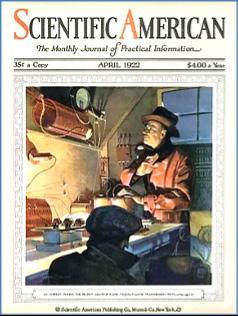
26 minute read
Legacies Of The 1921 Transatlantic Tests
By David and Julia Bart
By 1920, radio, or rather wireless telegraphy, was still fairly young, but the first generation of wireless explorers were now aging or passing away. That year, the American Institute of Electrical Engineers awarded Profesor Michael Pupin its Edison Medal for his work in math and physics associated with wireless communication, and, the Institute of Radio Engineers awarded Guglielmo Marconi its highest honor, a Gold
Medal, for his work. These two highly prized annual recognitions continue to be awarded today as the
Institute of Electrical and Electronic Engineers’ (IEEE)
Medal of Honor and the IEEE’s Edison Medal. It had been nine years since Marconi received the Nobel
Prize in 1909 for his early wireless research performed at the dawn of the 20th century. Professor A. Righi,
Marconi’s teacher who inspired Marconi’s lifetime of research, died in 1920, and Dr. Alexander Muirhead, who was associated with Sir Oliver Lodge’s early wireless experiments, also passed away. The Titanic sank in 1912, and a terrible World War recently ended in 1918.
These events and others all brought wireless telegraphy and the promise of radio into the public eye.
The ensuing few years would set the stage for a century of radio development. That progress is directly linked to a set of momentous experiments conducted in 1921 by members of the Radio Club of America (RCofA) and the Amateur Radio Relay League (ARRL). This article explores some of the legacies of those 1921 Transatlantic Tests. (See related articles in this issue of the Proceedings for descriptions of the tests and biographies of the participants.)
Cover of Scientific American (April, 1922) depicting an incident in the Transatlantic Tests, from an oil painting by Howard H. Brown depicting the Ardrossan, Scotland station.
RADIO IN THE EARLY 1920S
The first regularly operated wireless stations in the U.S. were located at Siasconset (Nantucket), Massachusetts in and on the Nantucket Shoals Lightship No. 66 in 1901. Twenty years later, the U.S. government had licensed 5,972 wireless stations plus 135 government shore stations and 470 ship stations, and an estimated 10,800 amateur radio operators were participating in radio operations. Wireless telegraphy and broadcast radio in the years 1920-1923 underwent profound changes. After World War I concluded, thousands of military trained radio operators returned home to join the ranks of amateurs and professionals interested in wireless. Americans in particular launched into the roaring 1920s filled with energy and optimism. The world of wireless was romantically and excitedly described in the popular press with dewy-eyed editorials speculating on the potential of wireless to establish a permanent world peace. The exploits of amateur wireless operators featured prominently in those press accounts. Amateurs, inventors, institutions, the press, and the public all interacted to spin a new fabric of meanings, and implications, for a wireless future around the new medium. Yet, ever since the Radio Act of 1912, amateurs remained exiled to the shortwave end of the spectrum, while military and commercial interests; especially, the Marconi Wireless Telegraph Company of America (American Marconi) and its successor, the newly formed Radio Corporation of America (R.C.A.), operated with their choice of frequencies. Broadcasting had become a privilege, not a right, and World War I left government, the military, and corporate interests in control. In November 1919, wartime restrictions lifted, and amateur radio operators were back on the air. They soon enjoyed a freewheeling, dynamic renaissance after enduring years of silence during the war when operations were prohibited by law. Now, permitted to return to a world 200 meters of wavelength and down, experimentation, contests of skill, new discoveries, and collaboration, as well as competition, marked an exciting
and challenging time of advances. In April 1920, QST, published by ARRL, circulated to ten thousand; by June 1923, circulation reached sixteen thousand, and women were on the air as well. As the airwaves filled with activity, radio operators sought contacts that were further and further away.
