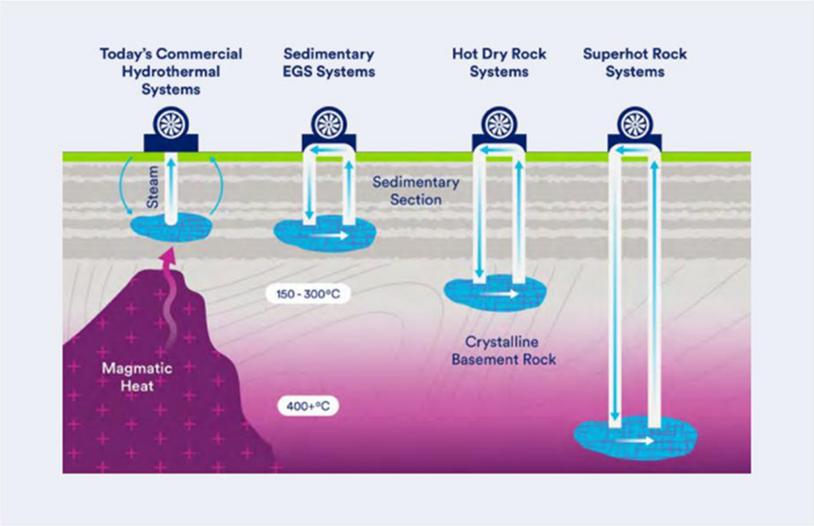
20 minute read
Enhanced Geothermal - GEOTHERMAL ENERGY SERIES Part 4 CHOA eJournal 2022 12 08
from December 2022 CHOA Journal
by CHOA
GEOTHERMAL ENERGY SERIES
O&G Expertise Unlocking the Earth’s Energy Potential: Part 4
Advertisement
BY TIM MONACHELLO AND PATRICK TANG, ATB CAPITAL MARKETS
TURNING UP THE HEAT: ENHANCED GEOTHERMAL
What deep drilling means for unlocking geothermal energy in new areas.
INNOVATION TO BRING GEOTHERMAL MAINSTREAM
In areas where high permeability, high temperature geothermal resources are not readily available (such as Alberta), enhanced geothermal systems (EGS) have been, or are being, developed that can be used to enhance the efficiency and/or economics of a geothermal project.
While the term EGS has sometimes been used to refer to just fractured geothermal systems and AGS (advanced geothermal systems) has been used to define other next generation systems, for our purposes, we use the term “EGS” to refer to all next-generation geothermal systems, which encompasses both new geothermal downhole technologies that can improve the power generation capabilities of lower temperature resources outside of known hydrothermal plays, new configurations that allow for deeper commercial drilling, and technologies that utilize geothermal resources devoid of an aquifer.
While most EGS applications are in relatively early stages of development and commercialization, we believe the wide scale adoption of geothermal energy is dependent on EGS technologies driving the economic viability of projects in areas previously unconducive to geothermal resource development.
ENHANCED GEOTHERMAL SYSTEMS
I. Deep Geothermal Resource Development:
Commercial geothermal power development generally requires a geothermal resource at roughly 150°C, and higher temperature resources will yield better economics. Perhaps the largest single headwind facing geothermal development has been that hydrothermal resources are generally too cold for power generation at commercially accessible depths.
Nevertheless, higher geothermal temperatures can be found around the globe at deeper intervals. While the geothermal gradient is not consistent around the globe, the temperature of the Earth’s crust increases around 2°-3°C/100m in depth on average, though this can range from 1°-5°C/100 m.
At roughly 10km depth, 150°C geothermal resource is nearly ubiquitous across the continental United States, while at 3 km depth, most resource is in the 75°-100°C range except for certain high-temperature geothermal sites that are the primary focus of current conventional developments.
That said, leading edge drilling technologies in the oil and gas sector have shown the ability to drill wells over 7-10 km (total measured depth), though these wells are typically only a few kilometers deep and sometimes over 7 km in lateral length, and well diameters are significantly narrower than for commercial geothermal development.
