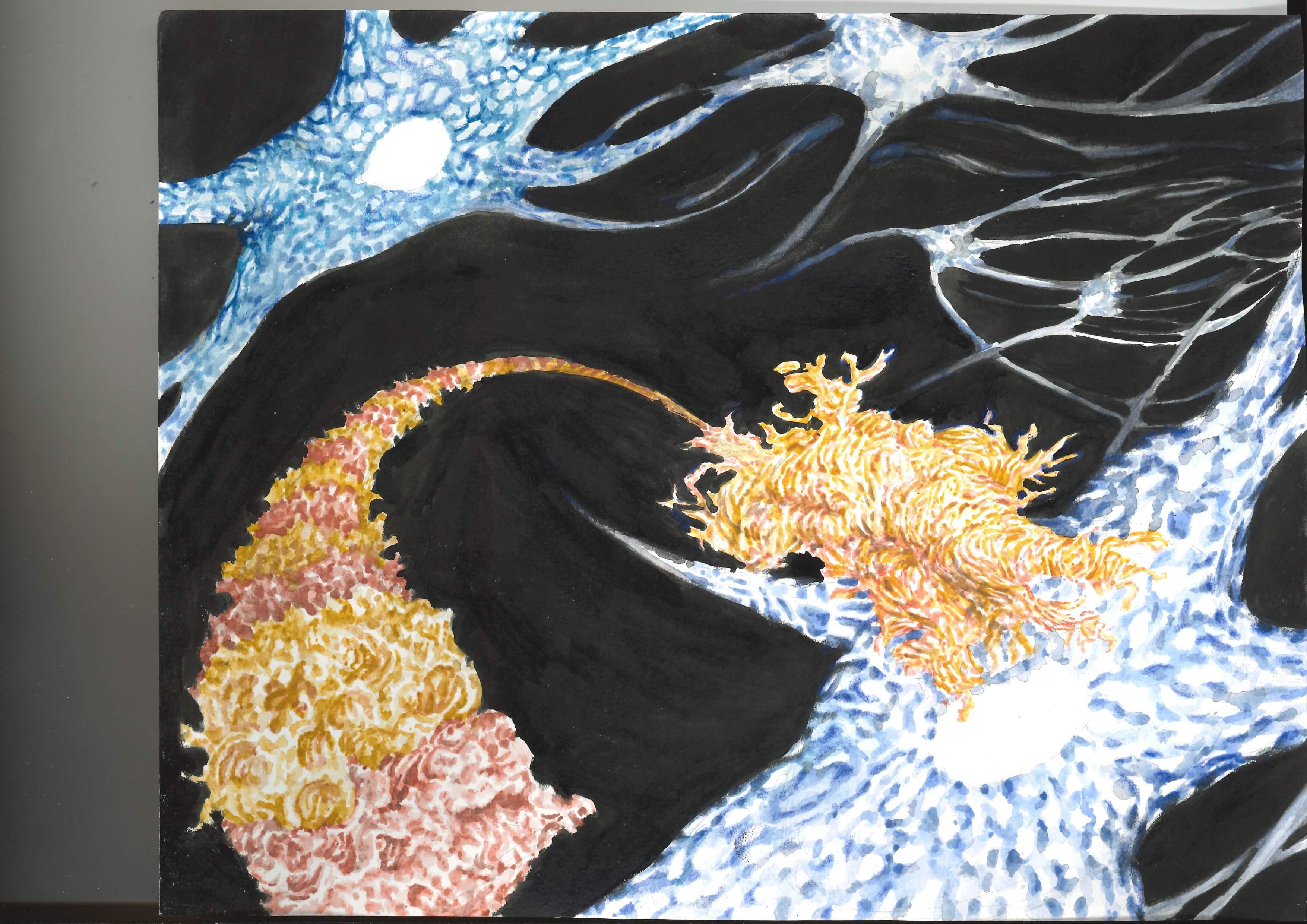
4 minute read
BIOLOGY MEETS PHYSICS
Tasmin Sarkany on how interdisciplinary scientific research might lead to major insights and discoveries about disease and the origin of life.