REACHING FOR DISTANCE
Efforts to transmit and relay signals across the U.S. stirred the imagination; and in January 1921, amateur transcontinental tests sponsored by the ARRL were successful, with one relay making its way across the country in just six and one half minutes. In early 1921, ARRL founder Hiram Maxim Percy, 1AW, managed a two way contact with V. M. Bitz, 6JD, in California. Why not reach across the Atlantic? Some claimed to have succeeded, but they lacked confirmations, and no satisfactory results had been achieved. In February 1921, ARRL announced plans to sponsor contests that made the attempt, but these first trials failed due to insufficient planning in too short a time period. The ARRL coordinated a new second set of tests, scheduled for December 1921, by publishing notices to American readers in QST and to British amateurs in Wireless World and Radio Review. ARRL further publicized its tests at its first convention, held in Chicago in September, where protocols, equipment, confirmations, and individuals were selected to participate. The Radio Club of America (RCofA) embraced the challenge, although late in its own planning, and rushed to develop its approach (see separate articles in this issue of the Proceedings). Edwin H. Armstrong led the U.S. team to transmit, and Paul Godley went to Scotland on behalf of the team to receive. Results were transmitted back to America via Marconi Company’s Carnarvon Radio Station, MUU, and were received by R.C.A.’s station, WII. Godley later regretted not sending his own confirmations, but he had been prohibited from operating his own transmitter in Scotland since he did not have a British operating license. On December 7, 1921, Godley heard an American amateur, 1AEP (later verified as 1AAP); and on December 9, 1921, he heard the RCofA’s station 1BCG, reporting back that “Signals from 1BCG were steady and reliable” and recording the “Remarkable performance.” Soon, he heard 30 more American amateur stations. Eight British stations also received transmissions from American amateurs. The tests were a resounding success, and signals had traversed the Atlantic on less than one kilowatt of power! Several records were set 1BCG during the tests, including distances of 3,800 miles to Amsterdam and 2,600 miles to California, and its signal was heard in all 50 states as well as Puerto Rico and Vancouver, British Columbia. By February 1923, American amateurs reported many contacts with British and French operators. By the following year, two-way transatlantic contacts became commonplace and worldwide communication had been launched. The story is remarkable, but what did it mean? What were the longer term impacts of the 1921 tests? For RCofA, a bright future was in store since, in the words of George Burghard, “The organization (RCofA) belongs to no one branch of the radio art, but to all branches, and therefore its duty at present must necessarily be one of education.” For the industry, change was on the horizon.
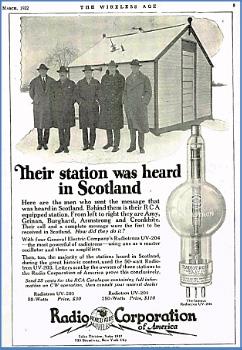
1922 Radio Corporation of America advertising in Wireless Age, noting that RCA UV-204 vacuum tubes were used at station 1BCG during the Transatlantic Tests.
STANDARDIZATION AND CONTROLS
Prior to 1920, amateur radio was “self-sufficient and self-contained.” The rapid expansion of amateur and commercial broadcasting necessitated increased regulation worldwide to insert some form of control over the expanding chaos on the airwaves. The First National Radio Conference took place in March 1922 to revise Federal laws and licensing. Global call signs were initiated in 1923, QSL cards proliferated as an accepted means of acknowledging contacts, standardized language developed for communication and acknowledgement (Roos’ International Auxiliary Language, ILO and Radio Auxiliary International Language Society, RAILS), QST English became accepted with its language of Q codes, amateur radio organizations proliferated, and by 1928, the International Amateur Radio Union (IARU) ratified its constitution following the 1927 International Radio Conference. These were important advances that both standardized operations and helped maintain clear paths for communication. After 1921, low-power less-expensive transatlantic communication was proven viable, which opened the door to greater usage of the radio spectrum. These legal controls, accepted standards, and normalized customs enabled the growth and expansion of international radio operations to proceed in an orderly way.