With Technological Advances, Deep Drilling Could Unlock Geothermal Around the Globe
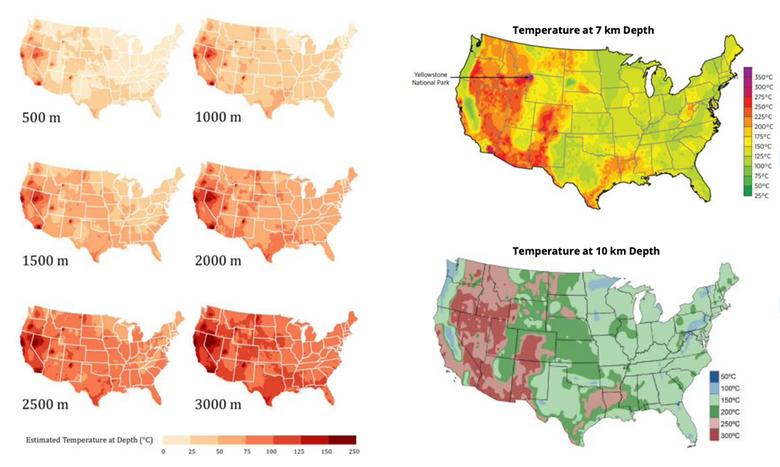
Figure 19 – With Technological Advances, Deep Drilling Could Unlock Geothermal Around the Globe
Source: National Renewable Energy Laboratory, Department of Energy, University of Michigan, ATB Capital Markets Inc.
THE DEEPER THE HOTTER: While the geothermal gradient is not consistent across the globe, on average the temperature of the Earth’s crust increases around 2°-3°C/100m in depth, though this can range from 1°-5°C/100m.
GEOTHERMAL COULD BE ACCESSIBLE EVERYWHERE WITH DEEP DRILLING: At roughly 10 km depth, nearly all of the continental US has geothermal temperatures at or above 150°C, which is hot enough for power generation.
TECHNOLOGICAL DEVELOPMENT IS REQUIRED: Current technology can drill wells to total measured depths in the 7-10 km range, though these are generally significantly narrower boreholes than are required in geothermal drilling, and measured depths highly weighted to the lateral section.
Superhot Rock Geothermal Could Be the Holy Grail
At depths of 10-20 km, high-temperature geothermal resource can be found nearly everywhere on Earth. This resource, known as superhot rock (SHR) geothermal, has been dubbed “the holy grail” of geothermal, as it has the potential to unlock access to commercial temperature resource regardless of location.
For context, a report by the Clean Air Task Force (CATF) suggests that SHR resources could deliver mature LCOEs in the US$0.020-$0.035/kWh range (US$20-$35/MWh) which it views as competitive with other dispatchable and intermittent energy sources. For context, the EIA estimates the LCOE for geothermal plants entering service in 2027 to be $39.82/MWh, and this is effectively based on incremental developments in currently feasible areas only.
Superhot Rock Systems Could be a Game Changer, but Require Innovation
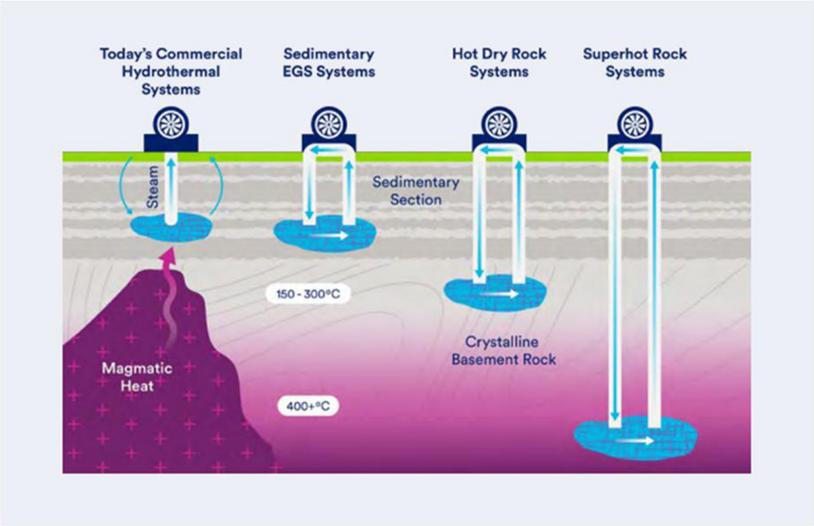
Figure 20 – Superhot Rock Systems Could be a Game Changer, but Require Innovation
Source: Clean Air Task Force, ATB Capital Markets Inc.
