A
Functional Medicine Perspective
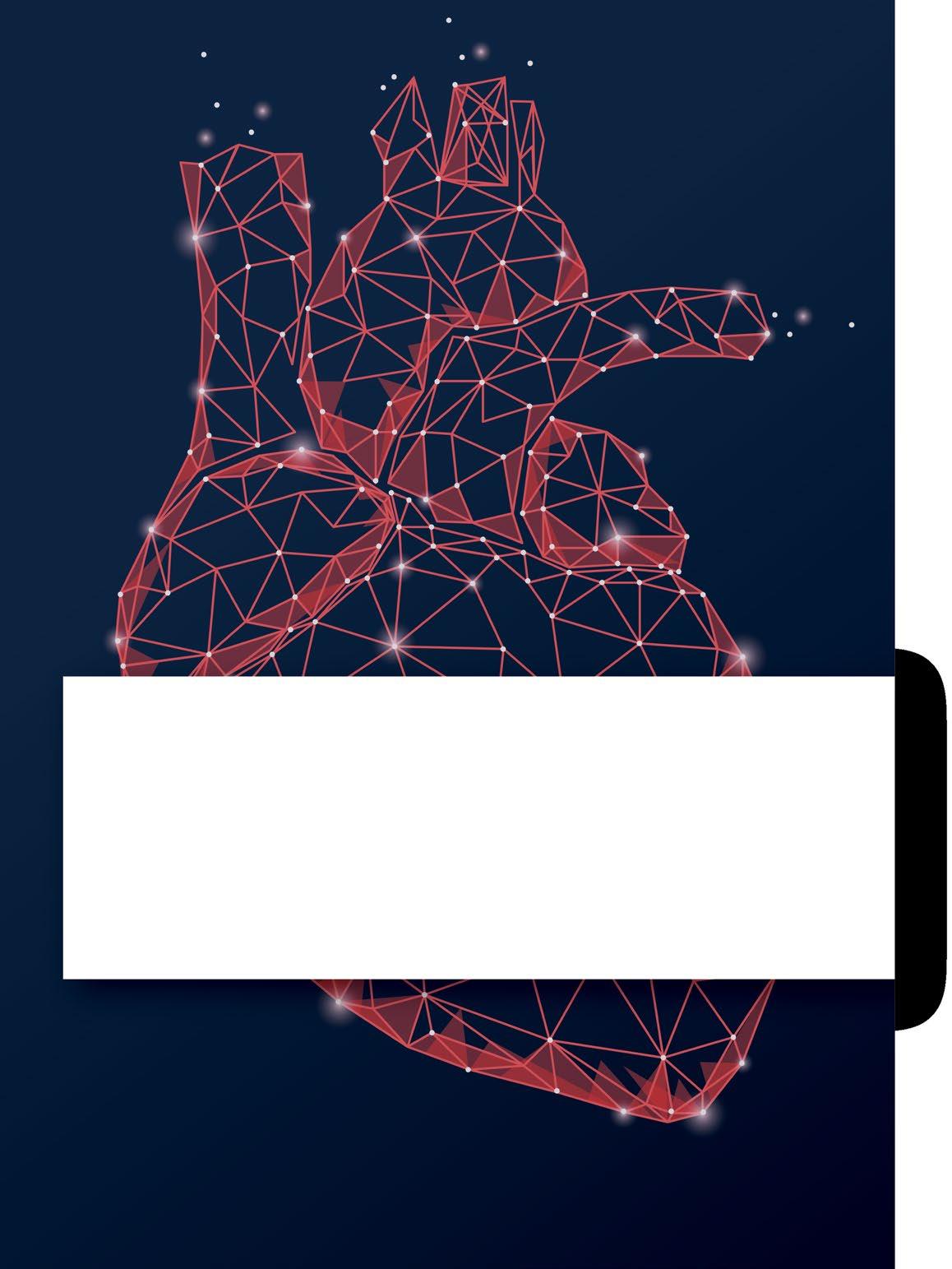
Modern Approaches to Cardiovascular Health
A Functional Medicine Perspective
By: Dr. Cory Ambrose, ND, MAT
Danielle Moyer Male, MS, CNS, LDN
Caitlin Higgins, MS, CNS
Key Learning Objectives
• Review cardiovascular anatomy and physiology, and delve into the fundamental pathophysiology of cardiovascular illnesses related to inflammation, oxidative stress, nutritional status, and endothelial health.
• Investigate the latest research highlighting the significance of nitric oxide in cardiovascular health and prevention.
• Learn strategic methods for recommending micronutrients, diet, and lifestyle adjustments to support cardiovascular health, aligning with the latest research findings.
• Explore contemporary research methodologies in cardiovascular health, identifying potential gaps in the current research landscape.
From a functional medicine perspective, many cardiovascular (CV) risk factors can be addressed, even minimized, through measures relating to prevention. This document aims to provide tools and empower the practitioner to suggest highly‑researched micronutrients to support CV function and discuss the latest evidence‑based functional medicine findings.
This information is provided as a medical and scientific educational resource for the use of physicians and other licensed health‑care practitioners. This information is not intended for use by consumers.
Dosing recommendations are given for typical use based on an average 150-pound healthy adult. Health-care practitioners are encouraged to use clinical judgement with case-specific dosing based on intended goals, subject body weight, medical history, and concomitant medication and supplement usage.
About the Authors
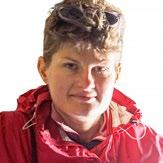
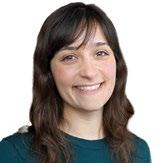
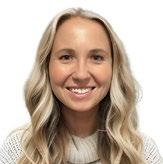
Dr. Cory Ambrose, ND, MAT is a naturopathic doctor, educator, speaker, and clinical science/medical writer. They are a proud alum of New York University, Relay GSE, and Bastyr University. Recognized for their award-winning content creation, Dr. Ambrose stands at the forefront of bridging crucial data gaps in functional medicine, botanical research, and underserved populations.
Danielle Moyer Male, MS, CNS, LDN is a Board-Certified Nutrition Specialist (CNS®) and a Licensed Dietitian Nutritionist (LDN). She holds an M.S. in Human Nutrition from the University of Bridgeport and a B.A. in Anthropology from Hobart and William Smith Colleges. Danielle serves as a nutrition instructor at the collegiate level and has been a clinical science writer for Designs for Health since 2022.
Caitlin Higgins, MS, CNS, a Board-certified Nutrition Specialist® and Senior Clinical Science Writer at Designs for Health, holds a Master’s degree in Clinical Nutrition from Maryland University of Integrative Health. Based in Atlanta, GA, she uses her expertise as a functional nutritionist and Reiki master practitioner to empower clients in reclaiming their wellness, joy, and alignment.
Introduction
Cardiovascular disease (CVD) is the leading cause of mortality worldwide.1 The Global Burden of Disease Study found that 50% of all global deaths (collectively) could be attributed to: hypertension, smoking, ischemic heart disease, and cerebrovascular disease.2 A population study reported the overall prevalence of cardiovascular diseases in adults over the age of 20 to be 48.6%.3 Cardiovascular diseases, along with major risk factors like smoking, are significant contributors to premature mortality and reduced quality of life due to severe disability.2 While the etiology of each cardiovascular disease varies, many share common origins.
These common origins can be categorized into two main groups: modifiable and non‑modifiable factors. Modifiable factors encompass (but are certainly not limited to) chronic inflammation, oxidative stress, and alterations in lipid and glucose metabolism. This document will specifically focus on the influence of nutritional status, exercise, micronutrients, and the microbiome on these modifiable origins of cardiovascular disease.
From a functional medicine perspective, many cardiovascular risk factors can be addressed, even minimized, through risk factor prevention. This document aims to empower and equip practitioners with tools to recommend evidence‑based micronutrients and nutraceuticals for cardiovascular support and discuss the latest scientific findings in functional medicine. Certain non‑modifiable cardiovascular illnesses fall outside the scope of this document. However, with ongoing functional medicine research, we anticipate addressing more conditions over time.