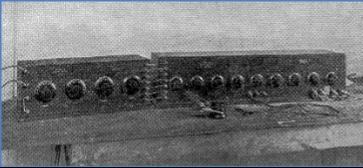
Paragon superheterodyne tuner and amplifier in the tent at Ardrossan, Scotland. Note, Godley used the Paragon RA-6, not the more recent RA10 used at 1BCG. (Courtesy QST, Feb 1922)
Interior and schematic of the Paragon RA-6 superheterodyne receiver used at Ardrossan, Scotland. (Courtesy QST, Feb 1922)
CW VERSUS SPARK
In continuous wave (CW) transmission, a perfectly uniform wave of constant amplitude is generated, leaves the antenna, and travels through space without losing its form. The distance it travels is depends upon the amount of power at the initial source. CW transmission may be obtained by several different methods, but the most popular method employed by most amateurs in the early 1920s utilized an oscillating vacuum tube. In the spark, discontinuous or damped method of transmission, the emitted wave is not continuous in form, and the amplitude of its oscillations is not constant. It is produced by the spark type of transmitter and travels through space until exhausted, or damped; thus, its distance is proportional to its initial amount of energy. CW with its constant amplitude, does not dampen out and is, therefore, known as an undamped wave. Spark based transmission required cumbersome transformers, huge condenser jars and ponderous spark dischargers of the stationary or rotary types to create the discharge of the radio wave. CW eliminated the need for this equipment. It was practically noiseless, operating conditions were greatly improved, and the operator could send and receive almost simultaneously; without having to manipulate large change-over switches to connect with the spark generating equipment or to disconnect and switch over to the receiving equipment. In 1921, spark transmission still remained the choice for long distance communication. Large, expensive, high power transmission required the ability to generate huge wattages of power that were sent crashing over the airwaves. Incredibly, the basic concept had not changed since Marconi first traversed the Atlantic with the letter “S” in 1901. Over the ensuing years, ever more powerful stations were built; and by 1920 a debate raged whether spark or continuous wave was the better, more efficient technology. The Transatlantic Tests of 1921 sent a clear message and could not be ignored; amateurs had traversed the Atlantic with only 1kW of power, and the bill to ARRL was only $1,900. It would only be a matter of time before CW would prevail.
CW AND R.C.A.’S UV-204 TUBE
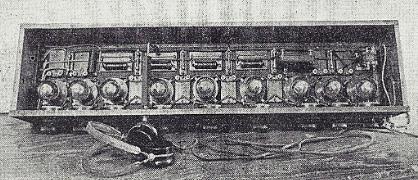
CW transmissions, when intercepted by the receiving station, are so sharp and constant in character that the receiver must be tuned exactly to the transmission’s wave length. Since tuning is critical to eliminating interference between stations, CW’s more selective methods of transmission made it preferable over damped wave forms of transmission. CW relied on the greater economy in power consumption brought by the use of radio tubes/ valves. CW could carry five times the distance spanned compared to older spark methods. It was not uncommon for a 1kW vacuum tube transmitter to outdo a 5kW spark type set. The use of vacuum tubes in transmission enabled not only CW, but also ICW, or interrupted continuous wave operation, as well as radio telephony, which combined CW and ICW techniques.