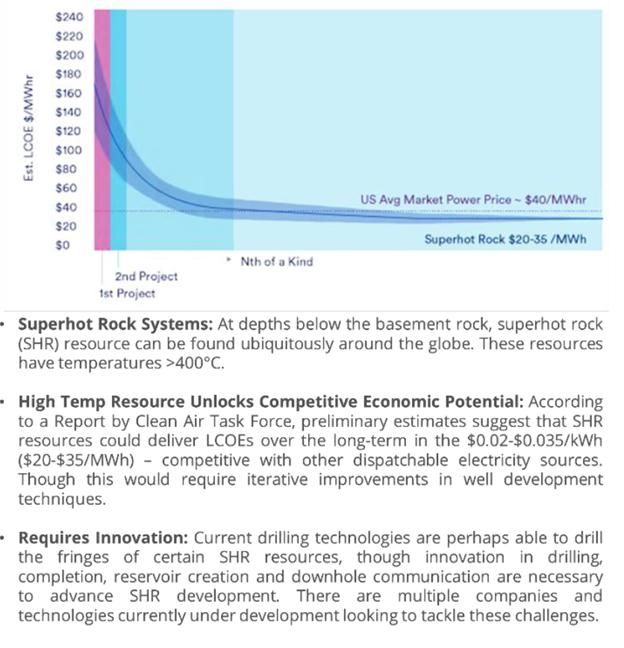
While drilling SHR systems appears attractive, there are at present certain challenges that require innovation to overcome. Primary challenges include:
CURRENT WELL DEVELOPMENT TECHNOLOGY NOT SUITED FOR SHR DEPTH AND TEMPERATURE: Depth limitations on current mechanical drilling techniques have not generally been tested, as most hydrocarbon wells are shallower than SHR depths, and rigs are generally designed to drill significantly farther both horizontally and vertically.
In addition, SHR reservoirs are located within crystalline basement rock, which is generally harder than sedimentary rock deposits closer to the surface. Ultra hard rock drilling can increase the cost of mechanical drilling techniques as equipment wear and time to drill increases in harder formations.
Moreover, mechanical drilling technologies and well construction materials are generally designed for temperatures in the 150°-300°C range and not for >400°C. At these elevated temperatures, the physical properties of metal parts, drilling fluids, well casing and cement can be altered.
BASEMENT ROCK GENERALLY REQUIRES RESERVOIR CREATION: Aquifers are not generally present within basement rock formations, and they would require the artificial creation of fresh water reservoirs by fracturing rock while limiting seismic risk and limiting or eliminating the use of fracturing chemicals.
Drilling Innovations Could Unlock Ultra-Deep Wells
Energy drilling is a new frontier of drilling technology that looks to replaces or supplement mechanical drilling techniques with new technology using a form of focused energy to soften, melt, or vaporize rock. Millimeter wave drilling, plasma drilling and hybrid mechanical laser drilling technologies are being developed that could significantly increase the depth capacity of commercial drilling operations and potentially reduce cost by up to 90% vs. current mechanical drilling technology. These technologies are generally in their infancy and are likely at least 5-10 years away from commercialization, in our view.
Quaise – Millimeter Wave Drilling:
Quaise is developing a technology that harnesses fusion research out of MIT to deploy millimeter wave technology using a gyrotron at surface that would essentially vaporize hard rock formations. The technology has been shown to work in small-scale demonstrations, and a 3,300 ft demonstration well is being planned with partner AltaRock. Quaise plans to use mechanical drilling technologies to drill to basement rock, then switch to millimeter wave drilling to drill to depths up to roughly 20 km.
We understand this technology could offer additional benefits: 1) not requiring drilling fluids given that the pressure generated from the vaporization of rock would ensure overpressure drilling; and 2) the heat created from the formation vaporization could eliminate the need for wellbore casing, as the heat essentially creates a glass liner on the surface surrounding the well bore (known as vitrification).
