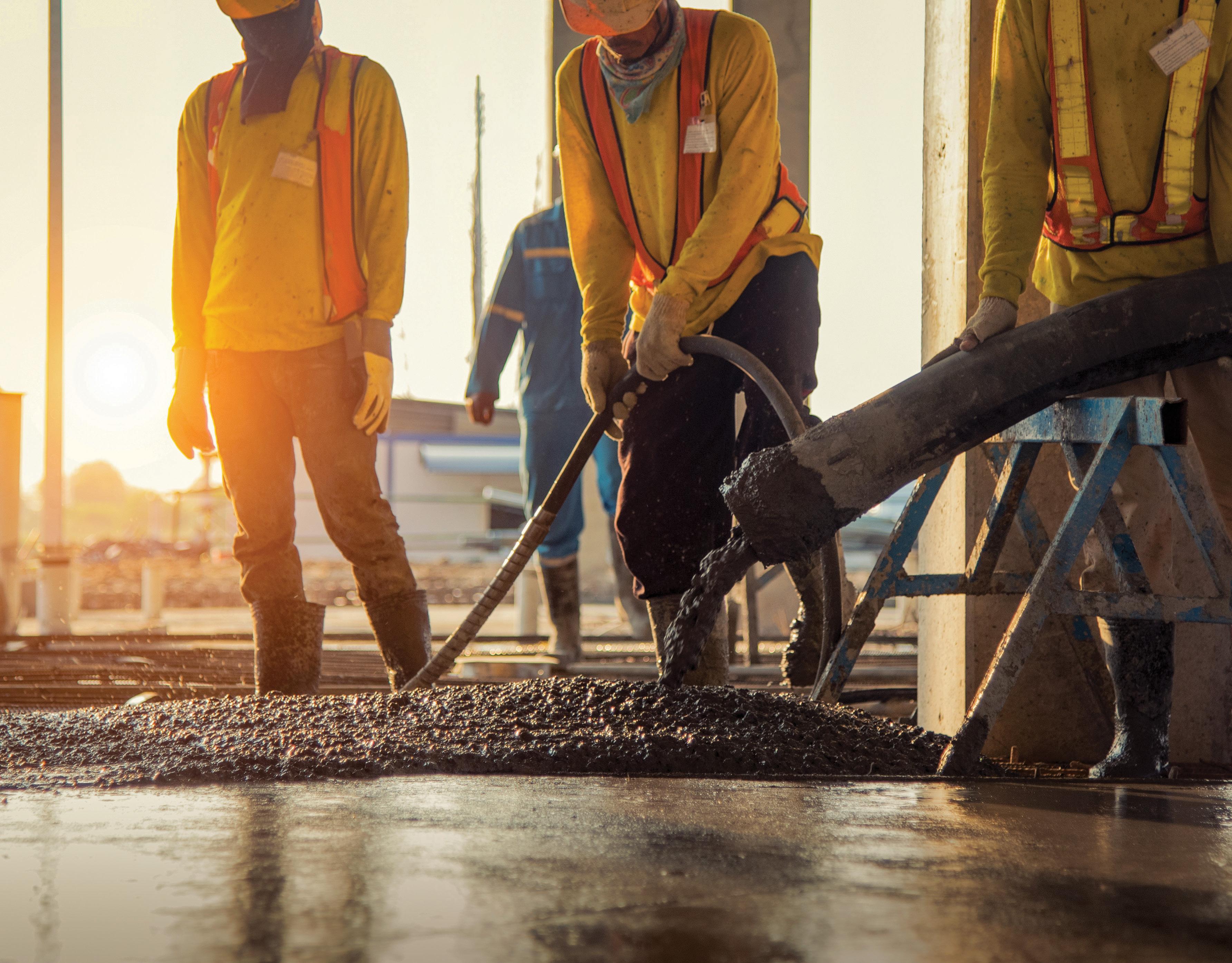
11 minute read
The Briefing
Concrete
The Quest for Carbon-Neutral Concrete
MIDDLE EAST Charles K Nmai, President of the American Concrete Institute, shares his thoughts on how the construction industry can work towards meeting carbonneutrality goals through concrete
It’s universally accepted that achieving carbon neutrality must centre around Portland cement and, accordingly, cement organisations such as the Portland Cement
Association (PCA), the Global Cement and Concrete Association (GCCA), and the Inter-American Cement Federation (FICEM) have either developed or committed to industry roadmaps for netzero concrete—that is, to fully decarbonise the cement and concrete industry by 2050.