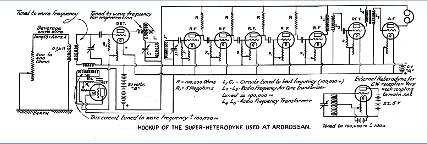
The 1921 Transatlantic Tests utilized R.C.A.’s UV-204 power vacuum tubes in the 1BCG transmitter. World War I yielded significant advances in transmitting tubes. After the war, the U.S. Navy asked to upgrade their shipboard stations to 250W and 1kW phone transmitters. Beginning in March 1921, the final stage tube used in these transmitters was a UV-204, which was later modified and renamed the R.C.A. Radiotron UV-204A. It was R.C.A.’s largest transmitting triode available for commercial sale. R.C.A.’s first commercial line of Radiotron tubes were actually developed and manufactured by General Electric and Westinghouse, under cross licensing agreements tied to R.C.A.’s initial formation in 1919 by GE, Westinghouse, American Marconi and others. Each company manufactured two tubes of the four tube series. The R.C.A. UV-204 made by GE was exhibited at the October 1921 Electrical Show in New York City as part of R.C.A.’s large and popular display of its entire tube series. The UV204 featured prominently in R.C.A.’s very first catalogue, published in 1921, that highlighted the use of Radiotron tubes in experimental CW telegraph and telephone sets. The UV-204A became widely available to the general public in the summer of 1923. The maximum permissible anode voltage was 3,000V, maximum anode dissipation 250W, and the maximum anode current was 275mA. The R.C.A. UV-204 and UV-204A were notable in that they could be operated in a vertical or horizontal position and they had exceptionally long operating lives. The UV-204 continued in production until mid-1926. The UV-204A was so successful that it was still in production in 1931. American Marconi’s newly built station in Chatham, Massachusetts opened in 1921 and employed tube transmitters. The following year, R.C.A. began using tube transmitters for service from the U.S. to England and Germany. R.C.A. also began substituting tube transmitters on ships to replace spark sets and began replacement of crystal receivers by tube receivers on ships. Tubes remained dominant into the 1960s, when they began to be replaced by transistors, which arguably miniaturized the basic principles of tube design and operation, but at the molecular level. The 1921 Transatlantic Tests’ demonstration of tubes demonstrated that they were the option of the future for generating efficient long distance communication.
EVEN FURTHER DISTANCES ACHIEVED
The development of vacuum-tube equipment and new circuits helped greatly advance amateur radio. A March 1921 QST article, “Progress,” reviewed how over the previous nine years the transmitting range covered by amateur Ralph H. G. Mathews in Chicago, Illinois had increased from only 4 to nearly 3,000 miles. Far more sensitive regenerative and superheterodyne radios began supplanting crystal detectors, even while vacuum-tube CW transmitters began replacing spark based communications. Together, these developments in the efficiency of radio communications achieved staggering improvements in the functionality of radios. Coincidentally with the 1921 Transatlantic Tests, American efforts to span the Pacific were also underway. On January 18, 1922, A. H. Babcock in Berkeley, California (6ZAF) and Clifford J. Dow in Maui, Hawaii (6ZAC) engaged in two-way radio traffic across the Pacific. The British were also reaching for distance on the short waves. In October 1924, Ernest Simmonds (2OD) and then Cecil Goyder (2SZ) were heard in New Zealand, and a month later Ernest Simmonds made contact with the Australian station 3BQ. With these successes, many stations started to make contacts all over the world at shorter and shorter wavelengths. It was soon discovered that long distance contacts could also be made during the day, not just at night; and as a result, the first transatlantic daytime contacts were made in February 1925. Finally, in 1926, Brandon Wentworth, 6OI, worked all continents from his station in California for the first time.
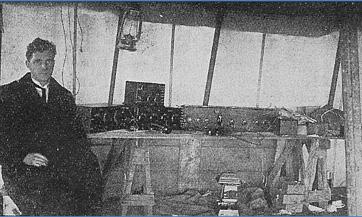
Receiving tent at Ardrossan, Scotland with Marconi Inspector D. E. Pearson. Table includes Baldwin headphones, General Radio Company precision wave-meter, Burgess batteries, an A.P. amplifier tubes, RCA U.V. 200 vacuum tubes, Paragon superheterdyne and regenerative receivers. (Courtesy QST, Feb 1922)
EVER SHORTER WAVELENGTHS