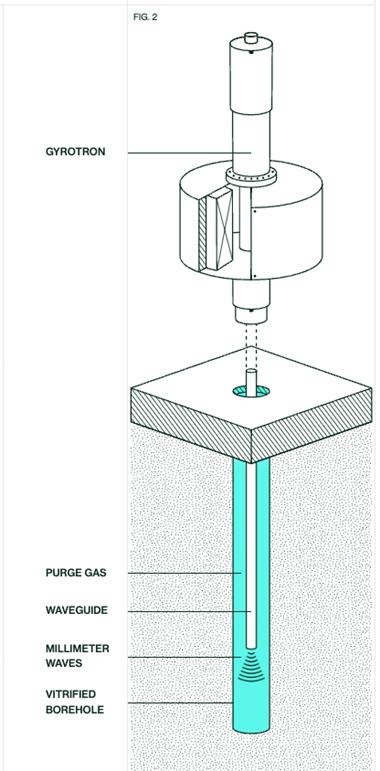
Figure 21. Quaise Assembly Diagram
Source: Quaise Energy
If successful, Quaise estimates that its technology could be up to 90% cheaper than conventional deep well drilling technologies. We note that, in August of 2021, Nabors Industries made a US$12 mn investment in Quaise, with Nabors’ Chairman, President and CEO stating “We see in Quaise one of the most potentially disruptive technologies in the space, and we believe the team is well positioned to capture the ultimate potential of this technology.”
GA Drilling – Plasma Drilling:
GA Drilling, based in Slovakia, is developing PlasmaBit® technology, which essentially uses a pulsed plasma technology to create short, high-energy pulses at high frequency causing acute increases in rock temperature that disintegrate the rock’s surface. GA Drilling also contemplates using mechanical drilling technologies to reach basement rock and then deploying PlasmaBit® technology using a coiled tubing unit to reach depths of 10 km or more. We understand that GA Drilling’s PlasmaBit® has yet to be proven in the field, though it has undergone extensive lab testing. We note that, in March of 2022, Nabors Industries made a US$8 mn investment in GA Drilling. Nabors noted that it planned to leverage GA Drilling’s Centre of Excellence and to integrate GA Drilling’s technology into its ecosystem and further remarked that “Nabors intends to become a key player in the upcoming expansion of geothermal energy.”
Foro Energy – Laser-Assisted Drill Bits:
Foro Energy is developing a laser-assisted drill bit that uses advanced fiber optics to transmit high-powered lasers for use in deep drilling operations. The lasers are paired with mechanical drill bits and are used to essentially soften the formation for mechanical drilling. Foro Energy states that its laser-assisted drill bits could be 10 times more economical than conventional drilling technology in hard rock formations. We also note that Fraunhofer IPT, along with “LaserJetDrilling” project partners, is developing another system that would combine lasers with mechanical drill bits for use in hard rock formations.
II) Horizontal and Fractured Well Designs:
Horizontal well designs are being used to increase the productivity of producing geothermal wells by increasing the area that wells collect water within the high-temperature producing formation, and some projects have also enhanced flow rates in lower permeability formations by fracturing the reservoir. In addition to improved production rates per well, pad well designs can increase cost efficiencies, as multiple wells can be drilled from a single surface location. There are numerous companies developing technologies to optimize fractured well designs for geothermal leaders include Fervo Energy and Sage Geosystems. DEEP Earth Energy Production Corp. is an example of a company that plans to utilize horizontal drilling for geothermal power development – the initial phase of its project is planned with 34 horizontal well bores (18 producing and 16 injecting), each with a vertical depth of roughly 3,500 m and a horizontal reach of 3,000-4,000 m.
III) Closed Loop Geothermal Systems:
In our view, closed loop geothermal systems are the furthest departure from conventional geothermal systems, as they do not use reservoir water directly. Instead, closed loop systems are designed to use a thermosiphon to circulate water through a closed loop without the need for pumps. The closed loop design uses heat to power generators to produce electrical power. Closed loop designs rely on the advanced horizontal drilling capabilities developed in the oil and gas industry to drill multiple horizontal wells that are connected within the reservoir to create a loop.