These roadmaps involve different strategies and include savings in clinker production, savings in cement and binders, efficiency in concrete production, and efficiency in design and construction— which speaks to the objective of ‘construction productivity’.
This includes the decarbonisation of electricity, carbon capture and use/storage, and the re-carbonation of concrete.
Savings in cement and binders and efficiency in concrete production are not new to the industry. Supplementary cementitious materials (SCMs) such as fly ash, slag cement, calcined clay/metakaolin, silica fume, and natural pozzolans are used worldwide, depending on availability, to optimise Portland cement concrete.
Other SCMs such as rice husk ash and, in recent years, ground-glass pozzolans are also available regionally. Blending SCMs with Portland cement during the manufacturing process is also not new, though not extensive in North America, where concrete producers typically add SCMs separately, usually as a replacement for Portland cement.
The main difference between blending during manufacturing and separate addition is the Portland cement
75%
replacement that can be achieved by the latter. Separate addition offers flexibility with respect to the types and amounts of SCMs that can be used in a given concrete mixture. Historically, fly ash and slag cement replacement levels have ranged from about 10 to 30% and 25 to 50%, respectively, and the higher ends of the ranges have typically been used in mass concrete applications.
Concrete mixtures with combinations of SCMs are currently being used to achieve significant replacement levels of Portland cement in an effort to reduce the carbon footprint of the mixtures. Portland cement replacement levels of 70 to 75% are being used routinely in projects throughout the United States, particularly in high-rise buildings in New York City and California, and replacement levels of about 83% have been reported.
These low cement content concrete mixtures require proportioning expertise and the right choice of chemical admixtures to meet project requirements. The projects in New York City include One World Trade Centre and its companion towers, 432 Park Avenue, and 30 Park Place (Four Seasons hotel)—all constructed between 2006 and 2017 with mixtures that contained fly ash, slag cement, and silica fume (high-reactivity metakaolin in the case of 432 Park Avenue for aesthetics).
The San Francisco Public Utilities Commission Headquarters building, constructed between 2009 and 2012, had a 70% replacement of Portland cement using fly ash and slag cement. Not to be outdone, the concrete mixtures for the Goethals, Bayonne, and Tappan Zee Bridges in New York City, constructed between 2013 and 2017, had Portland cement replacement levels of 55 to 65% using fly ash and slag cement (plus silica fume for Tappan Zee).
What is unique about these projects, and all the others not mentioned, is that the owners, through their design professionals, required the use of concrete mixtures with a low-carbon footprint. Indeed, the push for low-carbon concrete is currently being driven by owners.
Greater success can be achieved if design professionals proactively take the lead and collaborate with stakeholders to achieve net-zero concrete by 2050! Also fascinating is the fact that common SCMs—fly ash, slag cement, silica fume, and highreactivity metakaolin—were used.
A wealth of information on these SCMs already exists in ACI documents, and one of our immediate goals is to repackage the information for the general public and highlight and provide a list of low-carbon SCM concrete projects.
Traditional SCMs have been used successfully to significantly reduce the carbon footprint of concrete; however, there are issues that must be acknowledged. Issues include availability, depending on geographic location; lack of storage silos at concrete plants for SCMs; and code limitations on SCMs for air-entrained concrete mixtures exposed to de-icing chemicals in service.
These issues can translate into higher costs. For example, Portland cement and SCMs must be shipped to Hawaii, making SCMs more expensive for design professionals on the islands.
The need to lower the carbon footprint of concrete has led to increased production of Portland limestone cement (PLC) and increased focus on alternate cements and binder materials.
Alternative options
The global cement industry is exploring different concrete mixtures to find a way to reduce their carbon footprint.
Achieving carbon neutrality
Efforts to achieve carbon neutrality must centre around Portland cement, says Charles K Nmai.
