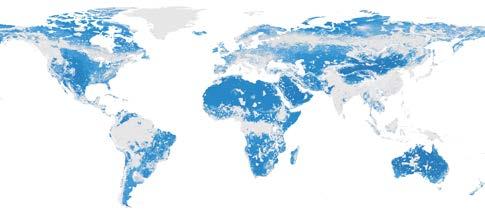
14 minute read
5.3 Special focus: International trade of hydrogen and derivatives
Green hydrogen production can provide flexibility to the power sector through price-responsive electrolysers and the storage of hydrogen to follow the seasonality of renewable resources.
To balance renewables internationally, in addition to cross-border electricity exchange, international trade of hydrogen can provide an opportunity to link low-cost renewable resources with demand centres, increasing the distance renewable energy can be transported compared with electricity. Although such trade is possible, hydrogen markets are expected to be regional to a large extent and much smaller than today’s oil and gas sectors.
Advertisement
Hydrogen trade can offer multiple opportunities for some countries:
• It can turn countries that have vast good-quality resources and import energy today into future exporters.
• It can provide diversification and sustainable alternatives to oil and gas.
• It can lead to a technology race in electrolysis and fuel cells.
• It can improve the energy security of importers by providing an alternative carrier with access to a more diversified mix of suppliers.
These prospects are leading to new hydrogen diplomacy, especially by countries that have limited renewable potential, such as Germany and Japan (IRENA, 2022f).
Global hydrogen demand could increase by a factor of almost seven by 2050, to reach 74 EJ in IRENA’s 1.5°C Scenario – a large increase but still just a fraction of global energy consumption (Figure 5.10). Reaching that figure will require an accelerated pace of renewable capacity deployment, although some fossil fuel-based hydrogen could be substituted for renewablesbased hydrogen. Two-thirds of the hydrogen supply is expected to be from renewable hydrogen and one-third from fossil fuel-based carbon capture and storage.
In 2050, about a quarter of all the hydrogen produced could be globally traded, with about equal shares transported by pipelines and ammonia ships (Figure 5.11). Pure hydrogen is expected to be transported mainly using existing natural gas networks that are repurposed to carry hydrogen. They represent the cheapest option for transporting pure hydrogen, at costs of USD 0.08–0.12/kg per 1 000 kilometres (km) in 2050. Europe has an integrated network and heavy industrial activity; some countries have limited production potential and high renewable costs. Latin America is less integrated than Europe and has larger distances to cover, but it also has large regional cost differences, as a result of differences in the cost of capital.
The costs of transporting hydrogen through new pipelines would be twice as high, at USD 0.16–0.24/kg for every 1 000 km, but it would still be lower than shipping options for distances of 3 000–5 000 km, according to (IRENA, 2022h) analysis.
FIGURE 5.10 Final energy demand in 2050 and share of green hydrogen trade
2050 - Where we need to be (1.5-S)
348 EJ Total Final Energy Consumption
Renewable share in district heat: 90%
Renewable share in hydrogen: 66%
Coal 2%
Natural gas 4%
18%
Modern biomass
Electricity (Nuclear) 5% District heat
4% other renewables
12%
Hydrogen (direct use and e-fuels)* Share of hydrogen traded internationally: 25%
Electricity (Natural gas)
Oil 4%
51%
Electricity (direct)
90%
Renewable share in electricity
FIGURE 5.11 Global hydrogen trade map in 2050 under optimistic technology assumptions
United States
Latin America
1094PJ Europe 136PJ
4771
PJ 2 382 1606
PJ PJ
North Africa Middle East China Republic of Korea
India
South East Asia Japan
South Africa Australia
NH3 Flow (PJ) 0 – 100 101 – 300 301 – 800 H2 Flow (PJ) 0 – 100 101 – 600 601 – 900 LH2 Flow (PJ) 0 – 100 101 – 600 601 – 900
Exporter Importer/ Exporter Importer PJ H2 Flow within region LOHC Flow (PJ) 38
PJ
NH3 Flow within region
Note: Figure is based on the following optimistic assumptions for capital expenditures. PV: USD 225–455/kilowatt (kW); onshore wind: USD 700–1 070/kW; offshore wind: USD 1 275–1 745/kW; electrolyser: USD 130/kW. The weighted average cost of capital is per 2020 values without technology risks across regions. Green hydrogen production is based on assessing land availability for solar PV and wind. Demand is in line with a 1.5°C Scenario. For more details, see (IRENA, forthcoming-a). Disclaimer: This map is provided for illustration purposes only. Boundaries and names shown on this map do not imply the expression of any opinion on the part of IRENA concerning the status of any region, country, territory, city or area or of its authorities, or concerning the delimitation of frontiers or boundaries.