Closed loop designs are in the early days of development, but they offer significant potential advantages over conventional designs: 1) they do not rely on well permeability or natural flow rates, which can increase the applicability to less favourable resource environments; 2) they reduce the impact of produced brine on equipment (including scale, corrosion, etc.); 3) they do not require pumps, which significantly reduces or eliminates the parasitic load on the system; and 4) they could offer greater dispatchability compared to conventional geothermal power plants. That said, the closed loop design does not allow for the extraction of hydrocarbons or other minerals from the formation. Closed loop systems are being advanced by companies in both Canada and the US, of which we highlight Canada-based Eavor Technologies (see below) and US-based GreenFire Energy.
CASE STUDY: Eavor Loop - Using Innovation to Solve Conventional Issues
Founded in 2017 in Calgary, Alberta, Eavor Technologies is a pioneer of conduction based closed loop geothermal systems, and it is developing technologies that we believe could be transformative for geothermal energy developments. We understand Eavor has developed and patented many innovations that could enable the drilling of hermetically sealed closed loop geothermal systems, which do not rely on aquifers or require pumps, use a thermosiphon to create flow, and could potentially be drilled almost anywhere on Earth.
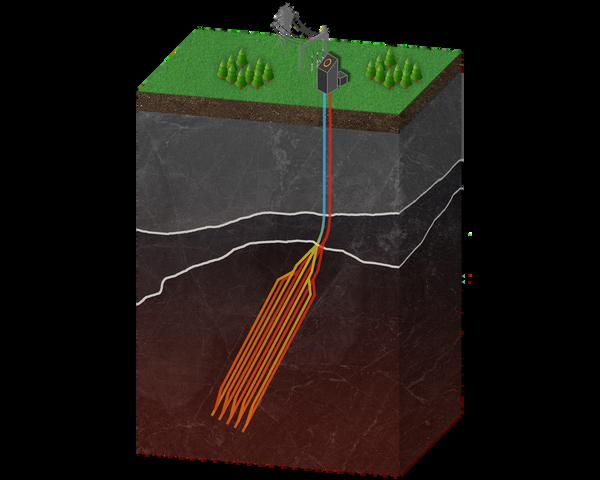
Eavor also claims a multitude of other advantages of its design. In future designs (Eavor-Loop 1.0 and Eavor-Loop 2.0; see Figure 22), Loops will be placeable in various 3D planes from a single surface location, increasing heat extraction from the resource and consolidating wellbores into a single facility. As such, this would allow geothermal to be economically developed from areas not previously thought to be feasible using conventional or other enhanced geothermal systems Furthermore, the thermosiphon can be started and stopped without any input power, meaning that the geothermal power plants can be used to efficiently meet power demands (“load following”). When flows are halted, the working fluid can absorb more geothermal heat and, upon restart, the higher temperature working fluid can push power generation rates above normal operating capacity for a period of time up to three times as much making Eavor’s technology suitable for both baseload power and peaking power plants. This feature, called dispatchability, allows for Eavor-Loops to work symbiotically with intermittent power such as wind and solar.
Eavor Capital Costs and LCOE
Management claims that the power generation of Eavor-Loops is both highly reliable, predictable, and each Loop can last for roughly 100 years. The output of each Loop is simply a function of the well design and the thermal resource, which can vary meaningfully from project to project and can impact returns.
CAPITAL COST: While project costs are subject to significant variability; we understand that Eavor Lite costs roughly $25 mn, and Eavor’s Germany project (four Eavor-Loop 1.0s) is slated to cost roughly $220 mn. Beyond this, Eavor’s management has estimated that it would cost roughly $100 bn to develop 2,500 Eavor-Loops through 2030 Based on this, we estimate that each Eavor Loop could cost roughly $30 mn-$60 mn to develop. At roughly $10 mn/MW, the capital cost of Eavor Loops is significantly higher than most renewable projects, but Eavor-Loops benefit from low operating costs, high utilization, and significantly longer useful lives than solar or wind projects, with a 100-year expected life.