Understanding the science of modifiable cardiovascular illnesses and the latest research in helping to optimize cellular health and cardiovascular function may promote CV disease prevention and help your patients live richer, fuller lives.
CARDIOVASCULAR DISEASE ORIGINS
Factors that influence modifiable
CV Disease origins:
• Nutritional status
• Physical activity
• Diet/Lifestyle
• Phytonutrients
• Vitamins/Minerals
• Microbiome
• Nitric oxide status
• Oxidative stress
• Chronic inflammation
• Endothelial health
• Lipid metabolism
• Cellular energy
• Blood glucose balance
• Microbial health
Genetic etiologies
Epigenetics
Concurrent illnesses, socioeconomic status, and other related factors fall between modifiable and non-modifiable.
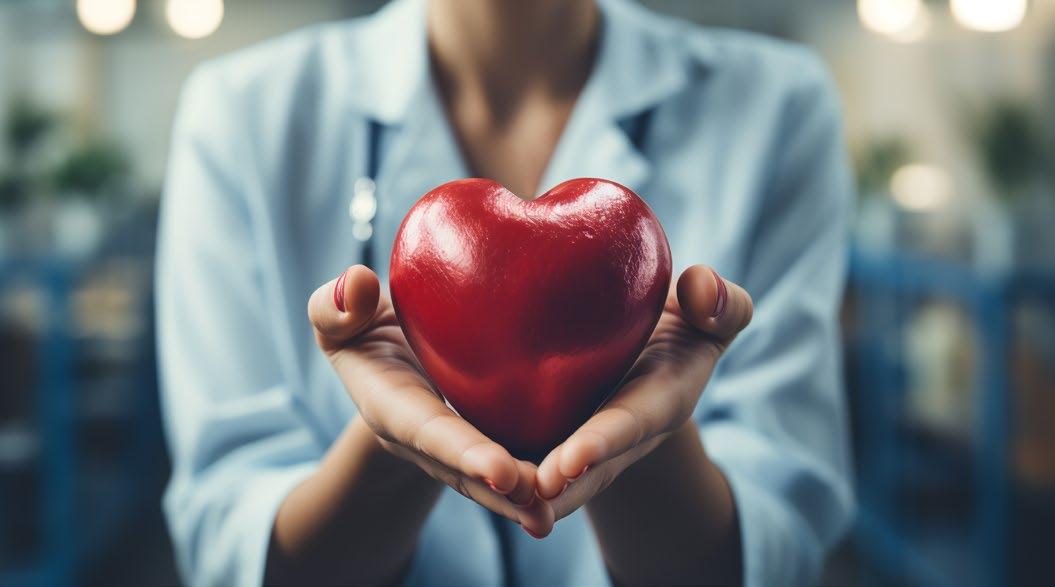
Modifiable Factors that Influence Cardiovascular Health
The purpose of this section is to briefly review and highlight the key physiological processes relevant to later discussion. For a more foundational review of anatomy and physiology, we encourage the reader to register for the accredited Dietary Supplement Specialist™ Certification Program by Designs for Health.
Oxidative stress
Oxidative stress is linked to the etiologies of many chronic illnesses throughout the body; this includes atherosclerosis and many other cardiovascular diseases.2,4–6 Oxidative stress is caused by an overaccumulation of free radical reactive species. Of these, reactive oxygen species (ROS) have been a primary focus in research. The main ROS agents include hydrogen peroxide (H2O2), superoxide anion O2•–, and hydroxyl free radical (•OH). By nature, ROS are unstable molecules, and either exist as free radicals or break down easily into free radicals.7 In addition, reactive nitrogen species are another class of molecules that are also involved in similar cellular damage and oxidative stress‑related processes.7 These relate to the nitric oxide (NO) pathways covered later in this document, potentially causing reductions in the availability of NO.
ROS are normal byproducts of many fundamental biochemical processes within the cell, including mitochondrial metabolism and the activity of many enzymes, most notably the NADPH oxidase (NOX) family of enzymes.4 The NOX group of enzymes produce ROS and have been shown to trigger inflammation. In turn, ROS‑induced inflammation further influences the activity of NOXs.4 NOX also participates in crosstalk between mitochondria and enzymes
related to the nitric oxide pathways,4 discussed in more detail in the section in this document on nitric oxide status. In addition, ROS play some other roles within the body; for instance, hydrogen peroxide participates as a second messenger in some cell signaling pathways.7 While ROS are important byproducts of normal cellular function, imbalances in ROS cause deleterious changes to many parts of the body. This includes the induction of damage to the vascular endothelium, resulting in atherosclerotic changes.4 Increased levels of ROS cause the oxidation of certain proteins which results in the secretion of inflammatory mediators.4 Oxidative stress is linked to the inflammatory process through signaling molecules such as peroxiredoxin‑2.4 Oxidative stress can also induce the activity of nuclear factor‑kB (NF‑kB),4 an inflammatory mediator which will be discussed more in later sections of this document.
The mitochondria in endothelial cells are particularly vulnerable to oxidative stress; downstream effects include mitochondrial dysfunction and ROS‑induced endothelial dysfunction.4 ROS also cause damage to the mitochondrial electron transport chain and can disrupt calcium homeostasis. In addition, ROS cause an increase in outer mitochondrial membrane permeability, which can further induce the inflammatory process.4
The body contains a complex system to control the balance of ROS. This system requires molecules, enzymes, and specific nutrients that help support antioxidative status in cells and tissues, such as vitamin C, vitamin E, and glutathione.8
The glutathione system
Glutathione (GSH) is considered the body’s “master” antioxidant and is critical for the maintenance of antioxidative balance and helping protect cells from oxidative stress. Deficiencies in glutathione have been linked to hypertension and many other chronic illnesses.8
The glutathione system consists of glutathione, several enzymes, and other molecules that support many biological pathways including antioxidation, phase II detoxification conjugation, and the pentose phosphate pathway. Glutathione acts as a powerful antioxidant in two main reactions: 1) Glutathione directly neutralizes free radicals. 2) The glutathione redox reaction where hydrogen peroxide (H2O2) is converted to water. This reaction involves two glutathione molecules in a reduced state becoming oxidized to glutathione disulfide by the selenium‑containing enzyme glutathione peroxidase. Glutathione disulfide can then be recycled back to glutathione by the enzyme glutathione reductase.9
A critical precursor to glutathione is N‑acetyl‑L‑cysteine (NAC), which helps support antioxidative status in the body by helping to increase GSH levels and inactivate ROS.10,11
Other antioxidative pathways
Outside of the glutathione system, other important molecules help support antioxidative status and are often measured and quantified in research. These include the enzyme superoxide dismutase (SOD), which helps transform the superoxide anion into more stable molecules. SOD is thought in some literature to be an overall reflection of antioxidative status.2
Lipid peroxidation is a pathway involving increased free radical production and oxidative stress. Malondialdehyde (MDA) is a measurable product of lipid peroxidation and is a
common marker reported in research. Elevated MDA levels have been linked to certain chronic illnesses.12,13
Nuclear factor erythroid 2‑related factor 2 (Nrf2) is a transcription factor associated with over 200 genes. It helps to modulate antioxidative metabolism, support a healthy inflammatory response, and preserve homeostasis in the presence of cellular stress.14 It helps regulate glutathione S‑transferase and helps modulate many aspects of antioxidative status.15 Several phytonutrients covered later in this document help support the Nrf2 pathway, including resveratrol and pterostilbene.16
Major risk factors for CVD, including smoking and diabetes, increase the generation of ROS and thus increase oxidative damage.4 Evidence suggests that the administration of antioxidants or the attenuation of ROS generation may help mitigate the damage caused by ROS in the vascular endothelium.4 Many micronutrients discussed in this document help support antioxidative status and optimal endothelial function.
