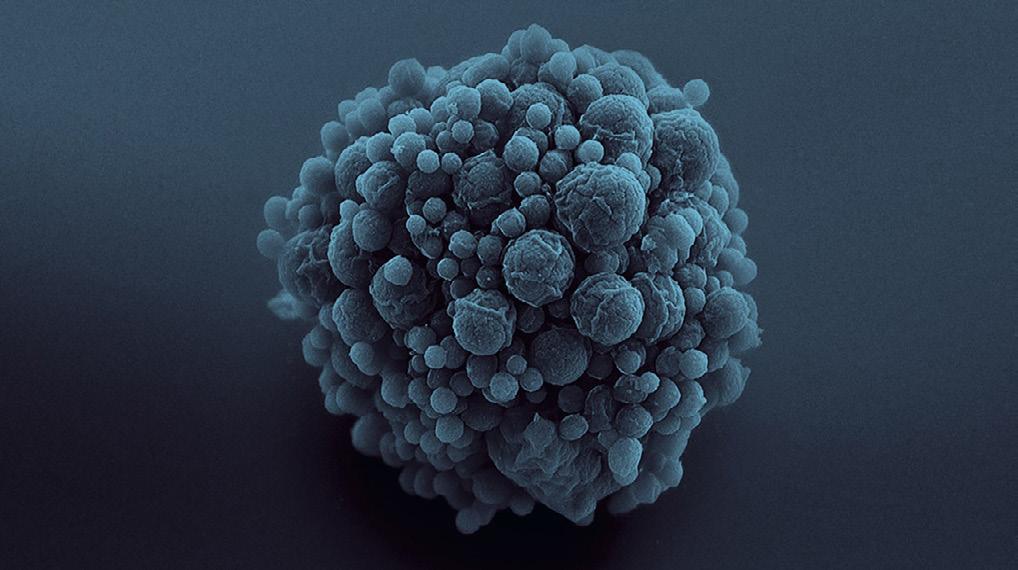
9 minute read
Origin? The story of the replicator. Jonas Flohr
Origin? The story of the replicator.
Jonas Flohr Upper Sixth
Craig Venter’s new bacterium was a major breakthrough in the world of synthetic biology
It is 3.8 billion BC. We are on a planet 151.7 Somewhere within this chaos, somewhere million km away from the fuel of the solar within Earth’s upper atmosphere, a system, the Sun, and located in a galaxy that chemical reaction ignited by ultraviolet would later be named the ‘milky way’ by its radiation from the sun and by the power future inhabitants, Homo sapiens. At the of lightning fuses several atoms to form a moment our planet is but a giant mass of small organic molecule. There is something rock in space, formed by the accumulation special about this molecule, for it is capable of material from a solar nebula. But it is of acting as a template for other atoms to about to become one of the most unique combine and form more of itself. It is an places in the entire universe; at least as enzyme with an affinity for its own kind. far as we are aware. Because of its ability to selfThe conditions are replicate, we shall call our just right. The scene molecule the ‘replicator’. has been set. The replicator begins to If a Homo sapiens copy itself and gradually were to travel back more and more replicators in time, he or she accumulate in Earth’s would see an entirely oceans. The replication different world. It process is never perfect. is a hostile planet, Sometimes mistakes are its atmosphere made, producing replicators containing mainly methane gas, water that may vary in composition, prevalence vapour, ammonia and hydrogen, as well time (how long the replicator exists as a little carbon dioxide and nitrogen. before it breaks apart again), frequency Far beneath the thick, dense layer of of replication, and replication accuracy. gases, volcanoes spew out their lava and ashes into the skies. Lightning pierces Then something important happens. The the darkness, thunder crashes; a never- supply of ‘building block’ substrate atoms ending storm. of the replicator enzyme becomes limited,
so much that growth of the replicator population can no longer increase exponentially. For the first time in Earth’s history, we have a selection pressure. Now one should be careful with the term ‘natural selection’ because we are only talking about simple molecules, yet essentially, it is a primitive form of natural selection. We have all the criteria that are required: 1. Reproduction - generations of replicators are formed. 2. Heredity - replicators identical to their precursors are formed. 3. Variation - due to ‘mistakes’ that occur during the replicating process. 4. A selection pressure - due to the limited supply of resources (the substrate atoms). This results in a primitive form of competition amongst replicators for ‘building block’ substrate material, and, due to the variation among the replicators, some are more likely to replicate and produce replicator ‘offspring’. Evolution has been ignited.
There are many reasons as to how a ‘mistake’ (we would call them ‘mutations’ in the context of genetic changes) may prove to be beneficial to a replicator.