2050
Industry stakeholders hope to achieve net-zero concrete by the target date of 2050
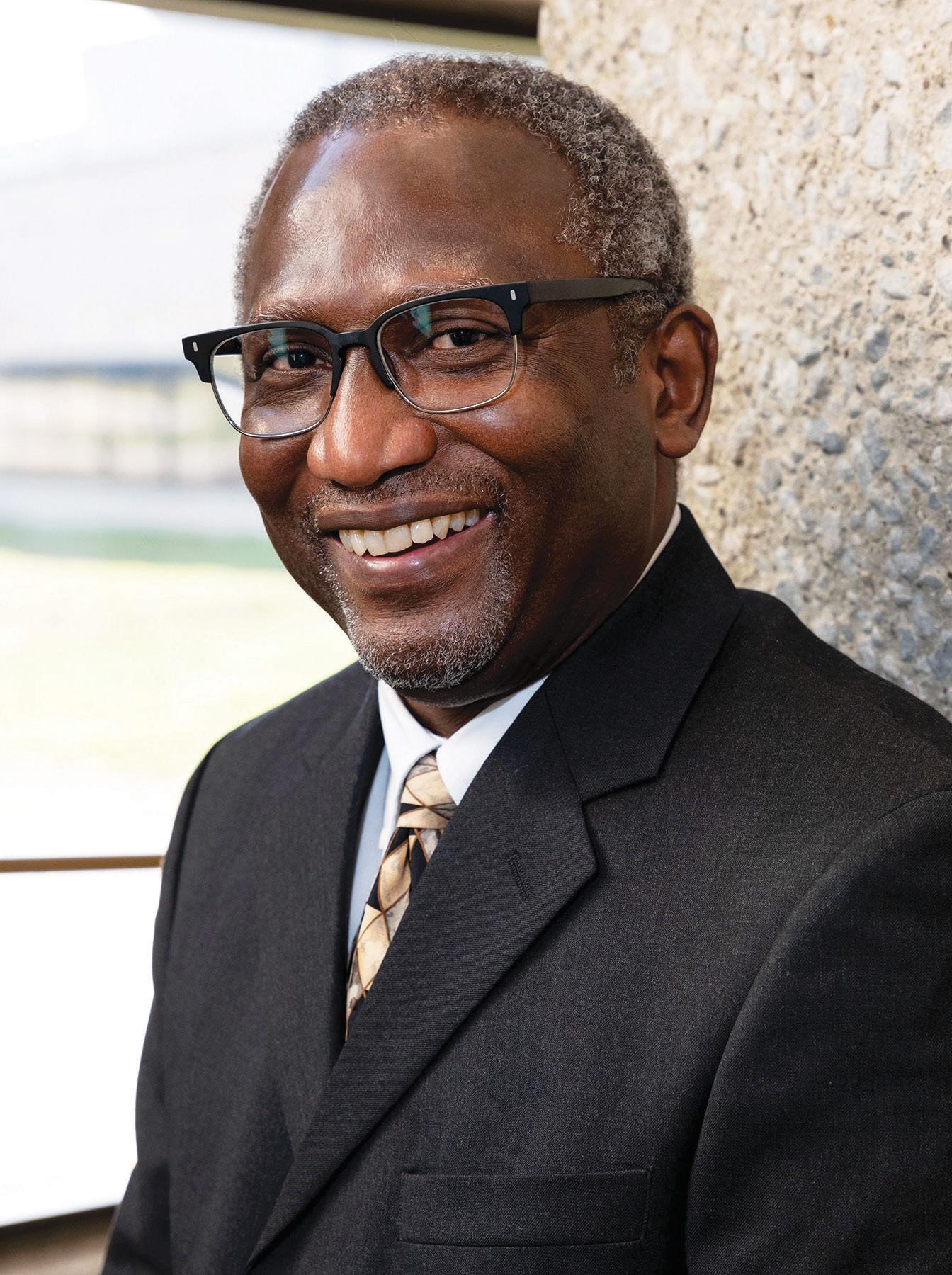
In 2018, ACI Innovation Task Group (ITG) 10 published two documents on these materials: ITG-10.1R-18: Report on Alternative Cements; and ITG-10R-18: Practitioner’s Guide for Alternative Cements. Readers should note that an alternative cement is defined as “an inorganic cement that can be used as a complete replacement for Portland or blended hydraulic cements, and that is not covered by applicable specifications for Portland or blended hydraulic cements.”
Binder materials covered by this definition include geopolymers, activated glassy cements, activated fly ash cements, activated slag cements, calcium aluminate cements, calcium sulfoaluminate cements, magnesia cements, and CO2-cured cement. Several of these alternative cements have been available and used in niche applications prior to the current focus on low-carbon concrete.