For longer distances or places without existing natural gas infrastructure, ammonia ships become the most attractive option. Eighty percent of ammonia is not converted back to hydrogen but instead used directly as chemical feedstock or as fuel for the maritime sector. Transport by ship represents a relatively small component of the ammonia supply cost, so longer distances can be covered without significantly increasing the total delivered cost. The most cost- and energyintensive step is the reconversion back to hydrogen, which can be avoided by using the ammonia directly rather than as a hydrogen carrier. If the end use is a hydrogen-derived commodity, it is thus more effective to produce the commodity in the low-cost location and transport the commodity than to transport hydrogen and perform the conversion in the importing region.
The global volume of hydrogen trade by pipeline would be relatively small compared with today’s natural gas trade; by 2050, it would be about a third more than current natural gas imports to Europe. Repurposing a fraction of today’s network would therefore be enough to supply future needs. Using existing infrastructure is essential for future trade. It is also the most promising option for avoiding stranded natural gas assets and enabling low-cost regional trade of pure hydrogen.
In contrast, ammonia trade would reach almost 25 times its current level and require significant ramping up. Liquid hydrogen has a niche role in global trade. The high equipment cost – as a result of cryogenic conditions and boil-off losses (which reduce the pathway efficiency) – limit its application.
Liquid organic hydrogen carriers must overcome various challenges, including low hydrogen content, inherent high energy consumption for reconversion to hydrogen, small market size and high inventory cost. These factors restrict their role in 2050 unless technological breakthroughs occur that change the landscape.
A disadvantage of both these hydrogen carriers is that they would require further transformation; energy carriers that use the energy in the form in which it would eventually be used are more effective for global trade. The trade of commodities that embed hydrogen or require hydrogen for production, such as direct reduced iron, could be a win-win opportunity, linking good renewable resources with key materials for the energy transition while minimising the additional cost represented by shipping (Gielen et al., 2020).
New trade markets can lead to different roles for energy players. Some of the largest potential exporters of hydrogen by pipeline in 2050 are Chile, North Africa and Spain, which together represent almost three- quarters of the pipeline trade market. North Africa and Spain take advantage of their high-quality solar resources and proximity to northwest Europe, which has high hydrogen demand and poor renewable resources and leverage an existing natural gas network. For Morocco, exporting hydrogen would mean a drastic change in its energy balance, transitioning from a net energy importer to an exporter that exports more than 2.5 times its entire 2019 national energy supply solely by pipeline. Morocco – which is currently an importer of grey ammonia – could become a leading green ammonia exporter. Together with Australia and the United States, it is projected to account for three-quarters of the global ammonia trade market. Chile would also export hydrogen exporting the equivalent of 60% of its primary energy supply in 2019. On the importing side, Japan, the Republic of Korea and the Rest of Europe49 are expected to import almost all of their hydrogen and ammonia demand (IRENA, forthcoming-a).
49 Regional disaggregation includes the G20 members in Europe (France, Germany, Italy and the United Kingdom); other EU countries and the Rest of Europe (European countries that are not in the European Union).