Take prevalence time, for example. A mutation may result in a replicator having a longer prevalence time, which would increase the chances of substrate molecules colliding with the replicator’s active site to assemble a new replicator. Replicators with longer prevalence times have a greater chance of colliding with substrate molecules and therefore have greater success at reproducing than those with shorter prevalence times. Therefore, over many generations, the frequency of long-prevalence replicators would increase in the population. Prevalence time is just an example of a characteristic - arising from random mutations - which would increase a replicator’s chance of copying and ‘producing’ offspring replicators.
Over time, the replicators evolve more efficient mechanisms which increase their chances of survival, possibly the most significant of which is the formation of the first cell: a replicator enclosed by a phospholipid bilayer. The extraordinary characteristic of phospholipids is that they form a bilayer in aqueous environments due to their hydrophilic glycerol heads and hydrophobic fatty acid tails. They would have been present in the early prebiotic oceans, and therefore it is possible that one of our early replicators, by chance, found itself surrounded by a phospholipid bilayer. Being surrounded by a membrane is a huge advantage in terms of evolution:
1. It prevents the enclosed molecules from reacting with outside molecules - hereby providing continuity in the composition of materials inside the cell.
2. It provides a stable environment for chemical reactions (such as copying of our replicator) to occur, surrounded by an environment that is constantly changing.
3. Any other proteins (such as enzymes) which the replicator codes for are available only to the replicator itself, hereby increasing the efficiency at which the replicator can work.