LCOE: Currently, we understand Eavor’s LCOE is well above economic levels - likely in the $250/MWh range That said, Eavor is targeting a $50/MWh LCOE, driven by efficiency gains from drilling techniques, project design, and other optimizations as the Company improves its commercial deployment. Specifically, Eavor believes that if its well test in New Mexico goes as planned, it could achieve $50/MWh reliably in the Southwest US where the geothermal heat gradient is ~600C/km) – over the next 3-4 years At the $50/MWh target LCOE, Eavor Loops could be competitive with other renewable forms of power generation but could offer significant advantages over wind and solar including 1) being a source of baseload power and not having intermittency issues and 2) the ability be deployed in regions where wind and solar resource are sub-economic or where land costs are prohibitively expensive.
The Path to Commercial Eavor-Loops
Eavor Technological Development – From Pilot to Scalable Commercial Application
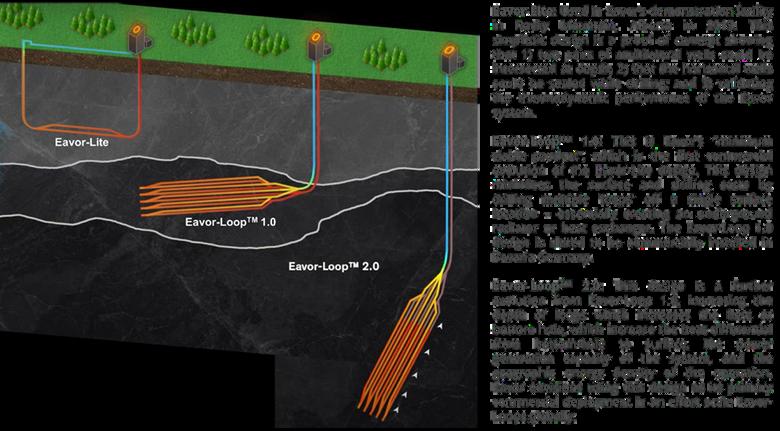
Figure 22 – Eavor Technological Development – From Pilot to Scalable Commercial Application
Source: Eavor Technologies Inc , ATB Capital Markets Inc
Eavor-Lite: Used in Eavorès demonstration facility in Rocky Mountain, Alberta in 2019. This simplistic design is a proof of concept showing that 1) two pairs of multiateral wells could be intersected at depth; 2) that the horizontal wells could be sealed while drilling; and 3) validating the thermodynamic performance of the Eavor system.
Eavor-Loop™ 1.0: This is Eavor's "minimum viable product", which is the first commercial evolution of the Eavor-Lite design. This design minimizes the surface and drilling costs by drilling multiple 'loops' off a single surface location - essentially creating an underground radiator or heat exchanger. The Eavor-Loop 1.0 design is slated to be commercially installed in Bavaria Germany.
Eavor-Loop™ 2.0: This design is a further evolution from Eavor-Loop 1.0, increasing the depth of loops which increases the heat at bottom hole, which increases the heat differential from bottom-hole to surface, the power generation capacity of the system, and the geographic energy density of the operation. Eavor envisions using this design as its primary commercial deployment in an effort scale Eavor-Loops globally.
Phase 1: Eavor-Lite Demonstration Facility – Complete
Eavor’s pilot project, Eavor-Lite, demonstrated the Eavor-Loop technology near Rocky Mountain House, Alberta. The Eavor Lite facility produces roughly 1 MW thermal and can be fitted with an ORC to generate power; we understand that an ORC would be roughly 10% efficient at current formation temperature.
Precision Drilling was selected as the drilling contractor for this project, and two of the Company’s rigs broke ground in 2019. Spud to rig release was 46 days. In doing this pilot, Eavor achieved certain goals. From an overall perspective, demonstration of its technology in a less geothermally-active area (750℃, low relative to more common formations in Iceland or the US) showed prospective customers the applicability of its closed loop geothermal design in a more common resource environment.
Eavor’s pilot also served to prove that the Company could:
1. Drill and Connect Laterals: Critics of Eavor’s design noted the technical difficulty of drilling and connecting two laterals to form a loop. However, Eavor leveraged technology from the oil sands geomagnetic positioning, in particular – to accurately position wellbores well beneath the Earth’s surface.