Using IRENA’s base assumptions for capital expenditure and the weighted average cost of capital (WACC), some countries and regions with vast renewable potential could remain or even become net hydrogen or ammonia importers. They include Argentina, Indonesia, Latin America,50 the Middle East, the Rest of Asia and Turkey. In all these places, the WACC is higher than elsewhere and may continue to be so in the future. For green hydrogen and derivatives, most of the cost is in capital assets rather than operating costs; the cost of capital thus represents a large share of the total cost.
50 Latin America excludes two G20 countries (Argentina and Brazil) and two other countries (Colombia and Chile), which were modelled separately.
There is a great uncertainty about how the weighted average cost of capital (WACC) will evolve over the next 30 years (Bogdanov et al., 2019; Egli et al., 2018). Although most countries are expected to develop experience with renewables and other clean energy technologies, reducing technology risk over time, differences in country risk profiles will most likely remain. The base assumption for this study (IRENA, forthcoming-a) was that aspects other than technology that affect WACC remain roughly as they are today, with some degree of convergence.
As a sensitivity analysis, an alternative scenario was run in which all countries and regions have the same WACC, highlighting the quality of resources rather than the cost of financing projects. It narrowed some of the differences in electricity production cost between regions, reducing the incentive for trade. Global hydrogen trade is 20% lower than in the reference scenario, with almost equal reductions in pipelines and ammonia ships.
The picture looks very different in some countries. In the “same WACC” scenario, hydrogen production declines by 80% in Australia and more than 95% in Chile. The advantage these countries have today, given their relatively low WACC and excellent resources, is sufficient to justify the additional cost for long-distance shipping.
In a world in which the cost of financing green hydrogen projects is the same everywhere, the difference in resource quality alone is not enough to justify long-distance transport (especially for Chile, which is farther from potential markets). In particular, developers of large projects with access to affordable finance and large amounts of equity can significantly reduce the cost of capital for such projects, especially if they are also the off-takers or have a long-term contract with a reliable off-taker. Some difference between countries will remain, including local currency and extreme weather event risks.
On the flip side, some of the regions that would benefit from this change would be the ones that have good renewable resources but high cost of capital today. The Rest of the Middle East (excluding Saudi Arabia) would nearly triple its hydrogen production in the “same WACC” scenario, with a similar increase for Latin America. Turkey would become a net exporter (it is a net importer in the base scenario). With a lower cost of capital in North Africa and Sub-Saharan Africa, Spain would go from being a net exporter to a mere trading hub between North Africa (which has better resources) and the Rest of Europe.
The role for these countries and regions will be defined mainly by the evolution of the cost of capital between now and 2050 compared with other regions. Countries that address industrial development, supportive policy and long-term certainty on the framework with ambitious decarbonisation targets, among other issues, will see low-cost capital flowing to them to take advantage of their renewable resources, creating economic growth and opportunities (Box 5.5). Large companies with solid balance sheets and access to low-cost capital can help develop competitive green hydrogen projects at costs well below the country values used in this analysis.
Ensuring that large hydrogen projects can be financed affordably will be key, as many countries enjoy excellent renewable resources. Once the cost of transport is added, the key determinant for potential trade will be a sufficient cost differential to justify the necessary trade infrastructure.
Blended finance leveraging multilateral banks and development finance, in combination with net zero-aligned investment portfolios, will be key to deliver the scale of investment required by the energy transition at an affordable financing cost in all regions, including a scale-up of green hydrogen in regions with good renewable potential but traditionally high cost of capital, enabling international trade of green hydrogen.
FIGURE 5.12 Difference in green hydrogen production in selected countries and regions in 2050 as a result of change in the weighted average cost of capital
Hydrogen production (EJ/yr) 9
8
7
6
5
4
3
2
1
0
Middle East Australia Chile Spain
Latin America Europe Turkey Argentina Di erentiated weighted average costs of capital
Same weighted average cost of capital
This analysis provides an overview of potential developments for hydrogen trade at the global level. It does not get into the specific costs (and financing cost) of single projects, which might be substantially different, depending on project developers, off-takers and the structure of the project finance. For export-oriented investments, reliable off-takers can mitigate risks and reduce the cost of financing large projects; developers with strong track records of delivering large renewable energy projects can also access debt financing at favourable rates .