The publication of the two ITG reports preceded the inclusion of alternative cements in ACI 318-19 with the stipulation that the use of such cements is permitted if approved by the licensed design professional and the building official. ACI 318-19 also requires the approval to “be based upon test data documenting that the proposed concrete mixture made with the alternative cement meets the performance requirements for the application including structural, fire, and durability.” The need to have test data documenting performance for the application points to the need for proper assessment and validation of alternative cements and other innovative materials for the concrete industry that are not covered by existing standards and specifications.
The pursuit of low-carbon concrete has inspired the introduction of a wide range of products promoted to minimise the Portland cement content of a concrete mixture. These include, but are not limited to, CO2 injection systems, admixtures based on synthetically produced crystalline calcium silicate hydrate (CSH) nanoparticles, synthetic limestone aggregates manufactured from waste CO2, and a powdered admixture based on a blend of dry-expanded polymeric microspheres and mineral powder that eliminates the need for air entrainment in frost-resistant concretes.
Into the Metaverse: Fusing the Real World with the Digital
MIDDLE EAST Drew Gilbert, design manager at OBM International, looks at the opportunities presented by the metaverse for the AEC industry
Anew architectural era is among us, the creation of place making has teleported to a parallel world. A realm that bypasses physical constraints of materiality, structural integrity and enclosure from the physical elements. This has unleashed a new kind of reconceptualization of what architecture is, what it can be, and how we will experience it. As the metaverse concept has been around for decades, it has become a buzzword with the announcement of Facebook becoming Meta and billions of dollars being invested into metaverse technologies. Experiencing the internet will have a new connotation as we enter ourselves into the 2D screen into a fully immersive 3D world; A 3-Dimensional world of connectivity that enables users to shop, visit friends and family, meet with colleagues and clients all in one place. We must ask ourselves the question on how to best utilise this evolving technology to enrich our lives and the built environment.