The 1921 Transatlantic Tests showed the viability of using shortwaves to span distance. Experimentation to find strategies that employed different antenna systems and the quest for lower power alternatives exploded after 1921. The free access to wavelengths lower than 200 meters allowed entrepreneurs a wide open space to experiment. Boyd Phelps encouraged amateur experimenters to try ever shorter wavelengths in his March 1922 article in QST, “Radio Below 200 Meters.” Soon, he was operating tests with J. C. Ramsey (1XA) and Frank Conrad (8XK later known as KDKA) to achieve good signals down to 100 meters. In 1923, KDKA, in addition to its normal operation on 360 meters, began transmitting on shortwave wavelengths from 80 to 100 meters. In 1924, Marconi discovered that shortwave transmissions of 30 meters could be heard in daylight and at night around the world, marking the often recognized beginnings of skywave transmission. In the subsequent decades, broadcasting over ever shorter wavelengths became viable, right down to the present day as research surrounding the use of
HIGH POWER STATIONS AND LONG WAVES
The concept of using high power to drive long distance radio transmission had not changed since Marconi’s own efforts to transmit cross the Atlantic. In 1901 and 1902, Marconi built high-power wireless stations in Poldhu, Cornwall, England; Glace Bay, Nova Scotia; and South Wellfleet, Cape Cod, to compete with transatlantic undersea cable service. Twenty years later, and following a World War, the basic approach remained the same, build big antennas and use more power. Following the war, while the amateurs were now restricted to operating below 200 meters and searched for low power alternatives to drive transmission and reception, government and commercial interests continued investing in high power stations. The U.S. government already operated several high power stations, starting just before World War I and continuing into the 1940s. The most well-known of these was NSS operated by the U.S. Navy from 1913-1941 at Arlington, Virginia, which was opened to establish reliable long distance radio communication. In 1921, American Marconi, which soon came under the direction of R.C.A., built the largest shipto-shore radiotelegraph station in the U.S. in Chatham, Massachusetts. Between 1920 and 1923, R.C.A. opened high power stations at Radio Central at Rocky Point on Long Island, New York; Tuckerton, New Jersey; and Warsaw, Poland. Each operated with 200kW Alexanderson alternators, used Beverage wave antennas, transferred their signals over private wire lines, and used ink recorders for reception of wireless telegraph signals. R.C.A. had plans to open stations in six other countries in Europe and South America. High power stations were also being constructed by others. The development of radio telephony, expansion of smaller stations, and growth of broadcast radio in the early 1920s all posed risks that demand could shrink for high power services at these stations when shorter distance radio communication was involved. The rapid growth of broadcast radio, especially in the U.S., also shifted demand toward consumer and related amateur applications. Nevertheless, the high power stations did communicate at distances of up to 4,000 miles. Even so, the Transatlantic Tests of 1921 foretold a future where low-power, physically smaller equipment would eventually be developed that would replace these giant transmitters, and shortwave transmission would replace the long wave technologies. Longwave radio does survive and is still used today because it can diffract over obstacles like mountain ranges and travel beyond the horizon, following the contour of the Earth. This mode of propagation, called ground wave, can be received up to 1,200 miles from the transmitting antenna. Very low frequency waves, below 30 kHz, can be used to communicate at transcontinental distances, and can penetrate saltwater to depths of hundreds of feet. So, it is used by the military to communicate with submerged submarines. Low frequency waves can also occasionally travel long distances by reflecting from the ionosphere at distances exceeding 190 miles from the transmitting antenna. Long waves are used for non-directional beacons, time signals, submarine communication, low frequency experimental radio (LowFER), and some broadcasting notably in Europe, North Africa and Central Asia as well as some amateur radio operations. Today, most modern radio systems and devices use wavelengths which would have been considered ultra-short and were not well known in 1921, but the Transatlantic Tests helped pave the way for this future development.
ANTENNAS
The 1921 Transatlantic Tests succeeded in no small part due to the use of a Beverage wave antenna in Ardrossan, Scotland. It had been invented earlier that year and was first published by Harold Beverage in the November 1922 issue of QST. The 1921 tests provided a unique opportunity to demonstrate the superior performance of
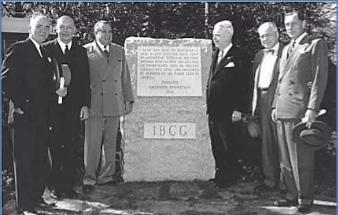
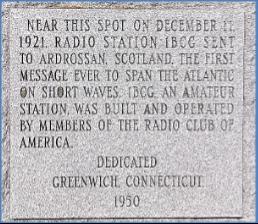
1950 memorial dedication in Greenwich. (L-R): Paul F. Godley, Maj. Edwin H. Armstrong, George E. Burghard, Wilbur A. Peck and Dr. Caldwell (both of Greenwich) and Ernest V. Amy.