Inflammation
Chronic inflammation is associated in literature with the development and progression of many cardiovascular‑related pathologies.17 During the process of endothelial cell damage, oxidative stress and inflammatory processes are interconnected,4 and the activity of ROS is linked to many different inflammatory pathways and molecules that are highlighted below. During the inflammatory process, endothelial cells undergo major changes, which include:4
• Increased expression of pro inflammatory mediators
• Increased permeability of plasma proteins into the vasculature
• Activation and increased prevalence of adhesion molecules
Pro‑inflammatory mediators
The NF‑κB pathway is one of the most important elements in the discussion on the inflammatory response. ROS oxidize the IκB kinase (IKK) complex, which causes NF‑κB activation.4 Hydrogen peroxide can also directly increase the activity of NF‑κB through translocation to the nucleus.4 After activation, NF‑κB targets molecules associated with endothelial cell dysfunction.4 These include the pro inflammatory cytokines tumor necrosis factor‑α (TNF‑α), interleukin (IL)‑1β, and IL‑6; these in particular are often involved in CVDs and certain metabolic‑related illnesses.4,17 TNF‑α, IL‑1β, and IL‑6 have also been linked to endothelial monocyte infiltration and insulin resistance.4
NF‑κB also activates cell adhesion proteins including vascular cell and intracellular adhesion molecules 1 (VCAM 1 and ICAM 1).4 Research suggests that molecules and enzymes such as SOD2 can prevent the activity of NF‑κB.4 Many micronutrients covered in this document help support the modulation of NF‑κB, the inflammatory response, and oxidative stress.
Additionally, several single nucleotide polymorphisms (SNPs) located in or near genes are linked to atherosclerosis, leukocyte migration, cellular adhesion, and inflammation. These have been associated with inflammation of the arterial wall and the overall development of certain CVDs.2
Inflammation, senescence, and CV function
Aging is a contributing factor to the development of cardiovascular diseases. In addition, CVD is a leading cause of morbidity and mortality in older adults.2 This is thought to be due to increased oxidative stress‑induced endothelial dysfunction and inflammation.2
Certain inflammatory markers associated with CVD have been observed in elevated concentrations in elderly populations. These include IL‑6, C‑reactive protein (CRP), fibrinogen, soluble VCAM 1, and monocyte chemoattractant protein‑1 (MCP‑1), an adhesion molecule responsible for leukocyte recruitment to vascular tissues.2 Age‑associated frailty has been linked to inflammation, as measured in elevated CRP and IL‑6.2
Additionally, immunosenescence has been linked to both chronic inflammation and the development of many illnesses, including certain CVDs.2 Immunosenescence is a term describing the changes that occur to the immune system during the aging process.18 This may involve altered inflammatory and metabolic processes, reductions in T cell activity, and increased susceptibility to infectious disease. Immunosenescence impacts many immune functions, which can lead to the decline of B lymphocytes, reduced diversity in T‑cell receptors, and a weakened production of immune cells from the bone marrow. The function of neutrophils, macrophages, and natural killer (NK) cells can also become impaired.19
Macrophages are important players in immune function and the inflammatory response. More specifically, M1 macrophages are involved in the inflammatory response and secrete cytokines such as IL‑6, IL‑1β, and TNF‑α.20 M2 macrophages secrete cytokines related to osteogenesis and angiogenesis.20 Macrophages can switch from M1 to M2 phenotype under certain conditions.20 Delays in the macrophage phenotype switch can be caused by mitochondrial dysfunction and endoplasmic reticulum stress.20 Certain botanicals covered in this document such as resveratrol may support healthy levels of IL‑1β and NF‑κB, biomarkers associated with M1 macrophages. Because of this, resveratrol may help support the M1 to M2 phenotypic switch.20
On a broader level, cellular senescence contributes to age‑related illnesses such as CVD and has become a significant focus in recent research. Studies suggest that even a relatively small quantity of senescent cells (SCs) may cause tissue dysfunction.21 While rare in younger individuals, SCs increase in many tissues within the body during the aging process.21 Tissues containing SCs may be more vulnerable to disease onset and progression, increased inflammation, and decreased function over time.22 Certain micronutrients and botanicals, including resveratrol, can help support the body’s response to specific senescent cells, including senescent macrophages.20 Many micronutrients discussed in this document help support healthy aging, the inflammatory response, optimal cellular functioning, and cardiovascular health.