It can often seem that biology, chemistry and physics are growing further apart, but some of the current major scientific frontiers defy this. Lying on the boundary of theoretical physics and cell biology is the fascinating study of liquid-liquid phase separation in cells. Although the name may sound obscure, this research promises amazing applications in areas ranging from the study of Alzheimer’s and Parkinson’s disease, to insights into the origin of life.
Advertisement
It all started with the problem of how biochemical reactions in cells are spatially organised – how does a cell make sure that the chemicals needed for a reaction all congregate in the right place for it to occur? This is easy to explain when reactions occur in compartments (organelles) bound by membranes, which means that chemicals are confined to where they need to be. But it is more puzzling when we consider the many “non-membrane bound” organelles in cells. Here it turns out that the explanation can be found using theoretical physics.
Although scientific history is full of examples of physics being applied to biology (optical microscopes and X-ray diffraction techniques are applications well established in biological research), the research into phase separation in cells is exciting because looking at biological systems from a theoretical physics standpoint is still very new.
Research into phase separation
A leading research group that is looking into this is the ‘Mesoscopic Physics of Life’ group at the Max Planck Institute for Complex Systems, run by Dr Christoph Weber. The group investigates the theoretical physics of ‘liquid-liquid phase separated droplets’ inside cells. Liquid-liquid phase separation refers to two liquids of different compositions, which are separated in space due to a repulsive interaction between their molecules. Weber and colleagues use the fact that, ultimately, this relates to a very general principle in physics: systems will tend to a state in which the energy of the system is at a minimum. In thermal physics this means that two liquids can separate into a droplet and a surrounding liquid. The important point here is that there is no membrane, or solid boundary, separating these two liquids.
In 2009, Clifford Brangwynne (Princeton) showed that structures called ‘P granules’ in the nematode Caenorhabditis elegans actually displayed liquid behaviours. This meant that the physics of liquid-liquid phase separation could be applied to these ‘granules’ and other similar structures observed in cells.
There are many important outcomes that arise as a result of these droplets being liquid-liquid phase separated: since the droplet is liquid, there is fast diffusion within, meaning that chemicals in the droplet will be well mixed. Thus chemical reactions can occur in these droplets, and it may even be favourable to do so if the droplet has a chemical composition with a higher concentration of reactants (or proteins) than the surrounding liquid.
Applications to Alzheimer’s disease?
A thorough understanding of this has the potential to revolutionise multiple areas of biology. For medical science, the study of cellular compartments with high concentrations of proteins holds particular relevance for diseases such as Alzheimer’s and Parkinson’s disease. These diseases are characterised by solid or crystalline formations occurring in the cell. It has been suggested that these occur due to the formation of a liquid droplet going wrong and instead forming a solid. Therefore, gaining insights into the theoretical physics of phase transitions could tell us more about why these diseases occur.
New views on the origin of life?
Another implication of research into phase separation inside cells may be new discoveries about the origin of life. On early Earth, the atmosphere, land, and sea contained a huge variety of chemicals, including many based around carbon. These chemicals were used to synthesise biological molecules (amino acids), which are the building blocks of proteins. One major research question on the origin of life is how this dilute mixture of amino acids became concentrated enough to allow for the chemical reactions that ultimately lead to the occurrence of life. Since phase separation can spatially organise reactants to concentrate them, this research may provide answers into how life could have originated on Earth.
Recently, physical research into the dynamics of droplets has also led to discoveries on how the droplet shape can change under different conditions depending on the chemical reactions happening inside the droplet and other conditions such as pressure and temperature. A study in 2016, using the theory of these droplets, showed that they are able to divide under certain conditions. The computer modelling simulations of this process showed shapes very similar to those in cell division, so it has been postulated that the physics of these droplets may even be used as a model for protocells (cell-like objects before the cell as we know it had evolved). This would be a huge advancement in the study of the origin of life, stemming from the combination of theoretical physics and biology!
Using theoretical physics to study liquid-liquid phase separation in cells is a fascinating example of how interdisciplinary scientific research can lead to major insights and discoveries. This might just be the start of a new era of interdisciplinary studies as major frontiers in science – for when we collaborate with those in different fields, we never know what we can find.
Tasmin Sarkany is an Undergraduate in Physics at Lincoln College.
Artwork by Emma Braine