this new antenna design, and an opportunity to publicize its success. A Beverage antenna consists of a horizontal wire from one-half to several wavelengths long (tens to hundreds of meters at HF to several kilometers for longwave) suspended above the ground, with the feedline to the receiver attached to one end, and the other end of the Beverage terminated through a resistor to ground. The antenna has a unidirectional radiation pattern with the main lobe of the pattern at a shallow angle into the sky off the resistor-terminated end, making it ideal for reception of long distance skywave (skip) transmissions from stations over the horizon that reflect off the ionosphere. However, the antenna must be built so the wire points at the location of the transmitter. The skip effect explains why the power of the transmitter for shorter wavelengths is less important than the distance to the receiver. The advantages of the Beverage antenna include excellent directivity and a wider bandwidth than resonant antennas. Its disadvantages are its physical size, requiring considerable land area, and inability to rotate to change the direction of reception. Installations often use multiple antennas to provide wide azimuth coverage. In 1921, Beverage was granted a patent for his antenna. Beverage long-wave receiving antennas up to nine miles long were installed at R.C.A.’s Riverhead, New York; Belfast, Maine; Belmar, New Jersey; and Chatham, Massachusetts receiver stations for transatlantic radiotelegraphy traffic. AT&T built what may be the largest Beverage antenna ever constructed, an array of four phased Beverages three miles long and two miles wide, in Houlton, Maine, for the first transatlantic telephone system opened in 1927. Exploration of antenna designs as the critical component of radio transmission and reception continued after the 1921 tests. The central question of how to match the frequency of the antenna’s capabilities, related closely to its length, to the transmitted wavelength was not overcome until the emergence of fractal antennas beginning in 1988. Fractal antennas are based on a different approach that maximizes the effective length by increasing the perimeter of material that can receive or transmit electromagnetic radiation within a given total surface area or volume. This new approach permits a considerable reduction in antenna length, and is now widely used in counterpoises, loads, ground planes, and filters, all parts that can be integrated with antennas, and which are used in television, cell phones and other applications. But, even Fractal antennas can trace their heritage to the legacy of Beverage’s work.
IMPROVED CIRCUITRY OPENS THE DOOR
Edwin H. Armstrong’s regenerative circuit dated from 1912 and was already being widely used by 1920. His superheterodyne circuit traced its roots to 1918 and was not well known, but the adaptations employed in the 1921 Transatlantic Tests and its success brought early, wide recognition. Its superiority of performance over other forms of receiver was soon unquestioned. It not only provided improved sensitivity and selectivity, but greatly simplified the external interactions with the circuits that were necessary for reception. The superheterodyne circuit standardized and built in the intermediate frequency within the radio set, reducing the need to tune only the desired frequency to match the amplifier. This approach enabled the general public to operate far simpler radio reception equipment. Manufacturers responded by producing radios with fewer knobs and adjustments, which brought results quicker and easier, making radio more appealing to the non-technical enthusiast. This opened the door to a huge potential audience who could participate in radio, once programming and other on-air content developed. R.C.A. soon brought out its first commercial superheterodyne receiver in 1924; and in 1925, the same year the electrodynamic speaker became available, R.C.A. brought out a receiver capable of operating from alternating current. All of these depended on advances
in receiver circuitry and in tube designs, which parlayed Armstrong’s original concepts for receivers into commercial products that were available and useable by the general public.
EVER SMALLER CONSUMER RADIOS
The use of the regenerative and superheterodyne circuits, the incorporation of ever more efficient radio tubes/valves, and the ability to reduce the size and complexity of the entire radio system launched a quest to make ever smaller and more portable options available to the radio consumer. Lower power and easier means of reception opened the door to a century of development. After 1921, the radio consumer would witness reductions in the physical size, power requirements, and cost of radios, making them more affordable and attainable. Radios transformed from room sized technological agglomerations of equipment operated by technical hobbyists to consumer products that evolved from floor-furniture pieces, to table tops, to small portable radios. Decades of development followed, all based on the initial premise from 1921: that size and power could be minimized without sacrificing function.
