
6 minute read
Particle Piñatas
Another pharmacogenomic test is the HLA-B gene test, which also tests for variants of a gene in patients. The HLA-B gene has several variations depicted by different numbers. For example, pa‐tients with a HLA-B*1502 gene variant cannot take Carbamazepine to treat epilepsy and seizures, as they have a higher risk of developing adverse re‐actions. Patients with a HLA-B*5801 gene variant can develop a potentially life threatening dermato‐logical disorder called Toxic Epidermal Necrolysis if given a medication used to decrease high blood uric acid levels. These pharmacogenomic tests are not widely used in hospitals, mainly due to con‐cerns about costs, which can range from around £100 to £2,000. Another reason is due to the chal‐lenge of showing how these tests results can be utilised clinically to improve patient outcomes. As researchers continue to study genotypeguided drug therapies with the goal of improving clinical outcomes, some focus has been put on re‐search that can help identify novel molecular tar‐gets that drive disease progression in certain individuals. It is likely that these targets are relat‐ively uncommon, and drug development will be difficult, but the benefits will be huge. In an effort to shed more light on the future development of personalised medicine, the Centre for Personalised Medicine at University of Oxford serves as a com‐munication and engagement vehicle for students, academics, clinicians and the public to explore the benefits and challenges of personalised medicine. Although much work remains to be done in the field, personalised medicine offers an opportunity to transform the future of healthcare. “Personalised medicine and Pharmacogenomics testing offer the possibility of more precise therapeutics for several drugs and disorders.”
Sandra Adele is studying for a Masters in Pharmacology at Green Templeton College. How accelerators sweeten up the Standard Model of Particle Physics Particle Piñatas
Advertisement
"T here is nothing new to be discovered in physics now. All that remains is more precise measure‐ment”. This famous quote by Lord Kelvin in 1900 spoke of a perspective shattered almost immediately by a stream of significant discoveries, such as the discovery of the electron in 1897, the nucleus of the atom in 1911, and the proton in 1919. Suddenly, our understanding of the “building blocks” of our world had completely changed. The atom, once accepted to be absolutely indivisible, was now just a doorway into an untouched realm of discovery. Throughout the years, a new model of the fundamental particles, forces, and interactions of the universe was developed: The Standard Model of particle physics. Behind the dawn and growth of this new era was the innovation of accelerators and colliders. Accelerators focus and boost subatomic particles to higher energies and velocities using electromagnetic fields to produce well-focused and high-energy beams. In many ways, the utility of accelerators and colliders can be equated to piñatas. Only when you beat the piñata do you realise what is inside of it. Colliders operate on the same principle, with the impact being between two particle beams and the highly anticipated sweets corresponding to subatomic particles we might discover. The harder the impact, the more sweets come out. Therefore, if you handed a toddler the stick, you would get far fewer sweets than if a grown teenager were to beat the piñata open. “The atom, once accepted to be absolutely indivisible, was now just a doorway into an untouched realm of discovery”
What does all of this mean? We simply do not have ac‐cess to studying many particles until we reach high enough collisional energies. And just like the toddler needs time and funds to grow into a teenager, accelerators also need time and funds to advance and reach these higher potential collisional energies. Accelerator physicists are simply playing
How accelerators sweeten up the Standard Model of Particle Physics D espite their high collisional energies, circular colliders are not perfect. Accelerated charged particles following a bent trajectory radiate away energy (synchrotron radiation). This means for lighter particles, like electrons, acceleration using a circular collider is not very effective unless the ra‐dius is very large, adding massively to the cost of production. Circular colliders also need intricate systems of superconducting magnets to steer the beam and ensure collision. These colliders are also very expensive: the LHC cost around £3.85 billion whereas the largest linear collider, SLAC, cost £0.31 billion. Consequently, linear colliders, which accelerate in a straight line, are of significant interest even though they currently have lower energy reaches.
Certain phenomena are better studied at spe‐cific energies, which are easy to miss if we search only at higher energies. Hence, the lower-energy reaches of linear colliders do not render them ob‐solete. In fact, due to synchrotron radiation, circu‐lar colliders usually deal with larger-particle collisions (like protons as at the LHC) whereas lin‐ear colliders are able to study the same physics much more precisely due to cleaner signals and data. Designs for an International Linear Collider (ILC) and Compact Linear Collider (CLIC) have been proposed to study various elements of the Standard Model and its contemporaries, such as more detailed measurements of the Higgs boson. The FCC, ILC, CLIC are three cookies in a jar full of potential accelerator technological ad‐vances. With several new methods still in develop‐ment, the scientific renaissance of the 19th and 20th centuries that inspired our quest to discover the ‘truth’ of our reality is just the beginning. Al‐though the piñata is yet to burst, we can be certain that whatever trickles out will be remarkable. Sparshita Dey graduated from St John’s College with a Masters in Physics in 2019. “Despite the brilliance of our current Standard Model, there are still large gaps in our understanding...” the role of parents and they are taking their role very seriously.
The Large Hadron Collider (LHC) in Switzerland is the largest collider in the world: a circular accelerator with a 27km diameter. Over the years, research conducted there and at other fa‐cilities around the world have led to the develop‐ment of an incredible database of fundamental particles. A recent triumph of the LHC was the confirmed detection of the Higgs boson, the particle that explains why matter has mass and whose existence was postulated in 1964 but had never been empirically shown.
