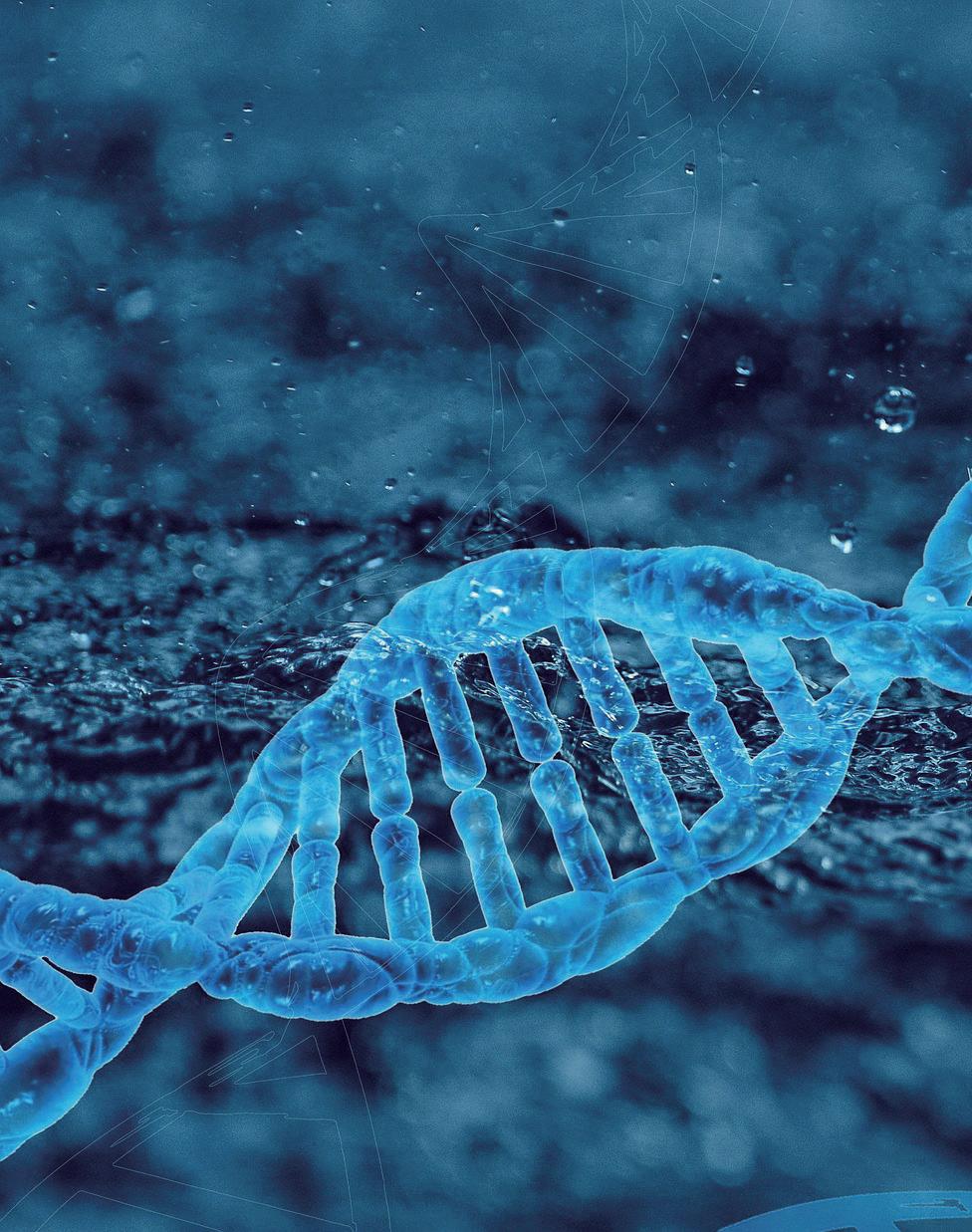
4 minute read
Synthetic Biology in Drug Development
Journalist | Jungyoon Ryu | jungyoon_r@yonsei.ac.kr
Designer | Soyun Kang | soyun525@yonsei.ac.kr
Advertisement
The past few decades were marked by blooming innovations in genetic engineering where existing genes were explored and exploited to satisfy human needs. However, we are currently facing the introduction of a new era in biotechnology, which is highlighted by an emerging field, synthetic biology. Taking a step further from simply analyzing and altering existing DNA sequences, synthetic biology aims to code and synthesize novel DNA sequences that are expected to function in a desired way. Thusly designed DNA sequences consisting of an assortment of genes and elements of choice can be applied in cells to create redesigned biological systems. Currently, researchers in the field are attempting to artificially synthesize the whole genome of unicellular organisms such as yeast and have partially succeeded. The availability to completely design from scratch and put together biological systems and modules make synthetic biology a valuable apparatus in various areas that deal with live organisms. Especially since cell and gene therapy is of growing importance in therapeutics, tools and technology of synthetic biology are foreseen to be a powerful asset in drug discovery and development.