OPENING OF THE SPECTRUM
Prior to 1921, the spectrum below 200 meters was considered a wasteland, suitable only for amateurs to enjoy. But the success of the 1921 Transatlantic Tests set the table for many later technological developments in shortwave radio. Pittsburgh station KDKA was among the leaders in developing shortwave broadcasting early on. These bands of the spectrum enjoyed boom years in the Second World War and in the Cold War Era, with the British Broadcasting Corporation (BBC), Voice of America (VOA), and Radio Free Europe/Radio Liberty (RFE/RL) as well as Soviet/Russian and Chinese stations all among the biggest broadcasters of that era. They are still used in international government broadcasting and by private domestic stations. Today, air traffic control, utility stations not intended for the general public, amateur radio, time-signal and radio clock stations, satellite radio and TV, Digital Radio Mondiale (DRM), and some mobile platforms all use the shorter wavelengths. Other sporadic or non-traditional users of the shortwave bands include clandestine and numbers stations, unlicensed two way radio activity, pirate radio broadcasting, over-the-horizon radar, and ionospheric heaters used for scientific experimentation. Even smaller microwaves, with higher throughput for data upload and download rates than radio waves, can transmit more data, but are more limited in range and can be disrupted by solid objects. So, microwaves are often used for radar and satellite communications. Would any of this have been possible without the success of the 1921 experiments that proved the viability of the shortwave spectrum bands?
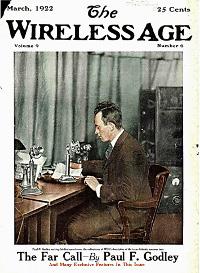
Cover of The Wireless Age (March, 1922) Paul Godley making his first speech over the radiophone at WDY, describing the Transatlantic Tests.
FUTURE DIRECTIONS
Long distance communication in 1920 was dominated by expensive and complex longwave radio transmitters and submarine telegraph cable. A transatlantic submarine cable was twice the cost of two longwave stations. Even so, cable operators remained largely unthreatened by competition from radio because cables were more reliable, worked equally well both day and night, were not affected by storms, and competition from radio actually stimulated innovations in cable technology. Shortwave radio under 200 meters was considered to have no practical value and was left to amateurs for experimentation. For 25 years, long distance radio evolved by increasing the size, complexity and cost of equipment. By 1924, the British Marconi Company considered its longwave stations to be obsolete. It offered to build a chain of shortwave stations across the British Empire that would cost only five percent of a comparable longwave chain and would use only twenty percent of the power but would transmit at three times the speed. Shortwave was clearly the future. In October 1926, Marconi gave a speech to the IRE and concluded, “I admit that I am responsible for the adoption of long waves for long-distance communication. Everyone followed me in building stations hundreds of times more powerful than would have been necessary had short waves been used. Now I have realized my mistake…” By the following year, shortwave radio had captured half the world’s intercontinental cable traffic.
The 1921 Transatlantic Tests represent the triumph of newly invented radio and antenna circuitry, the advent of more sophisticated vacuum tubes, and the beginning of a century of development toward ever smaller, less expensive and more widely available electrical communication technologies based on ever shorter wavelengths of the radio spectrum. It demonstrates that major contributions are made by independent inventors and experimenters. For the next 100 years, experimenters and inventors sought to continually improve wireless technologies and devices. That progress and invention initially brought the expansion of radio telephony and eventually spawned the smart phones, smart watches, smart TVs, wireless internet routers, GPS tracking devices, and Bluetooth headsets that we use today, all of which depend on wireless technologies. In many ways, the 1921 tests represent the beginnings of the modern radio and wireless era, moving electrical communications beyond wireless telegraphy and toward the many applications found today in everyday use. The Transatlantic Tests were widely heralded at the time in the amateur and professional as well as the general press, and they helped mark the dawn of an era that witnessed explosive growth and new advances in wireless telegraphy, two-way radio, and commercial broadcasting. The decades that followed brought the commercialization of this new form of communication. Indeed, the legacy of 1921 continues into the 21st century.