A 3-DIMENSIONAL WORLD OF CONNECTIVITY The metaverse will become a collection of fully immersive real-time environments interlinked/interoperable with one another where users (in the form of avatars/meta personas) can interact with the environment and each other either for business/entertainment purposes in decentralised and co-created worlds. In short, the metaverse is a virtual reality version of the internet where the outside will be shared as a digital form. It is all possible thanks to the emergence of Web3.0 technology, a world driven by data, decentralisation, and user autonomy.
The easiest way to visualise the transition from Web2.0 to Web3.0 is like moving from 2D to 3D. The existing protocols and services of today’s internet
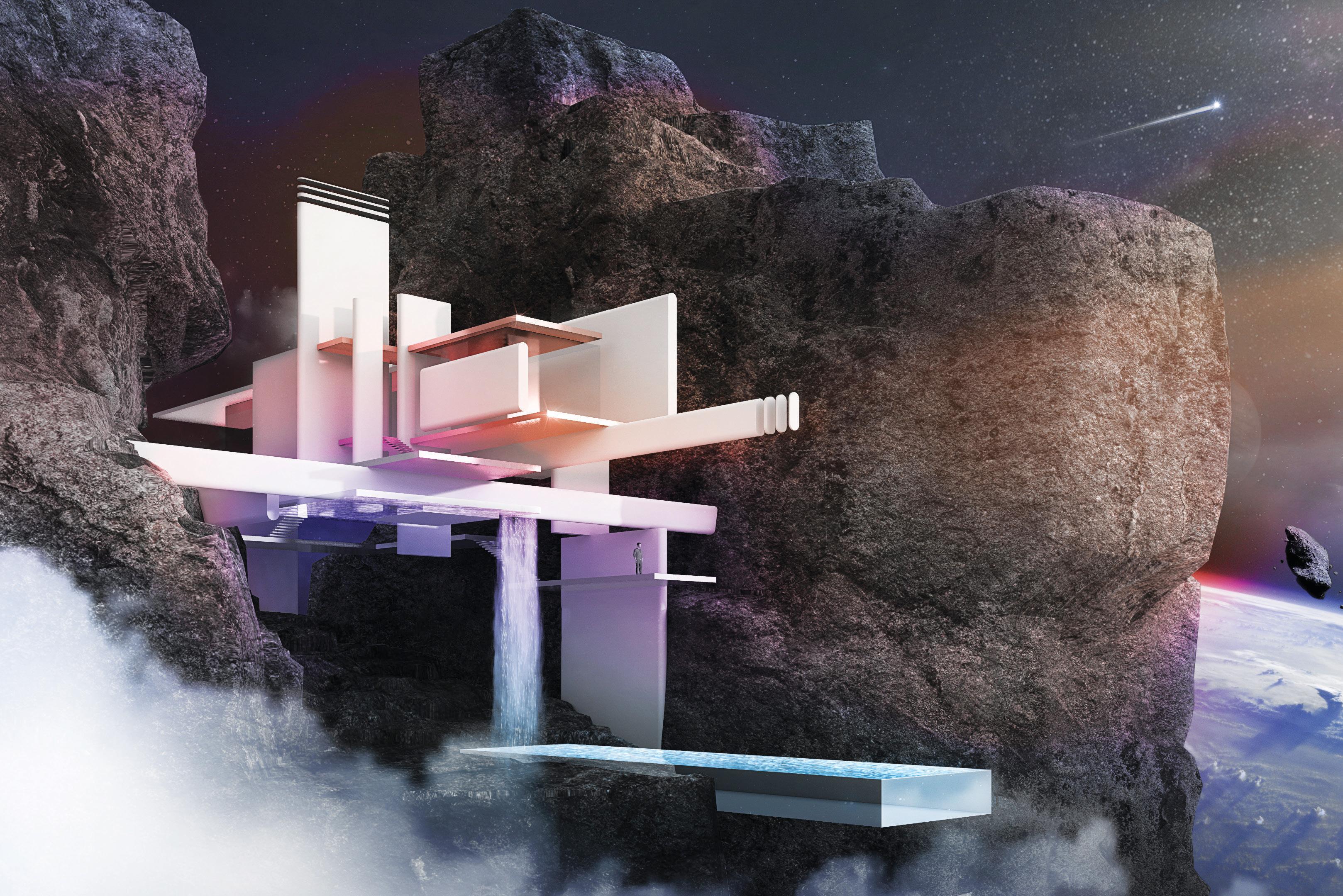
will still be visited, however with Web3.0, we will start to see services built on permissionless blockchains with open protocols, open standards, and built within open “worlds”. This provides massive opportunities for individual users, content creators, and businesses to expand and explore the latest evolution of the internet. While the infrastructure behind Web3.0 is still in the works, the future of the internet looks promising and it’s arriving faster than we think.
The primary aspect of architecture was intended to provide shelter to human beings from external actors, in the metaverse this is no longer the case. An entire new world of possibilities has been unlocked. What kind of expression, creativity, and design has become possible? As technology advances, the philosophies continue to evolve.
Each metaverse platform has a set of rules, for example a set plot size that may limit the height and footprint, polygon count that may limit the detail of the design, or even a cap on the number of users that can access at once, these new constraints will create a new kind of architecture and it will continue to adapt as the metaverse evolves. The methods of conceptualizing architecture in the metaverse will need to be reimagined.
As Architects building and shaping the future of Metaverse Architecture, we will start to incorporate aspects of multiple fields, for example user interface, content design, character design, landscape development, blockchain based protocols, and even game design. Within the metaverse there will be multiple virtual worlds that contain a mix between both the physical and digital world, where people can experience and engage in a vast range of activities.
Most activities will be centred around social activities like meeting with friends, clients, and loved ones, but there is also a utility aspect that can enable education to be easily accessed around the world, or even have easy access to governmental agencies and healthcare that are operated 24/7 by expert digital assistants.