In 2050 – when technology improvement and economies of scale will have led to lower conversion and transport costs – cost differentials between countries will become relatively small. Countries will thus choose one supplier over another at a similar cost based on factors other than cost. Long-term contracts and pipelines might be the most effective means for long-term trade relations in hydrogen, as they are today in natural gas, with ships better suited for spot market deals. Other soft factors – such as diplomatic relationships, agreements and geopolitical considerations – could play a larger role than relatively small cost differentials (IRENA, 2022f). One of the most important factors is the WACC and its evolution, which could upend the outlook for some particular countries (as shown in Box 5.5) (IRENA, forthcoming-a).
Cost and quantity determine the share of hydrogen that is traded. Production cost is affected not only by the quality of the resource but also to a large extent by the capital expenditure and the WACC. The places with the best resources in the world do not necessarily translate into the places with the cheapest hydrogen (Figure 5.13) (IRENA, forthcoming-b).
By 2050, the green hydrogen potential below USD 1/kg is about eight times global final energy demand in 2020. That cost is more than three times the natural gas price in the United States in 2020; it is comparable to the cost in high-price markets in 2020 and much lower than the import price into the European Union that prevailed in December 2021, which was above USD 4/kg equivalent (in energy terms). By some measures, it may even be a conservative target by 2050. For instance, the United States Department of Energy targets USD 1/kg by 2030 through its Hydrogen Shot (DoE, 2021). Chile targets USD 1.5/kg by 2030 in its strategy, and the latest estimates point towards USD 1/kg by 2030 (Gobierno de Chile, 2021). These values are the most optimistic; with more conservative assumptions, this potential will be lower. For instance, there may be places far from infrastructure that increase the production cost and the transport penalty or make the location inaccessible. However, good renewable resources are available around the world; combined with low-cost renewable generation and electrolysis technologies, the potential for large-scale production of competitive green hydrogen is great.
FIGURE 5.13 Projected levelised cost of hydrogen in 2050
Land not suitable for PV/wind electrolysis
LCOH (USD/kg)
0.6 1.0 1.5 2.0 2.5 3.0 3.5 4.0 4.5 <5.0 LCOH >5 USD/kg
Sources: IRENA, based on (Maclaurin et al., 2019; Mattsson et al., 2021) Notes: Exclusion zones: protected areas, forests, permanent wetlands, croplands (partial exclusion), urban areas, lands with a slope of 5% (for PV) or 20% (for onshore wind), and areas with population density greater than 130 people/km2. Cost assumptions are as per Figure 5.11. See (IRENA, forthcoming-b) for details. Disclaimer: This map is provided for illustration purposes only. Boundaries and names shown on this map do not imply the expression of any opinion on the part of IRENA concerning the status of any region, country, territory, city or area or of its authorities, or concerning the delimitation of frontiers or boundaries.
Hydrogen is not internationally traded today. Governments need to act in many areas if it is to be traded in the future. A market needs to be developed by creating enough hydrogen demand, with price transparency and competition that lead to an efficient allocation of resources. Costs across the entire value chain, from production to transport and end use, need to come down, as they are currently higher than the costs of alternatives. A mix of innovation, technology improvement, demonstration and scaling up will be needed to create global hydrogen markets.
A certification scheme needs to be put in place to make it possible to trace renewable energy all the way to green products, potentially inducing consumers to pay a premium. The certification scheme needs to be done with a consistent methodology and approach. Mobilisation of large investment flows is also needed to finance the more than 10 TW of renewable energy, 4.4 TW of electrolysis and 1.6 TWh of batteries that are needed to produce green hydrogen (IRENA, forthcoming-a). Innovative approaches to blending development and climate finance with private equity will be needed to deliver the scale and pace required by the energy transition.