SOURCES
Anderson, J. M., Supplying Tubes, Sets, and People to RCA: The General Electric Connection, AWA Review, Vol. 5, 1990, p. 1. Armstrong, E. H., “The Superheterodyne: Its Origin, Development and Some Recent Improvements,” Presented Before the Institute of Radio Engineers, Mar. 5, 1924, Proceedings of the IRE, Vol. 12, No. 5, Oct. 1924. Bartlett, R. A., The World of Ham Radio 1901-1950: A Social History, (Jefferson, NC: McFarland and Company, 2007). Beverage, H. H., The Wave Antenna for 200-Meter Reception, QST, Nov. 1922, p. 7. Boucheron, P., “At the Sending End of Radio: Spanning the Atlantic with Fifty Watts of Electrical Energy and a Few Facts Regarding CW Transmitters,” Scientific American, April 1922, p. 232-233. Burghard, G. E., “Station 1BCG,” Proceedings of the Radio Club of America, Vol. 2, No. 2, Feb. 1922. DeSoto, C. B., Two Hundred Meters and Down: The Story of Amateur Radio, (West Hartford, CT, American Radio Relay League, 1936). Douglas, S. J., Inventing American Broadcasting, 18991922, (Baltimore: Johns Hopkins University Press, 1987). First Atlantic Amateur Radio Contacts, Electronics Notes Website, https://bit.ly/3b7bJI6, accessed Aug. 30, 2021. Headrick, D. R., Shortwave Radio and Its Impact on International Telecommunications Between the Wars, History and Technology an International Journal, 1994, 11:1, 21-32, DOI: 10.1080/07341519408581852. Radio Apparatus for Amateur and Experimental Use With Instructions for Continuous Wave Operation, Radio Corporation of America, Sep. 1, 1921. Radiotraon Model UV-204A 250-Watt Output, Instructions J-84 Edition A, Radio Corporation of America, Jan. 1927. Record of the Development of Wireless Telegraphy, The Yearbook of Wireless Telegraphy, (New York: The Wireless Press, for years 1921, 1922, 1923). The Story of the First Trans-Atlantic Short Wave Message, 1BCG Special Issue: Proceedings of the Radio Club of America, Oct. 1950. To Be Where The Audience Is: Report of the Special Committee on the Future of Shortwave Broadcasting, Board of Broadcasting Governors, Aug. 2014. Tyne, G.F.J., Saga of the Vacuum Tube, (Berkeley Heights, NJ: Prompt Publications, 1994). Warner, J. C., “Part I-The Years to 1938,” RCA: A Historical Perspective, Radio Corporation of America, 1977. Westman, H. P., Radio Pioneers 1945, New York Section of the Institute of Radio Engineers, 1945. Wireless For Everybody, World Wide Wireless, Radio Corporation of America, Vol. 2, Nov. 1921, p. 5.
ABOUT THE AUTHORS
David Bart is Vice President of the Radio Club of America and a Fellow, Director, and Life Member of both RCA and the Antique Wireless Association as well as chairman of the RCA Publications Committee. He is a Life Member of ARRL, the former treasurer of the IEEE History Committee, and Vice President of the Museum of Broadcast Communications in Chicago. Julia Bart is an advisor to and a fellow of the AWA, a former officer of the Antique Radio Club of Illinois, and coauthor of numerous articles with David.
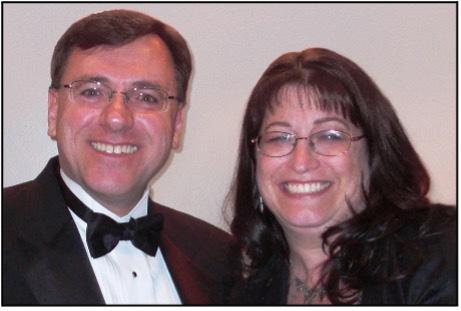