Each of the current metaverses offer different possibilities; A creator entering the metaverse must first ask what the most important aspects are of what they are trying to achieve. Aspects like daily
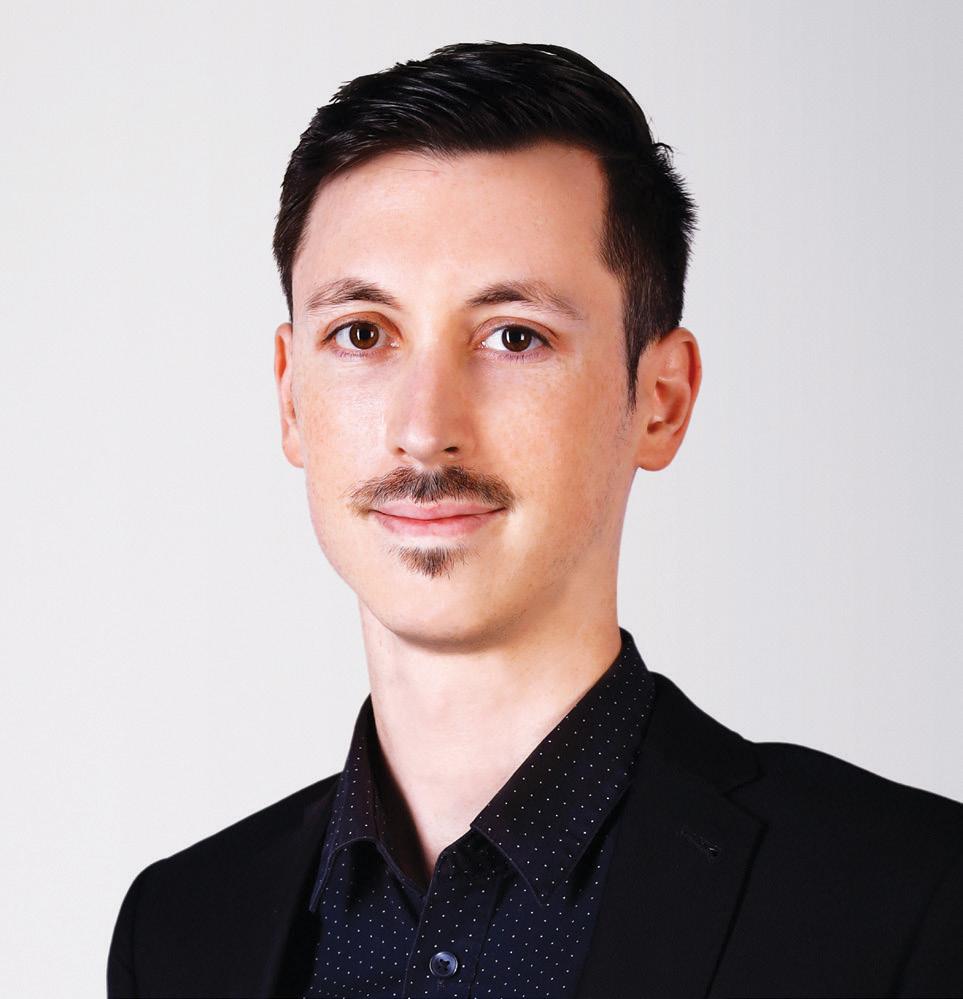
Different possibilities
Drew Gilbert says that different metaverses offer different opportunities to creators, designers and architects.
Decarbonising the Earth
Metaverses can utilise Digital Twins technology to represent the physical world and help control energy and emissions.
2030
user count, age and demographics of users, open or closed platforms (either enable full freedom of creation or to work within a “design” framework) along with other factors will determine which metaverse platform will best suit their needs. Entering a metaverse may or may not require the purchase of digital land in the form of a Non-Fungible Token (NFT) and purchased with the native cryptocurrency of the platform.
The structures in the metaverse are made of polygons or voxels that is defined as three dimensional pixels. Each one of the current metaverses offer different options. While some provide a sprawling, colourful, low poly wonderland. Expanding to other more tightly detailed worlds that focus on high-quality renders that explore niche ideas. And others use cubes as a standard building block for each ethereal build and an ingrained emphasis on recreating an Earth-like world.
OPPORTUNITY TO DE-CARBONISE THE EARTH As we are heading towards the next big-tech paradigm shift, we must consider the impacts it may have on our planet. Many blockchains such as Ethereum verify transactions through a method called “Proof-of-work” and this process is an energy consuming process where hundreds of computers on a network solve complex cryptographic
The metaverse will become a collection of fully immersive realtime environments interoperable with one another where users can interact with the environment and each other in decentralised and co-created worlds”
puzzles to verify transactions. Simply put, every transaction that is made using cryptocurrency on a PoW blockchain is energy consuming. The amount of energy consumed for the blockchain along is estimated to be 150 terawatt hours annually.
Luckily there are hundreds of blockchains all working towards a more sustainable future. Ethereum network which is the major network that powers the majority of the virtual transactions will be rolling out the next generation that will move to “Proof-of-Stake”, which reduces energy consumption while providing the same transaction verification. In addition to recycling e-waste and setting up 100% renewable energies datacentres, for example, Google and Microsoft are setting up run data centres on completely carbon-free energy by 2030.
Another way the metaverse can help in that is by using Digital Twins. Digital twins represent the physical world and allow users to interact with these digital replicas from anywhere and in a number of productive and efficient ways. They are especially effective in helping to control energy usage and carbon emissions.
The options are limitless, and the opportunities being presented by governments all over the world are making it more attractive and affordable to rethink how technology can help reduce greenhouse gasses.