The cell is greatly favoured by evolution, begins to multiply, and the frequency of cellular structures increases in the population. Slowly, over time, more complex structures evolve, leading ultimately to highly complex organisms like us, Homo sapiens. Now there are many theories as to how life on planet Earth began. The discovery of DNA by the Swiss chemist Friedrich Miescher in the 1860s, as well as the discovery of DNA’s 3D helical structure by Watson and Crick in 1958 have given us a ground-breaking leap forward in terms of being able to track back where we come from, all the way back to the first ever living cell.
Yet this is where we have a problem: DNA cannot replicate without enzymes inside the cell. Enzymes, on the other hand, cannot be synthesised without the genes which code for them, carried by DNA.
So, which came first? This is where our story from earlier comes in, which is but one of many theories as to how life on planet earth began. A solution to this ‘chicken and egg’ situation would be a molecule which combines both the chicken and the egg: one that is both able to hold genetic information as well as being capable of replicating itself without having to rely on protein machinery.
There have been several proposals for what molecule this may have been. Over the past century, the ‘RNA World Hypothesis’ has gained particular momentum and support among experts, suggesting that this nucleic acid may prove to be the solution.
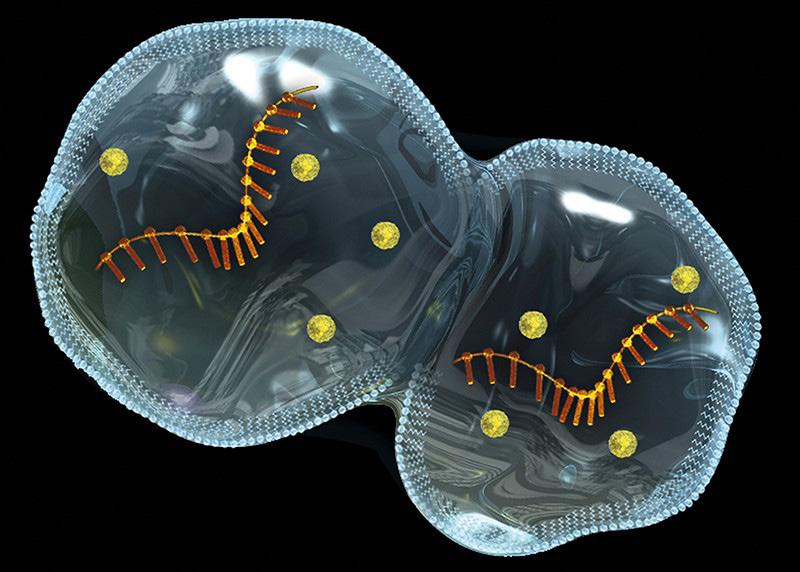
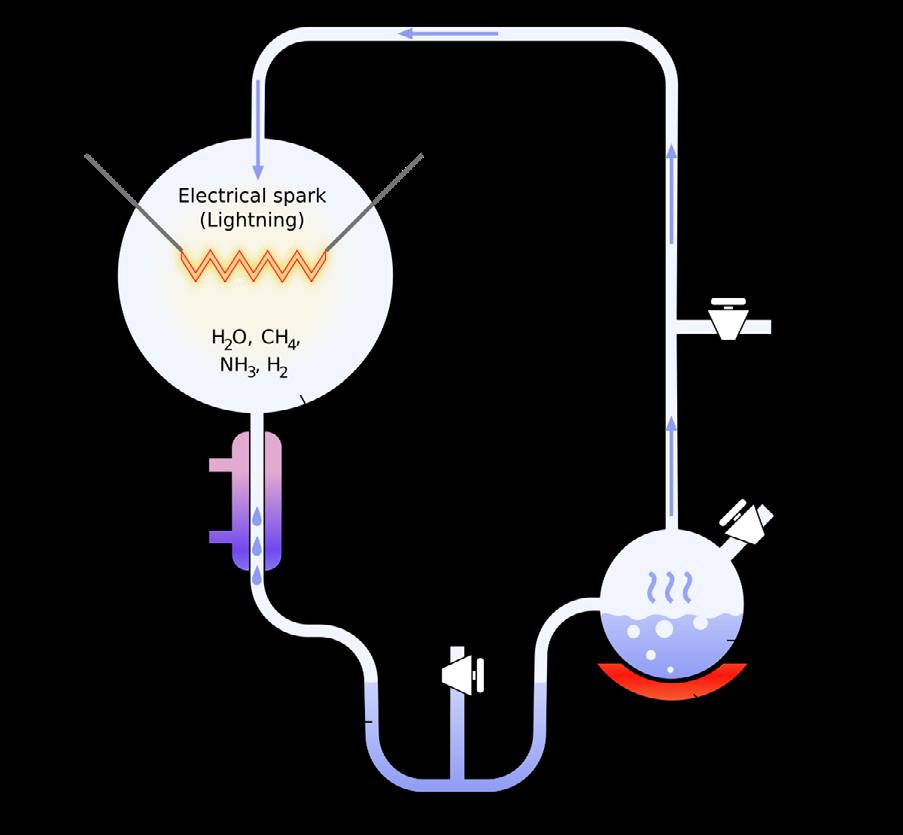
The Miller-Urey experiment creating early ‘building blocks’ of life
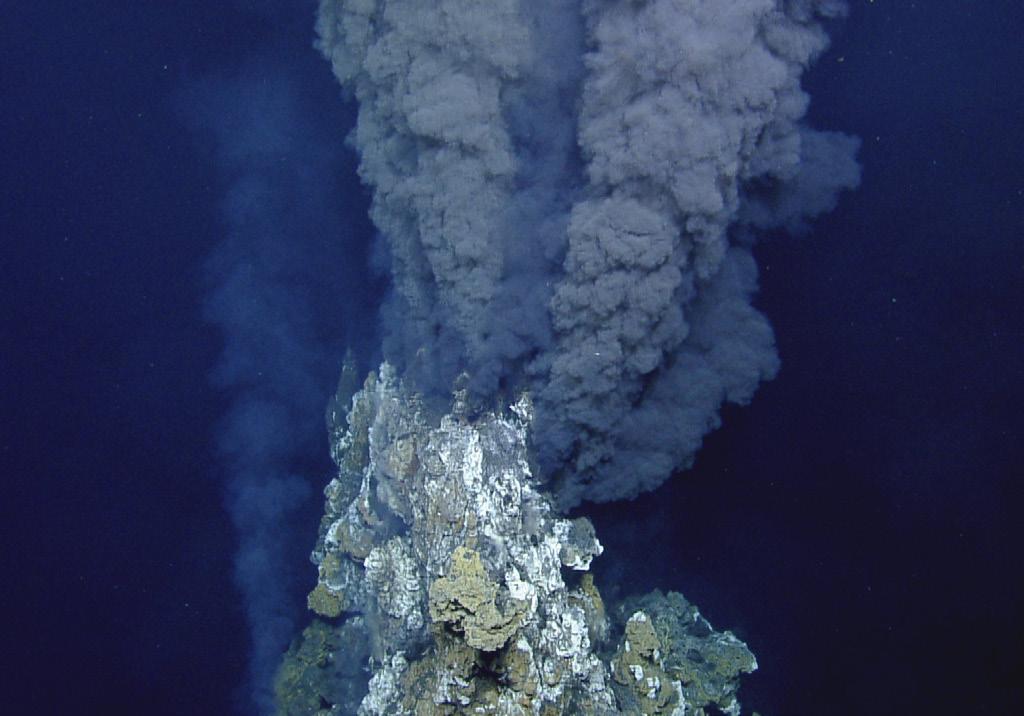
In the 1920s, scientists Alexandr Oparin and John Haldane proposed that life on Earth developed from non-living matter in a process of “gradual chemical evolution”. The Oparin-Haldane theory suggests that simple, inorganic molecules reacted to form ‘building block’ molecules such as amino acids and nucleotides, which accumulated in the oceans to form a primordial soup. These then combined to form larger structures capable of selfreplication. Hypothermal vents might have been typical sites for such an occurrence, as higher temperatures would promote chemical reactivity.