2. Maintain Wellbore Integrity: Given the vertical depth and lateral length of the lateral and the predominantly sandstone composition of the targeted formation there was some belief that casing/cementing the wellbore (specifically the lateral section) would be a challenge. As we understand, however, Eavor’s reliance on a “rock pipe” design does not require difficult casing/cementing jobs The positive pressure of the wellbore prevents the wellbore from collapsing, and the working fluid used by Eavor is specially formulated to prevent fluid migration to the formation.
3. Initiate a Thermosiphon: While proved in concept, a thermosiphon of this scale had not been commonly seen. Eavor Lite showcased how the closed loop thermosiphon would work without parasitic load.
Phase 2: Southern US Test Well Could Prove Deep-Drilling at High Temperatures – Planning
Eavor has plans to drill a test well in the southern US in 2022, which is planned to be a long-reach geothermal well with a multi-kilometer portion of the well drilled through hard rock below the sedimentary rock layer. This well, which we understand is expected to cost roughly $10-$15 mn to drill, has potential to be among the deepest commercial-grade wells drilled, and it will target downhole temperatures exceeding 3,000℃.
This project intends to prove:
1. Efficient Hard-Rock Drilling Capabilities:
While Eavor has not disclosed details, we understand it has developed a drilling technique that it believes can rapidly drill through high temperature, hard rock formations at economical rates of penetration (ROP). In our view, success here would be not only a major breakthrough for Eavor, but also a major breakthrough for the geothermal industry, as it would mark a significant step forward in the development of deep geothermal and SHR geothermal systems.
2. Use of Guidance Systems in High-Temperature Reservoirs:
Eavor’s planned test well will include a small offshoot well that Eavor will use to test that its guidance systems will work both at extreme depths and at temperatures in excess of 3,000℃. We understand that Eavor’s novel drilling technique is designed to protect its guidance systems from extreme heat. This test is designed to show that multiple legs can be successfully connected in deep, high temperature reservoirs. If the Phase 1 test well at this site successfully proves Eavor’s ability to drill and connect wells at extreme depths and temperatures, it would also set the stage for Eavor to complete a full scale Eavor Loop 2.0 at the site. It would also be a significant step forward in Eavor’s drive to improve economics management believes a successful test would validate its line of sight to $50/MWh LCOE in high temperatures resources in the Western US.
Phase 3: Eavor-Loop 1.0 The First Commercial Application in Germany – Planning
In May 2020, Eavor announced that it had entered into a letter of intent with Enex Power Germany to jointly develop an Eavor Loop 1.0 (shallow configuration) at Geretsried in Bavaria, Germany Enex was granted a geothermal concession/lease in 2004 and drilled two exploratory wells, with the most recent well drilled in 2017. Neither of the two wells was capable of producing fluid hot enough for conventional geothermal to be feasible However, the formation temperature and geothermal gradient (roughly 300℃) was suitable for the development of an Eavor Loop that would be capable of generating power and providing heat for district heating applications.
At the latest update, surface construction is slated to begin in October 2022, and it will be funded by Eavor. Eavor expects to use grant funding and inflows from strategic investments for downhole construction, scheduled to be completed in 2025, though we understand that full funding is not yet secured.
Commercially, the project will be eligible for payments under the German Government’s Renewable Energy Sources Act, which augments the electricity price received for 20 years from the date of project commissioning and will result in a fixed power price of €252/MWh (roughly C$400/MWh). Under Germany’s Energiewende (“energy transition”) policy, which aims to develop low carbon, environmentally friendly, and affordable power sources, Eavor’s technology was selected – indicating the strategic fit of geothermal developments with German environmental and energy policy.
Phase 1, with a cumulative 10 MW design, includes four Eavor-Loop 1.0s with a $220 mn budget (see Figure 21). We believe the well costs of the project are likely at least double the cost to drill in Alberta given the lack of oil and gas services, and we believe the costs of this project are likely to be elevated given it would be the first Eavor Loop 1.0 drilled. Similar to Eavor Lite, the location was strategically chosen to demonstrate the applicability of the technology in a lower temperature resource. We believe the project economics will be weak, likely even delivering a negative return profile. That said, the site is largely a demonstration of the technology at scale, and future technology and process innovations are expected to meaningfully improve economics on future projects.