Despite the brilliance of the current Standard Model, there are still large gaps in our understand‐ing, such as the nature of dark matter and energy, accounting for a speculated 96% of the universe and also the matter antimatter asymmetry of the observable universe. Other key theoretical at‐tempts include the unification of the four funda‐mental forces of the universe: the Standard Model does not account for gravity, which has been ex‐tensively described in Einstein’s general relativity. Research into new accelerator technologies is in full swing to facilitate our search for evidence, and maybe even discover new physics.
Physicists at Oxford and several other univer‐sities are actively involved in this effort. The Fu‐ture Circular Collider (FCC) has been proposed to follow up on research conducted at the LHC, but with ten times the collisional energy. This will al‐low them to both test long-standing hypotheses and raise new questions – the true essence of bluesky research. “Behind the dawn and growth of this new era was the innovation of accelerators and colliders” Particle Piñatas
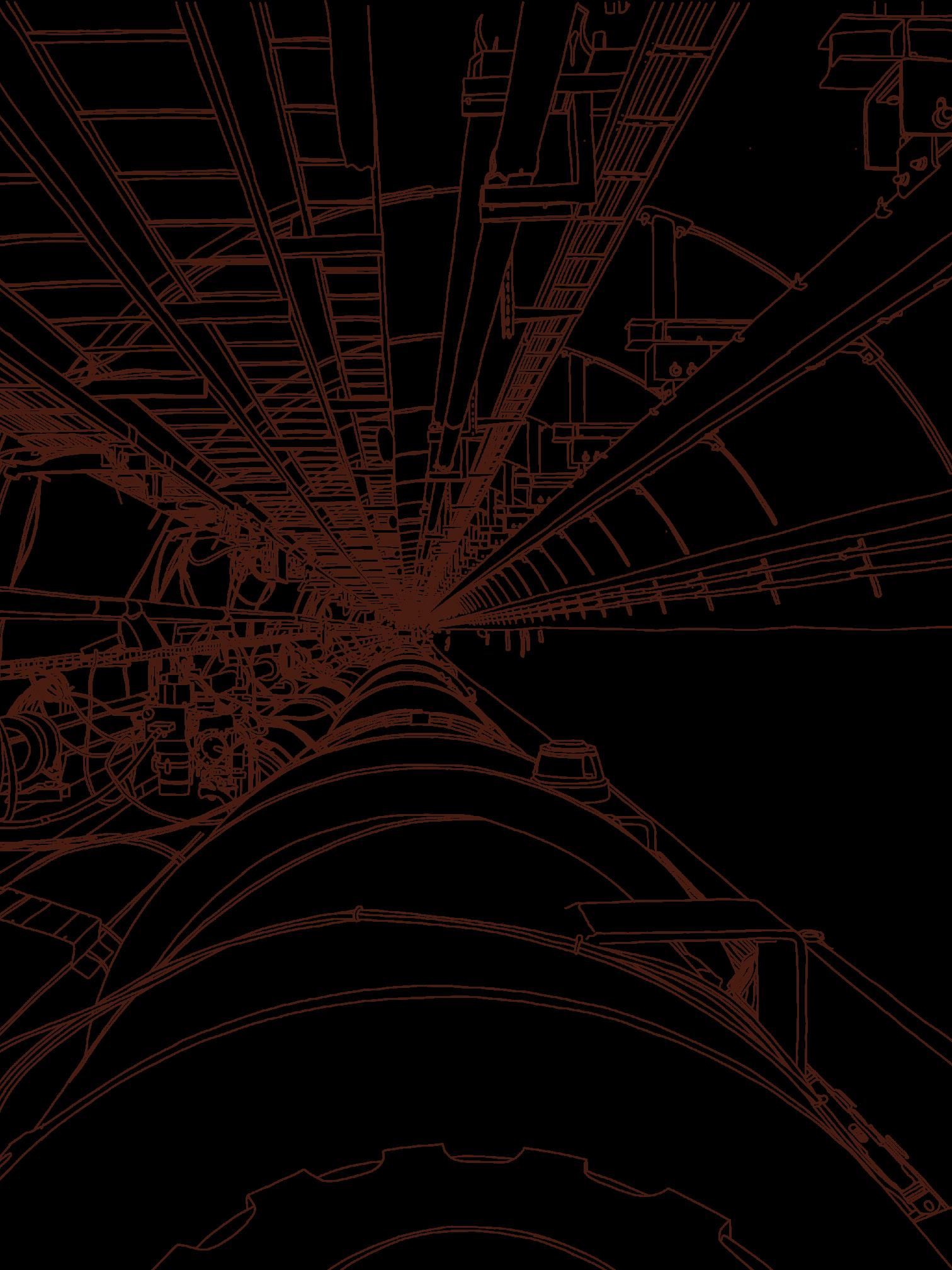
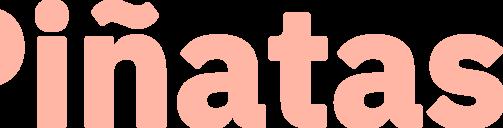