Advantages over prior forms of genetic engineering
The major difference between ‘conventional’ (pre-‘synthetic biology’) genetic engineering and synthetic biology is that the latter bestows a greater degree of freedom on the engineer. Genetic engineering in the conventional sense concerns with inflicting slight alterations to existing DNA sequences to confer enhancements on existing traits. Synthetic biology is rather likened to putting together a set of blocks to build something new than making improvements to a preexisting entity. The builder is provided with a bundle of building blocks, i.e., useful genes and DNA parts, and can readily choose which ones to adopt when assembling the blocks into a wanted form. The concept is more engineeringwired since procedures are modularized and standardized; biological parts are treated like components of a circuit.
So to speak, synthetic biology enables the creation of programmed cells or original forms of organisms that possess precisely the traits targeted to be exhibited. This aspect of synthetic biology makes it a substantial step forward from the status quo in genetic engineering since processes to attain a common objective are shortened. For instance, biological modules can be designed to express complex end products at high rates while previous methods aimed to obtain intermediates that are preferable over natural products. Intermediate substances must be processed further to gain the desired product. Hence, synthetic biology arranges more time- and cost-effective solutions with more room for revision of traits.
Tools and methodologies of synthetic biology
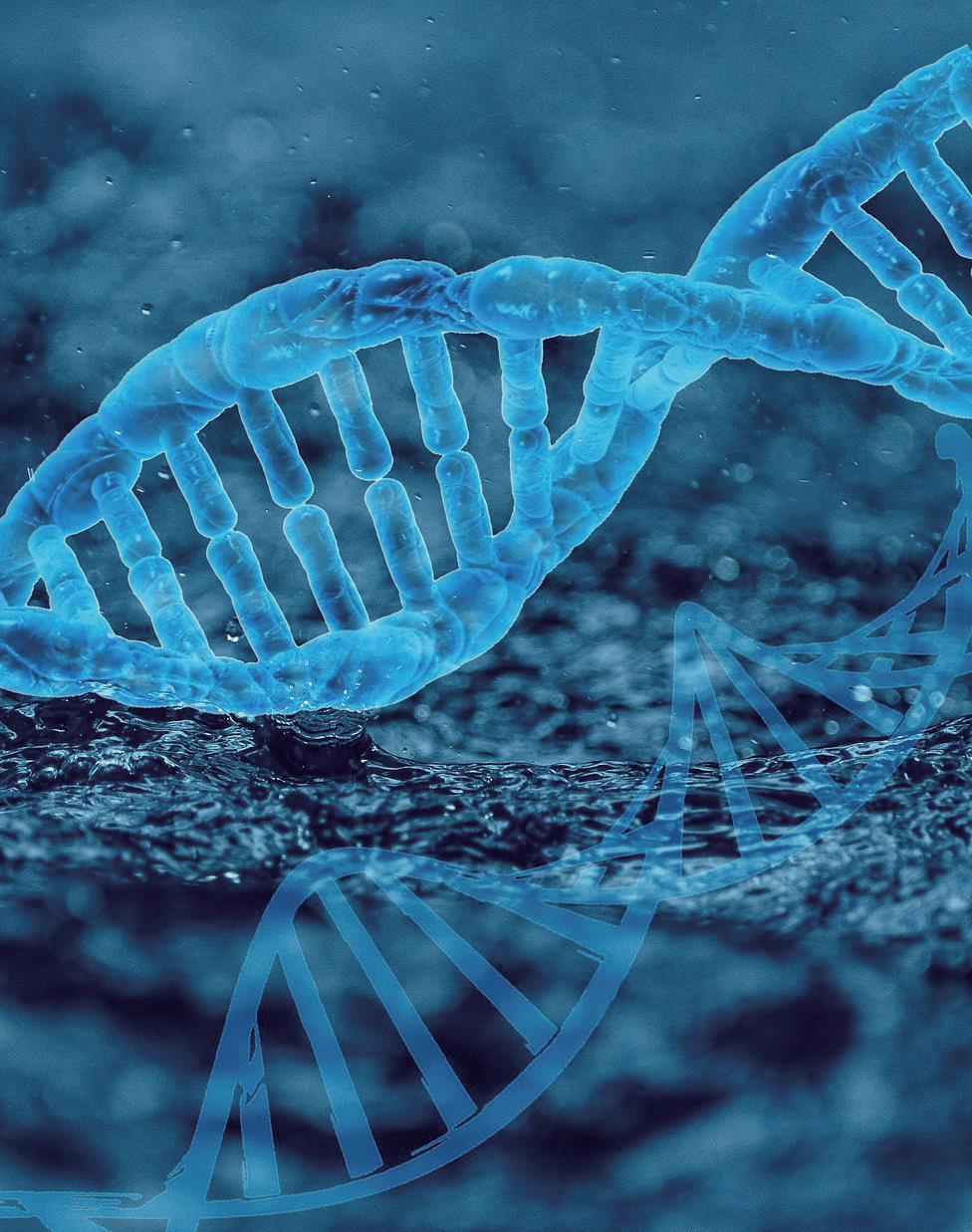
Methodologies in synthetic biology employs the technical tools established in genetic engineering, while being organized in a cycle of four essential parts: design, build, test, and learn. First, a biological system of a predictable function is designed based on the idea that biological functions can be modeled via assembly of standardized biological parts. A chassis, a physical platform (or host cell) on which designed DNA can be applied, is selected. Biological components deconstructed into bioparts are chosen and aligned in a way that is expected to produce a specific function when applied on the selected chassis. Bioparts applicable in the designs include enzymes, biosensors, protein or nucleic acid scaffolds, and other regulatory elements. Then, based on the design, DNA is engineered and assembled into bioparts or novel genomes through various strategies that exploit recombinant DNA technology or DNA synthesis techniques. The next stage includes prototyping and testing of the biological system. The cycle culminates in the ‘learn’ stage, where data acquired from the ‘test’ stage is analyzed by machine learning and statistical methods for optimization of development pathways and for information useful in the next round of design.