Nitric oxide status
Nitric oxide (NO) is a messenger molecule with numerous critical functions within the body. One of its primary functions is to serve as a vasodilator to promote increased blood supply to tissues and to relax the inner muscles of blood vessels, which promotes normal blood pressure and blood flow.23 Healthy NO status helps attenuate the atherosclerotic process by decreasing monocyte adhesion and atheroma formation.24 Clinical studies suggest that impaired NO production, recycling through the oral microbiome, bioavailability, and/or activity is associated with endothelial dysfunction in CVD, hypertension, and atherosclerosis.23,25–28 Additionally,
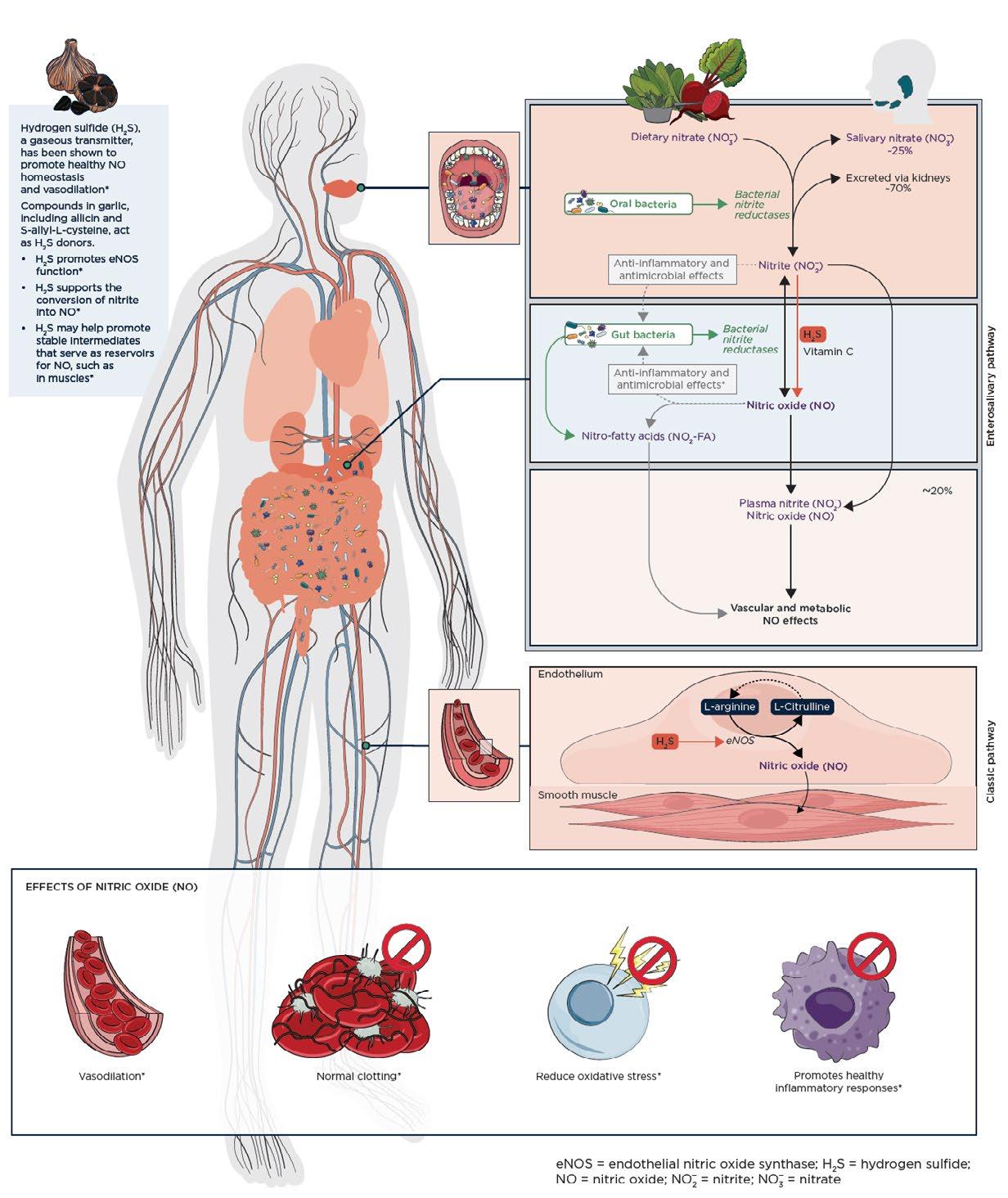
Pathways for Nitric Oxide Production
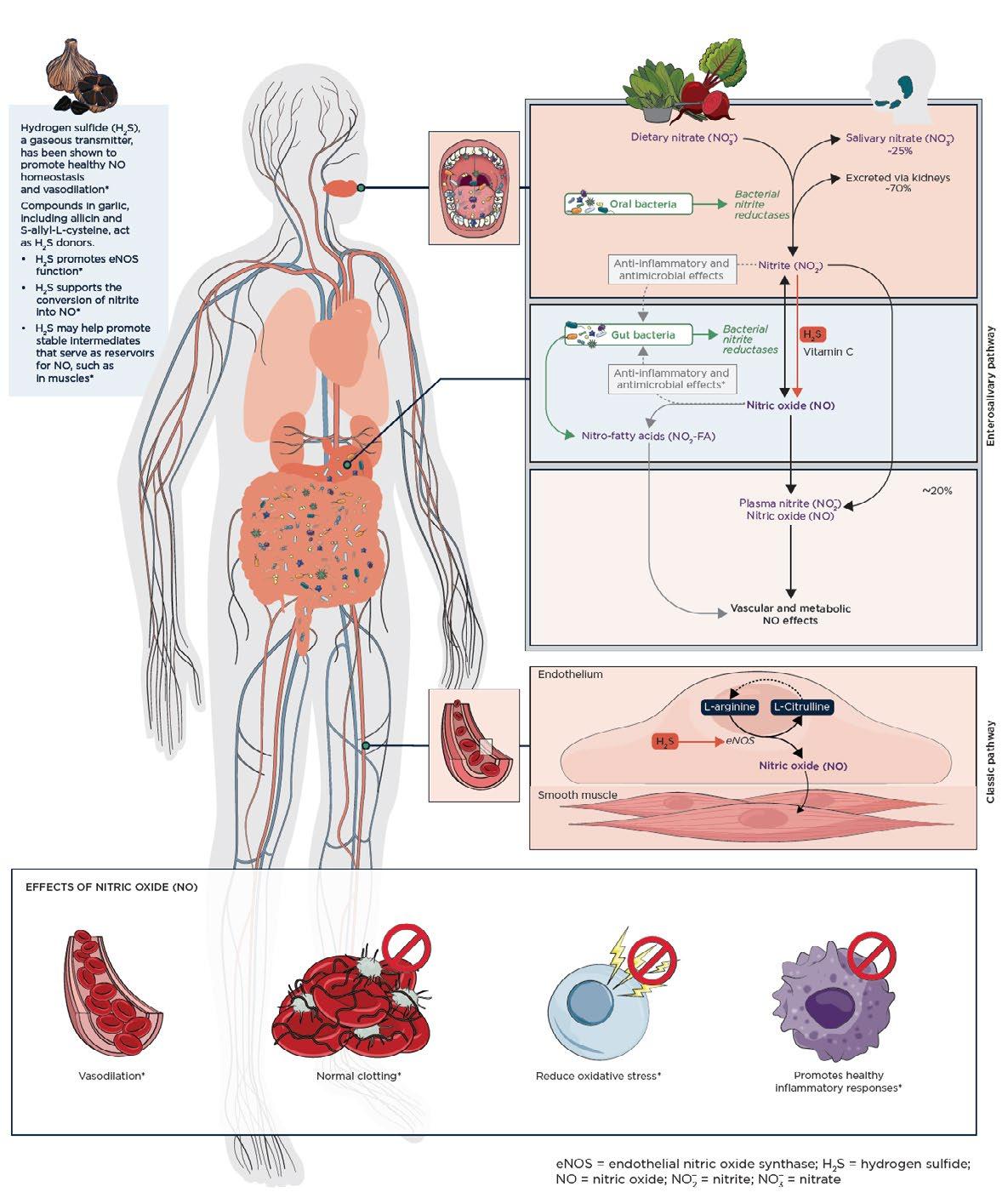
NO is a key factor for normal penile erectile function.29 Disorders that reduce NO synthesis or release in the erectile tissue are commonly associated with erectile dysfunction.30
There are two major pathways that work in tandem to maintain healthy NO homeostasis in the body: the endogenous pathway and the enterosalivary pathway. (See details in Figure 1.) NO is endogenously produced from L‑arginine, catalyzed by endothelial nitric oxide synthase (eNOS).23 This endogenous pathway activity may decline with age or in certain conditions due to decreased eNOS synthesis.25,27,28
The enterosalivary NO pathway begins with the digestion and rapid absorption of inorganic dietary nitrate (NO3 ) by the gastrointestinal tract. This nitrate then enters circulation and is either excreted through the kidneys (approximately 70%) or transported to the salivary glands (about 25%), where it forms salivary nitrate. Salivary NO3 is reduced to nitrite (NO2 ) by commensal oral bacteria. Swallowed saliva then delivers NO2 into the stomach, where it is reduced to NO by the acidic environment and in the presence of endogenous reductants, such as vitamin C and polyphenolic compounds.31–33 This will be discussed further in the section titled “Synergistic nitric oxide support.” The enterosalivary pathway is associated with elevations in plasma NO2‑ and cyclic guanosine monophosphate (cGMP), a sensitive marker of bioactive NO production.28 It is important to note that while normal NO status is necessary for cardiovascular health and blood pressure regulation, an overproduction of NO is associated with unregulated inflammatory responses.34,35
Endothelial health
Anatomy of the endothelium
Blood vessel walls contain a protective layer that separates the tissue from circulating blood called the endothelial glycocalyx (EGX); see details in Figure 2. The EGX is a thin gel‑like layer that acts as the first line of defense in vessel walls. Every blood vessel is lined with the EGX, which consists of a mesh of glycoproteins, proteoglycans, glycosaminoglycans, and plasma proteins.36
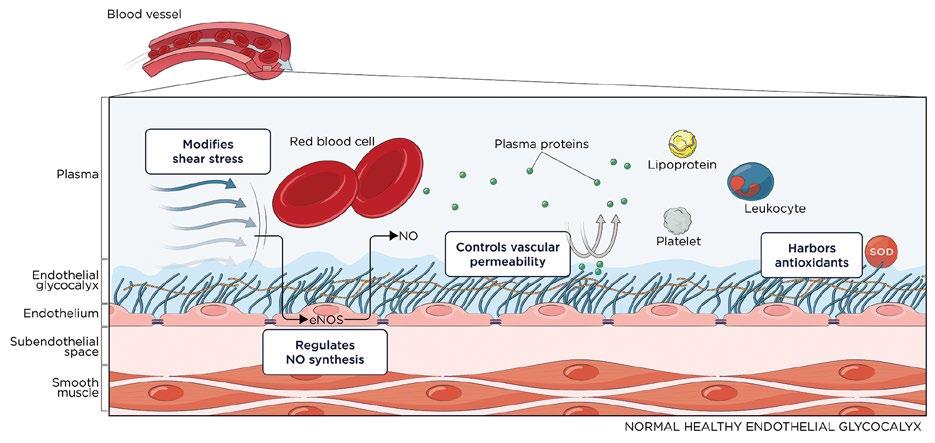
Damage to the EGX has been associated with aging, smoking, inflammation, and certain dietary changes. During the process of damage to the EGX, increased adhesion to vascular walls and subsequent arterial plaque development and remodeling may occur. 37
Endothelial damage and dysfunction
Endothelial dysfunction is caused by oxidative stress, increases in vascular ROS production, and vascular inflammation.4 Endothelial dysfunction further triggers a cascade of changes discussed in detail in this section (see details in Figure 3). These include: vasoconstriction, leukocyte infiltration, smooth muscle cell proliferation, and thrombocyte activation.2 Ultimately this results in physiological changes that have been linked to the development of atherosclerosis, hypertension, and other CV pathologies.2,4
One of the major causes of endothelial disruption is the imbalance of vasodilators.4 Nitric oxide, as discussed in a previous section, is a key vasodilator and is an integral component of endothelial health, vascular tone, and antioxidative status.4 ROS cause oxidation in arterial walls. This causes cellular damage and imbalances in intracellular redox homeostasis.4

Atherosclerotic changes
The underlying pathology behind many cardiovascular diseases is atherosclerosis.1 Atherosclerosis is a multifactorial process involving the thickening of blood vessel walls and a loss of vascular elasticity. During atherosclerosis, blood vessels collect plaques along the vascular wall. These plaques then build and can obstruct the blood vessel lumen.