Long-Term: A Goal to Deliver Power and/or Heat to 10 Million Homes over the Next Decade
We believe the appetite for geothermal today is high: we understand Eavor alone currently has hundreds of prospective projects worth over $25 bn in capital cost, which would add reliable baseload power and help contribute to power producers’ desire to achieve energy independence.
Eavor has set a goal to build enough capacity to deliver heat and/or power to roughly 10 million homes (we estimate roughly 10,000 MW of capacity) over the next decade. To achieve this goal, management estimates roughly 2,500 Eavor Loops (implying roughly 4MW/loop) will need to be built, requiring roughly $100 bn in investment (roughly $40 mn/loop, or roughly $10 mn/MW of capacity). We understand Eavor currently has hundreds of prospective projects worth over $25 bn in capital cost, including equity and/or licensing agreements with third parties, shovel ready projects, and royalty projects. We believe success and learnings in Eavor’s ongoing Germany-based Eavor-Loop could provide a catalyst to accelerate additional international project starts.
CHOA eJournal - 08 December 2022
The next articles in this series will drill down further Opportunities for the Oil and Gas Service Sector, Economic and Strategic Factors, and the Regional Landscape for Geothermal Development. Watch for your upcoming weekly issues of the CHOA eJournal, most Thursdays!
Appendix B: List of Geothermal Developers Geothermal Developers and Major Proposed Projects

Figure 41 Geothermal Developers and Major Proposed Projects
Source: Company Reports, ATB Capital Markets Inc
Appendix C: List of Geothermal Technology Companies Geothermal Technologies and Strategic Investors
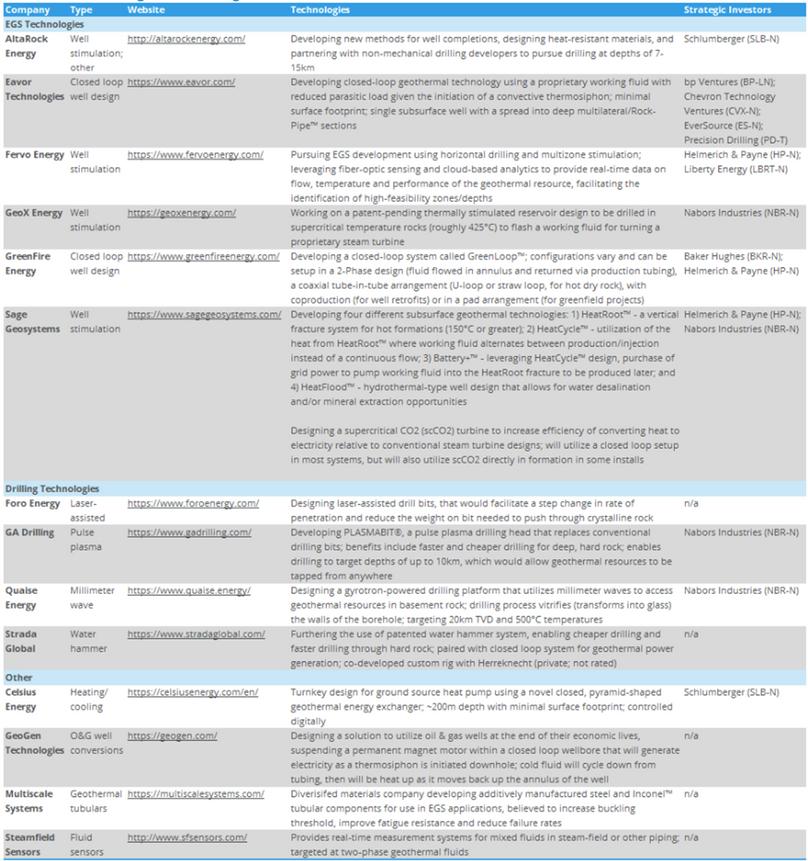
Figure 42 Geothermal Technologies and Strategic Investors
Source: Company Reports, ATB Capital Markets Inc.