Applications in drug discovery and development
Since synthetic biology allows the creation of a biological system or module directly aimed to serve the engineer’s targeted purpose, it is open to application in countless stages in drug discovery and development. Synthetic biology systems can act as new tools for studying and uncovering the molecular mechanisms involved in disease development, as well as for discovery and production of testable molecules. Drugs can be developed from the products of synthetic biology which range from chemicals to engineered cells themselves. Chemicals derived from engineered cells can be of greater complexity from the ones that could be produced through genetic engineering methods since genetic circuits consisting of multiple enzymatic reactions can be designed. Hence, such methods can cut time and cost required for production. When the engineered cells themselves are the aimed product, they can be used as pharmaceuticals in cell therapy and personalized medicine. The next generation of CAR-T cell therapy designed through genetic circuits will be able to overcome issues of the previous generation including resistance and off-target toxicity. Therapeutic designer cells which are whole cells redesigned by putting together bioparts will be able to attend to more specific needs of individual patients. Even more, the integration of synthetic biology with automation technology leads to the arrangement of highthroughput procedures for creating new biological systems, which will enable faster screening and analyses for suitable bioparts.
Restraints and opportunities
As many possibilities synthetic biology confers to the productive mind, it can give way to possibilities of adverse usage of the technology. For instance, it can become an easily accessible and cost-effective means to invent and manufacture biological weapons such as virulent strains of genetically modified viruses. Moreover, since methods in synthetic biology inevitably entail alteration of genetic sequences, it is important to contemplate the repercussions of tampering with natural genetic combinations. Most importantly, synthetic biology is not free of bioethical implications that are concerned with human embryo and germ-line genetic modification. Nevertheless, it is undoubtedly a promising field in the sense that it presents a breakthrough for treatment of formerly incurable diseases.
To date, the main object of the synthetic biology department of drug development was to create synthetic bioparts that can generate complex molecules and chemicals essential in the synthesis of useful drugs. However, it is anticipated that attempts in the field to build an entire cellular system through means of synthetic biology will enable the assembly of therapeutic designer cells that are capable of exhibiting a long-lived and target-specific therapeutic action while residing as a part of the patient’s bodily system. B