Atherosclerosis is influenced by various factors, including chronic inflammation, oxidative stress, the transformation of macrophages into foam cells, plaque formation, and other deleterious alterations to the function of the vascular endothelium.1,4 In the progression of atherosclerotic changes, endothelial dysfunction is the earliest observable change.4 Endothelial dysfunction causes the leakage of low‑density lipoprotein (LDL) molecules into the sub‑endothelium (intima). This causes a molecular accumulation, which then becomes oxidized by ROS. LDL in its oxidized form then signals the production of cell adhesion molecules such as VCAM‑1 and ICAM‑1 by dysfunctional endothelial cells. Proinflammatory cytokines are then attracted to the sub‑endothelium, which causes the increase of monocytes, neutrophils, lymphocytes, and mast cells in arterial walls. Here, monocytes then mature into
macrophages, which engulf lipoprotein particles to form foam cells, thus resulting in the formation of atherosclerotic plaque.4,38
Atherosclerotic plaque buildup has two major effects: a narrowing of the blood vessel, and a reduction in elasticity. This results in obstruction of the lumen and an increase in pressure. This can in turn lead to inadequate supply of blood to an organ or part of the body. This process of plaque buildup and occlusion is a common occurrence in the coronary arteries. Atherosclerosclerotic plaques can also form blood clots, which may lead to a cardiovascular event such as a stroke.38
The science of hypertension
In order to circulate blood through the cardiovascular system and supply sufficient oxygen and nutrients to far‑reaching cells and tissues, an adequate amount of force is required. Blood pressure refers to the amount of pressure exerted against artery walls, and it occurs in two phases: systolic and diastolic. Systolic refers to the pressure that blood exerts on artery walls when the heart beats. Diastolic refers to the pressure in the arteries where the heart rests between beats.39,40
Hypertension is a chronic elevation in blood pressure. It typically occurs when arteries become narrower, due to atherosclerosis or vasoconstriction. High blood pressure forces cardiac muscle to pump harder and exert more effort for it to drive oxygenated blood to cells and tissues. This additional pressure can damage the endothelium. This endothelial damage drives the pathophysiology of many CV diseases.4,40,41 Nearly half of American adults have hypertension (48.1%), and only 1 in 4 adults have their hypertension under control (22.5%).39 The global incidence of hypertension is estimated to be 1.39 billion.42
The endothelial injury caused by hypertension can worsen the atherosclerotic process.41 Laboratory studies have observed increases in endothelial dysfunction in isolated arteries that have been exposed to high pressure.4 Even short‑term increases in blood pressure have been associated with increases in oxidative stress and further endothelial disruptions.4
The renin‑angiotensin‑aldosterone system (RAAS) is an important component to the physiology of hypertension. Research indicates that the activation of RAAS is associated with increases in endothelial dysfunction. Clinical and laboratory studies have observed a RAAS‑induced overproduction of superoxide through the activation of one of the NADPH oxidase isoforms.2
Modifiable contributing factors to the pathophysiology of hypertension will be discussed in detail in further sections. Briefly, these may include: sodium imbalances or other aspects of nutritional status, gut microbiome imbalances, stress, abnormal sympathetic responses, and toxin exposure.
Lipid metabolism
Cholesterol is important for many critical functions in our bodies. Cholesterol serves as a precursor for the synthesis of steroid hormones, bile acids, and vitamin D. It is also an essential structural component of cell membranes; cholesterol is required to maintain membrane integrity and fluidity of each cell.
Several types of cholesterol molecules exist and play different roles in the body. These are:
High‑density lipoprotein cholesterol (HDL‑c) carries cholesterol molecules away from arteries back to the liver, where it is broken down and eliminated from the body.43 However, HDL‑c does not completely eliminate potentially harmful cholesterol molecules; approximately 25% to 30% of blood cholesterol is carried by HDL‑c.
Low‑density lipoprotein cholesterol (LDL‑c) accounts for most of the cholesterol circulating in the body.43 There does exist a distinction between the various sizes of LDL‑c molecules and their corresponding actions in the body, but the vast majority of research covered in this document quantifies only LDL‑c and does not differentiate between its different molecules; therefore, only LDL‑c will be discussed here. Future functional medicine clinical research may (and should) include this important distinction.
Triglycerides are a type of fat found in the blood that consists of a glycerol backbone and three fatty acids. Triglycerides convert and store unused energy from food in fat cells and provide the body with energy between meals. Chronically elevated triglycerides can contribute to atherosclerosis and increases in CVD risk.43
Abnormal cholesterol metabolism
Abnormal circulating concentrations of lipids may manifest as elevated total cholesterol, elevated LDL‑c, or decreased levels of HDL‑c, or some combination of these. Hyperlipidemia refers to the elevation of LDL‑c, total cholesterol, and triglycerides. Dyslipidemia is a larger umbrella term that also includes abnormal (lowered) levels of HDL‑c. Research studies often use these terms interchangeably, and as such, both terms will be employed in this document according to the terminology used in the corresponding citation.
Approximately 35% of adults in the United States over the age of 20 have elevated total cholesterol.3 Elevated total cholesterol and dyslipidemia impair cardiovascular function and increase the risk for cardiovascular conditions such as atherosclerosis and hypertension.44 One study reported that the presence of hyperlipidemia is associated with a two‑fold risk of developing CVD.45
The accumulation of cardiac lipids is associated with impaired mitochondrial function, proinflammatory states, and systemic increases in oxidative stress.46 In addition, hyperlipidemia promotes the development of atherosclerosis and influences the electrophysiology of the heart.46 It also affects systolic function and decreases coronary blood flow reserve and capillary density. Endothelial cell apoptosis and impaired left ventricular function have also been associated with hyperlipidemia.46
Dyslipidemia is also associated with thrombosis and embolism, and predisposes individuals to cardiac events.47,48 Primary dyslipidemias may be driven by alterations in gene expression. Comorbidities and environmental factors are causative in secondary dyslipidemias.49
Coronary artery disease
The coronary arteries supply the heart with blood. When the blood flow through the coronary arteries is impeded, the heart may no longer have a proper blood supply and cardiac events can occur. Severe structural damage and other downstream events may occur as a result.38
Coronary artery disease (CAD), also known in literature as coronary heart disease, is a heart condition characterized by atherosclerotic plaque formation in the vessel lumen.50 Currently,
CAD is the leading cause of death worldwide.50 CAD is estimated to represent 2.2% of the overall global burden of disease and 32.7% of CVDs, amounting to 15.5 million Americans.50 Coronary heart disease alone caused 41.2% of CV‑related deaths in the United States in 2020.3 Because of its prevalence, it is a major topic of research and will be discussed in later sections of this document.
CAD begins with a vascular insult and results in plaque formation through foam cell proliferation.50 The plaque may cause impaired blood flow and oxygen delivery to the myocardium, potentially resulting in angina symptoms.50 Plaque may rupture and cause thrombosis, triggering a subtotal or total blockage of the lumen.50 When this occurs, CAD may be classified as stable ischemic heart disease or acute coronary syndrome.50 If not controlled, CAD can further progress into congestive heart failure.50
Modifiable risk factors of CAD include smoking, abnormal lipid levels, poor nutritional status, toxic load, excessive alcohol consumption, and stress.50,51 Dietary and lifestyle recommendations to address these modifiable risk factors will be discussed in detail in later sections of this document.