Oparin and Haldane’s theory was strengthened by experiments made by Harold Urey and his student Stanley Miller in the 1950s. Urey and Miller designed a simple experiment in which they tried to recreate conditions similar to those which would have been present on the early Earth. They constructed an apparatus that linked a vessel containing heated water to a container of methane, ammonia, and hydrogen. They set up two metal electrodes on either side of the container and sent sparks through the gases, mimicking lightning. After a few days, examination of the contents of the water container showed that it contained large biological molecules, including amino acids. The Miller-Urey experiment was ground-breaking in the fact that it proved that biological molecules could be created from simple inorganic compounds under conditions similar to those present on our early planet. The theory suggested by Oparin and Haldane seemed more and more credible.
The Miller-Urey experiment was certainly a step forward. Yet, ground-breaking as these findings were, from a broad perspective, we still knew relatively little. How did these basic molecules then develop into more complex molecules capable of selfreplication? What kind of molecules were the early replicators? This is where the RNA World Hypothesis comes in.
Research over the past century has shown how special RNA truly is. It was in the 1960s that Carl Woese, Francis Crick and Leslie Orgel proposed that the first forms of life may have used RNA as their genetic material, as it is both capable of storing genetic information (as it does in RNA viruses such as HIV or Coronaviruses), and has the ability to act as an enzyme itself (ribozymes, for example, are RNA enzymes that self-catalyse gene splicing of introns by a phosphoester transfer mechanism). In 1989, research by Nobel Prize winners Thomas Cech and Sidney Altman showed that it was the RNA at the centre of ribosomes which acted as the catalyst in protein synthesis, not the protein (ribosomes are composed of both RNA and proteins). Many scientists decided to conduct further research into RNA’s potential, trying to synthesise alternative forms of the nucleic acid, whilst mimicking conditions which would have been present on the early Earth.
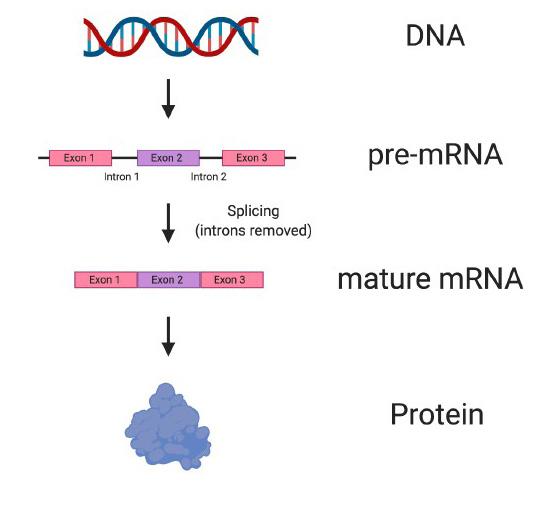
Biologist Gerald Joyce, for example, managed to synthesise two RNA molecules which were capable of synthesising each other through a positive-negative correlation, using only four component substrates. These had a replicating time of approximately one hour and could do this without the help of any proteins or other biological molecules. Furthermore, populations of the RNA enzyme molecules could be made to compete for limited resources within a common environment and would occasionally give rise to different variants, which were still capable of replicating. Joyce writes: “Over the course of many ‘generations’ of selective amplification, novel variants arise and grow to dominate the population based on their relative fitness under the chosen reaction conditions. This is the first example, outside of biology, of evolutionary adaptation in a molecular genetic system.” Joyce had been able to construct a scenario which strengthened the idea that RNA may have been involved in the early steps of development of life on Earth.
However, our idea of the early Earth, as well as how life on Earth first came to be is, to put it simply, microscopic. Without proper evidence, we can do nothing but speculate and construct theories as to how life may have evolved. The Urey-Miller experiment, as well as research into RNA’s potential as an early replicator in recent decades has certainly been a great step forward in perhaps uncovering the mystery of how life may have evolved on Earth. However, the big questions still remain unanswered. In 2010, biologist Craig Venter and his team synthesised their own sequence of DNA, which they then injected into a bacterium, whom they cleverly persuaded to replace its own chromosomal DNA strand with the synthesised one. As ground-breaking as this may be, Venter had still not managed to create his own life form. As Nobel Prize winner Richard Feynman once said: “What we cannot make, we do not understand.” So far, nobody has been able to create life from non-living material. So, one of the most fundamental questions remains: what was the spark that ignited life? What is the ingredient that makes life living? Science simply does not (yet) know the answer.