Cellular energy
ATP
Every cell in the human body requires adenosine triphosphate (ATP) for energy production. The maintenance of ATP levels is critical for cardiovascular health. Depleted ATP levels in the heart may be associated with altered cardiac cellular function.52
ATP provides energy for critical cardiac cellular functions. Conditions related to reduced blood flow or oxygenation can affect normal cellular production of energy compounds such as ATP. Reduced ATP levels can disrupt calcium balance in cardiac myocytes, causing calcium to remain bound to troponin for an extended period during the diastolic phase, potentially leading to diastolic dysfunction or alterations in ventricular function.52
Carnitine shuttle
The carnitine shuttle is a critical process for cellular functioning and for the prevention of the buildup of potentially harmful long‑chain substrates and acyl‑coenzyme A.53 Long‑chain fatty acids require the carnitine shuttle to facilitate fatty acid transportation.54 As a result, free carnitine availability may be a factor in the regulation of fatty acid oxidation.55
High amounts of carnitine are found in muscles of the human body. During physical exercise, mitochondrial fatty acid oxidation is a critical contributor to muscle metabolism.55 The mitochondrial availability of carnitine during high‑intensity exercise can be limited.
NAD+ and sirtuins
Nicotinamide adenine dinucleotide (NAD+) is found in every living cell and is essential to many important cellular processes including energy production in the mitochondria. NAD+ is involved in over 500 chemical reactions in the human body including reduction‑oxidation reactions; most anabolic and catabolic reactions such as fatty acid beta‑oxidation, glycolysis, and the tricarboxylic acid cycle; and the synthesis of cholesterol, steroids, and fatty acids.56 Biosynthesis of NAD+ is derived from tryptophan or salvage pathways from one of its four
precursors: nicotinamide riboside (NR), nicotinic acid, nicotinamide, and nicotinamide mononucleotide (NMN). Synthesis of NAD+ from tryptophan requires eight steps, whereas synthesis from NR to NAD+ requires two or three steps depending on the salvage pathway, with NMN as an intermediary.
Sirtuins (SIRT) are NAD+‑consuming enzymes, and NAD+ acts as a SIRT activator. NAD+ substrates, such as NADH and nicotinamide, act as inhibitors of SIRT.57,58 There are seven sirtuins in mammalian cells, which play a variety of roles in cellular function, including energy homeostasis, cell cycle, and apoptosis. Increasing NAD+ levels have been shown to activate Sirtuin 1 (Sirt1) to mitigate some of the age‑related effects and other downstream effects of reduced Sirt1 activity, such as mitochondrial function.59 These may also mediate inflammatory and stress responses, and support cardiovascular and neurological function.60 Because one of Sirt1’s main targets is NF‑κB, it is particularly relevant to the support of cardiovascular health and inflammation.57
Blood glucose balance
Research indicates that approximately 70% of individuals with prediabetes may develop type 2 diabetes mellitus (T2DM).61 More than 1 in 3 American adults (approximately 96 million) have prediabetes, and of those, more than 80% are unaware of their prediabetic status.62 Globally, the incidence of T2DM is predicted to rise from 415 million to 642 million by 2040.42 The risk of developing CVDs becomes elevated at the prediabetes stage.42
Research indicates that after progression to T2DM, the risk of CVD is approximately twice as high, which is equivalent to the risk associated with having had a previous myocardial infarction.42
Blood sugar imbalances and certain CVDs share some common etiologies. Oxidative stress and chronic inflammation are contributing factors to the development of insulin resistance, prediabetes, and T2DM.42 The topic of blood sugar imbalances deserves its own dedicated discussion beyond the scope of this document. However, because the discussion of glucose metabolism is often linked to cardiovascular health in research, it will be mentioned throughout sections of this document.
Microbial health
Evidence suggests that the gut microbiome may influence certain aspects of cardiovascular health. Compositional differences in gut microbiota have been observed in individuals with certain cardiovascular illnesses when compared to healthy populations.1 The gut‑heart axis refers to the bidirectional relationship between the gut microbiome and cardiovascular health.1 Evidence suggests that gut microbial composition may contribute to factors related to chronic inflammation and cardiovascular health.63 When compared to healthy individuals, research indicates that the composition of the gut microbiome may differ in individuals with certain CV diseases such as atherosclerosis.1 In addition, the abundance of certain microbes including those in the Bacteroides, Lactobacillus, and Clostridium genera has been linked to certain predictors of CAD.1 In one clinical study, the gut microbiome of individuals with coronary atherosclerosis was shown to have significant compositional differences when compared with healthy individuals.1
The downstream implications of this may include changes in the amount of metabolites produced by microorganisms.1 Microbial metabolites such as short‑chain fatty acids (SCFAs) have been linked to certain protective effects regarding cardiovascular outcomes.1 SCFAs including acetate, propionate, and butyrate are metabolites primarily made in the gut microbiome upon digestion of dietary fiber and are present in small amounts in some foods such as specific dairy products. They have been linked in research to certain aspects of blood pressure regulation.64 SCFAs have been shown to bind to certain olfactory receptors in the kidney, sending signals to play a role in the secretion of renin, an important molecule in blood pressure regulation.65 SCFAs have also been shown to maintain the epithelial barrier, reduce sympathetic activity, and support a healthy inflammatory response.64
Dysbiosis among certain gut microbiota may also influence parameters related to hypertension. A recent review article aggregated information on over 15 bacteria including those from the Methanobrevibacter, Oxalobacter, Klebsiella, and Salmonella genera.66 The authors reported that dysbiosis in the gut associated with these genera may be linked to the presence of hypertension. Other microbes have been associated with the presence of normal blood pressure, including Bifidobacterium, Butyrivibrio, and Coprococcus 66
Atherogenic changes and dysbiosis in the gut microbiome may be linked to cardiovascular illnesses such as acute myocardial infarction (AMI).63 In clinical studies involving individuals with AMI, gut microbial compositional differences were observed in 14 types of bacteria and over 30 microbial metabolites.63 Spirochaetes, Lachnospiraceae, Synergistetes, and Syntrophomonadaceae have been shown to be significantly more abundant after AMI, alongside decreased secretions of certain metabolites including SCFAs.63
Recent research has linked decreases in intestinal epithelial barrier health and gut microbial imbalances with certain changes related to cardiovascular function.63 A metagenomic analysis reported significant differences in gut microbial composition when comparing fecal samples from individuals with chronic heart failure with those from a control group.63
In addition, changes in LDL‑c levels in the bloodstream have been related to the formation of plaque in the blood vessels.63 Recent research has observed increases in LDL‑c levels and certain markers related to the inflammatory response concurrent with increases in Bacteroides fragilis (B. fragilis) levels. Increased levels of B. fragilis have also been associated with decreases in Lactobacilli and increases in Desulfovibrionaceae. The family Desulfovibrionaceae has been associated with deleterious changes in glucose and lipid metabolism.63
The body’s inflammatory response is also thought to be an important factor in the gut‑heart axis.63 Metabolites from microbes in the GI tract may also influence the inflammatory response and cardiovascular health. Butyrate and propionate have been shown to help support intestinal mucosal immunity and the inflammatory response. An animal study observed increases in colonic Treg cells and factors related to a normal inflammatory response in mice with diets fortified with butyrate and propionate. Decreased levels of SCFAs have been associated with age‑related changes and increases in certain parameters related to some CVDs. Certain probiotics and plant polysaccharides have been shown in recent research to help support gut microbial balance, the increase of SCFA levels, and the reduction of inflammatory cytokines such as IL‑1β, IL‑6, and TNF‑α.63
SCFAs have also been shown to help support the inflammatory response through the modulation of regulatory T cells, IL‑1ß, and IL‑6.63 Butyrate has been shown to help decrease TNF‑α levels and help increase plasma levels of IL‑10, a cytokine associated with anti‑inflammatory actions.63
Modifiable Factors that Influence Cardiovascular Health
SCFAs have been linked to the inhibition of histone deacetylase, an enzyme associated with alterations in blood pressure and inflammatory status.66
Emerging research has suggested that probiotics may help support some factors associated with certain cardiovascular pathologies. The supportive role of probiotics in CV health will be discussed in the following section.
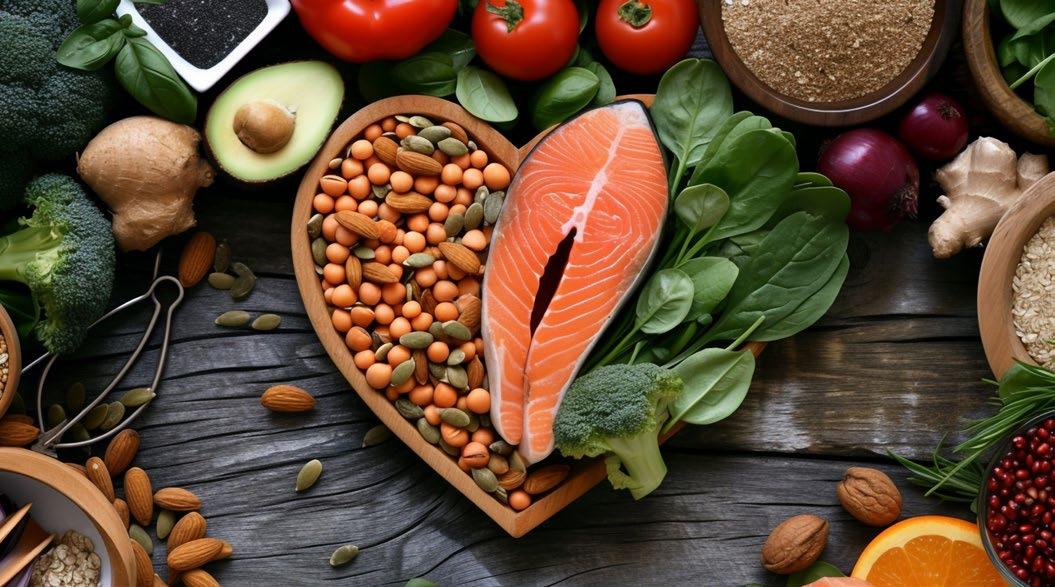
Micronutrients to Support Cardiovascular Health
While by no means an exhaustive list, this section highlights vitamins, minerals, botanicals, and other micronutrients that have been recently studied in the clinical setting. Although most micronutrients are discussed in this document individually, many have demonstrated synergistic qualities. Notably, emerging research on nitric oxide support and synergies among certain vitamins, phytonutrients, and minerals is discussed in a dedicated section titled “Synergistic nitric oxide support.”
This section also includes a reference table categorizing how the featured micronutrients may support overall cardiometabolic health.* The table serves as a guide to assist in making decisions based on specific patient needs. For instance, if a patient requires support in both cardiovascular health and blood sugar homeostasis, consulting this chart may suggest that resveratrol and/or delta‑ and gamma‑tocotrienols could be suitable choices to address both concerns.*
Key Nutrients
Quick Reference Chart
Rhamnan sulfate
CoQ10
Tocotrienols
Grape seed extract
Berberine
Fish oil
Magnesium
Vitamin D
Probiotics
Bergamot
D-Ribose
Alpha-lipoic acid
Selenium
Taurine
Nicotinamide riboside
Carnitine
Nattokinase
Nitric oxide synergistic support: Vascanox HP® ingredients
Rhamnan sulfate
Rhamnan sulfate is an extract from Monostroma nitidum (M. nitidum), a marine plant that is found off the coast of Japan. M. nitidum is a green algal seaweed that is often used for nori in miso soups and other culinary applications. The cells of M. nitidum are arranged in a single layer and contain many bioactive micronutrients including a high amount of chlorophyll, vitamins, polysaccharides, minerals, and proteins. Rhamnan sulfate is a sulfated polysaccharide located in the cell walls of M. nitidum; recent clinical studies indicate it may promote vascular endothelial integrity and cardiovascular health.67
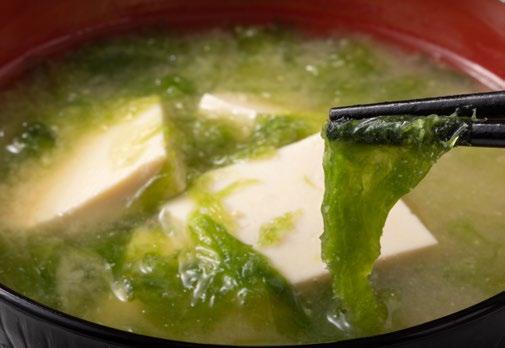
A laboratory and animal study investigated the potential role of rhamnan sulfate administration in cardiovascular health and endothelial integrity.68 In the laboratory portion of the study, human coronary artery endothelial cells and vascular smooth muscle cells were assessed for LDL permeability, inflammatory status, and vascular cell proliferation and migration in the presence of rhamnan sulfate. Laboratory results indicate that rhamnan sulfate helped support endothelial barrier function and helped promote normal cell proliferation and migration.68 In addition, in the presence of rhamnan sulfate, LDL permeability was reduced by 2.7 fold and results indicate that rhamnan sulfate exhibited protective qualities to help prevent further LDL deposition.68
In the controlled animal portion of the study, ApoE‑/‑ mice were used.68 ApoE‑/‑ mice are models used to simulate atherosclerotic changes.69 The mice were fed a high‑fat diet for 4 weeks as a baseline. For the treatment period, both the control and treatment groups received high‑fat diets for nine weeks; the treatment group also received 0.75 g of rhamnan sulfate per kg of body weight. Study results indicate that the treatment group experienced reductions in markers related to lipid metabolism and the inflammatory response in the presence of rhamnan sulfate administration. These effects were reported to be more pronounced in the female mice in the study.68
Overall study conclusions indicate that rhamnan sulfate helped support the barrier integrity of endothelial cells and acted as an inhibitor of NF‑κB pathway activation induced by TNF‑α.68 TNF‑α has been linked in the literature to the degradation of the endothelial glycocalyx (EGX).70 Rhamnan sulfate helped prevent the degradation of the EGX and the potential deposition of LDL.68
Additional studies indicate that rhamnan sulfate may also support the inflammatory response in vascular endothelial cells. Laboratory studies suggest that rhamnan sulfate may possess some properties to help attenuate platelet aggregation to potentially address some endothelial changes that may occur due to injury.67 Vascular endothelial injury may result in an increased expression of factors that may lead to hypercoagulation and changes to the rate of fibrinolysis.67
A controlled animal study explored the potential efficacy of rhamnan sulfate on mice treated with lipopolysaccharide (LPS).70 LPS in mice removes the vascular EGX and induces the expression of the pro‑inflammatory cytokines IL‑6, IL‑1β, and TNF‑α. Study results indicate that rhamnan sulfate administration helped attenuate LPS‑induced increases in IL‑6 and helped reduce the expression of other pro‑inflammatory and thrombus‑promoting agents.70 Rhamnan sulfate also helped reduce neutrophil infiltration into certain tissues and the expression of certain molecules associated with vascular adhesion including E‑selectin and VCAM‑1. Overall study results indicate that rhamnan sulfate helped to increase EGX levels in mice and helped protect against LPS‑induced damage.70
In human and animal studies, rhamnan sulfate has also demonstrated beneficial effects on lipid and glucose metabolism.67 Daily rhamnan sulfate supplementation for 6 weeks was shown in one clinical study to significantly reduce total and LDL cholesterol in individuals with borderline or mild hypercholesterolemia.67 Rhamnan sulfate may also interact with mucosa‑associated lymphoid tissue; studies indicate that rhamnan sulfate can bind to certain cells located in Peyer’s patches in the small intestine.67
CoQ10
Coenzyme Q10 (CoQ10), also known as ubiquinone due to its ubiquitous presence within the human body, is an important molecule in cellular health and cardiovascular function. CoQ10 has many roles in the body; it supports antioxidative status and cell membrane stability. It acts as an electron carrier in the mitochondrial electron transport chain during oxidative phosphorylation. It also plays a critical role in cellular energy production.71 CoQ10 supports cellular health through membrane stabilization, free radical scavenging, and stabilization of calcium‐dependent channels. It also plays a critical role in cellular respiration and ATP generation.72 Perhaps most famously, CoQ10 helps protect against statin‑induced myopathies.73
A prospective randomized trial investigating the potential role of CoQ10 in cardiovascular health reported that long‑term supplementation helped support symptom improvement and the prevention of certain adverse cardiovascular events in elderly individuals with heart‑related pathologies.74 Another clinical trial explored the potential efficacy of CoQ10 supplementation in the presence of heart failure with preserved ejection fraction (HFpEF) and impaired diastolic function in elderly individuals.74 This prospective, double‑blind, placebo‑controlled pilot study involved 19 individuals receiving 300 mg of CoQ10 daily for four months with 20 individuals receiving placebo. Biometrics assessed include echocardiography and serum N‑terminal pro‑B‑type natriuretic peptide (NT‑proBNP).74 NT‑proBNP is a biomarker used to test for cardiac stress and assess conditions such as heart failure, aiding in diagnosis, prognosis, and treatment monitoring.
While previous studies have observed improvements in diastolic function in the presence of CoQ10 supplementation in certain individuals, the authors of this study did not observe an overall significant effect on diastolic function at the study terminus. However, the treatment group experienced a greater decrease in mean NT‑proBNP levels as compared to placebo.74 Significant differences were also observed in certain echocardiographic parameters in the CoQ10 group.74
Study limitations include a relatively small sample size, a high dropout rate, and the measurement of biometrics unrelated to mitochondrial function or cellular energy production. In addition,
Micronutrients to Support Cardiovascular Health
the authors cite a relatively short treatment duration as an explanation of findings; more research is needed before clinical conclusions can be made. Study strengths include a carefully considered dosing rationale and a high compliance rate for the remaining participants.
CoQ10 has also been shown in recent studies to support gum health in response to age‐related changes; certain deficiencies in CoQ10 have been linked to periodontal disease. Increased concentrations of CoQ10 have been reported in studies to help suppress periodontal inflammation in the presence of diseased gingiva.72
Another prospective randomized double‑blind placebo‑controlled trial explored the potential role of certain micronutrients including CoQ10 to support cardiovascular health.75 This study included data from 221 older adults. The treatment arm consisted of 200 mg of coQ10 and 200 µg of selenium daily for 48 months. Quality of life and parameters related to antioxidative capacity and cardiovascular health were assessed. In particular, the amounts of albumin‑adjusted serum free thiols were evaluated throughout points in the study. Free thiols have been used as a biomarker for cardiovascular‑related health.75 Significant improvements in cardiovascular mortality risk and parameters related to systemic oxidative stress were observed at the study terminus. Improvements in serum free thiol amounts were reported and were correlated inversely with cardiovascular‑related risk. In particular, improvements related to antioxidative status and free thiol levels were observed to be greater in older individuals with lower selenium levels at baseline.75
Vitamin E tocotrienols
The vitamin E family consists of two subgroups: tocopherols and tocotrienols.76 These two subgroups can be broken down further into their corresponding isomers (alpha, beta, gamma, and delta), totaling eight vitamin E isomers.76
On a molecular level, the structural differences between tocopherols and tocotrienols contribute to their different actions. For instance, the bond types and composition of tocotrienol side chains help support a more even distribution and fluidity with the cell’s phospholipid bilayer.77
Research suggests that vitamin E tocotrienols exert clinically relevant benefits in cardiometabolic health that are unique to this group of isomers.76 In addition, tocotrienols have a relatively high bioavailability and may possess additional qualities in support of cellular health, lipid metabolism, and healthy blood glucose homeostasis.77,78 The annatto plant is the first and only true source of nature‑derived tocotrienol‑only vitamin E isomers.
Typical commercial vitamin E supplements are low or absent in tocotrienols and, instead, are high in alpha‑tocopherol. Interestingly, human studies reveal that if simultaneously administered, alpha‑tocopherol may attenuate the bioavailability of tocotrienols and may
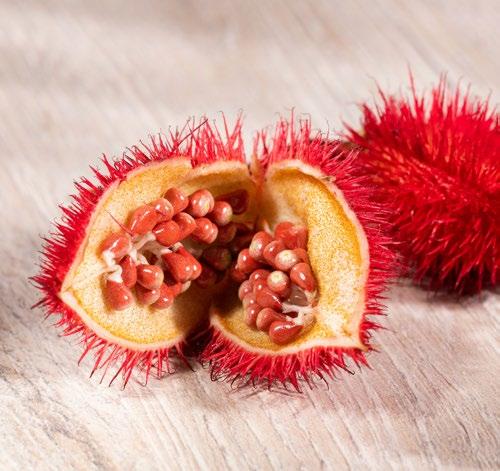
interfere with the potential clinical benefits of tocotrienols.76,77 Tocotrienols have been shown to be approximately 40 to 60 times more potent than tocopherols at protecting against cellular damage and oxidative stress.79 When supplemented without the presence of tocopherol isomers, vitamin E tocotrienols may promote cardiometabolic health in numerous ways.
Tocotrienols have been shown to help suppress IL‑6, TNF‑α, CRP, and malondialdehyde, and help modulate the NF‑κB pathway.80,81 Clinical studies have explored the potential supportive role of delta‑tocotrienol in support of optimal lipid metabolism; significant improvements in certain biomarkers related to inflammation, oxidative stress, cholesterol metabolism, and cardiovascular health have been reported.82
In vitro and animal studies have also shown that tocotrienols may help maintain healthy blood pressure, potentially mitigate atherosclerotic lesions, and help attenuate the effects of endothelial inflammation.83–85 Specifically, gamma‑tocotrienol has been shown in animal models to significantly reduce systolic blood pressure and improve endothelial NO synthase activity, both of which are key in supporting normal blood flow and circulation.86
A recently published systematic review and meta‑analysis explored the potential connection between tocotrienol supplementation and glucose metabolism in individuals with T2DM.77 The study included data from 10 randomized controlled trials that administered tocotrienol‑rich fraction as the primary intervention. Tocotrienol‑rich fraction consists of a 70% mixture of tocotrienol isomers. Sample sizes ranged from 43 to 300 participants and study durations were between 8 weeks and 18 months. Administered amounts ranged from 200 to 420 total tocotrienols. Parameters related to blood pressure, inflammation (high sensitivity C reactive protein [hs CRP]), and blood sugar homeostasis (HbA1c) were included in the meta‑analysis.77
Overall conclusions of the meta‑analysis include significant decreases in HbA1c. Upon subgroup analysis, significant reductions in HbA1c were indicated when the duration of T2DM was less than 10 years, when the intervention lasted less than 6 months, and with a baseline HbA1c lower than 8.0%. In individuals with an HbA1c baseline higher than 8.0%, greater reductions in HbA1c were observed when compared with those with a lower than 8.0% baseline upon tocotrienol supplementation.77 While only non‑significant decreases in systolic blood pressure and hs‑CRP were reported, these results indicate that future clinical studies assessing tocotrienols’ effect on these parameters are warranted.
Notable individual RCTs regarding blood sugar homeostasis support include one study involving 110 participants with T2DM on a hypoglycemic agent who were randomly divided to consume 250 mg/day of tocotrienols or a placebo for 24 weeks.87 Compared to the placebo, the experimental group exhibited improved glycemic control, reduced inflammation (analyzed through hs‑CRP, TNF‑α, and IL‑6), and decreased oxidative stress (analyzed through MDA).87 An RCT of 77 patients with prediabetes demonstrated that individuals consuming 300 mg/ day of tocotrienols exhibited significantly improved glycemic status and glucose utilization compared to the placebo.88
Another notable study described research regarding the efficacy of supplementation with delta‑tocotrienol on markers related to inflammation, oxidative stress, and cholesterol in individuals with hypercholesterolemia.89 Thirty‑one individuals over the age of 50 with hypercholesterolemia received varying doses of delta‑tocotrienol for thirty weeks. All participants received titrated doses (125, 250, 500, 750 mg daily) of DeltaGold® delta‑tocotrienol increasing every four weeks with a two‑week washout period in between. The American Heart Association Step 1 diet was also part of the study intervention. Biomarkers assessed include
serum total cholesterol, HDL‑cholesterol, LDL‑cholesterol (LDL‑c), triglycerides, and certain cytokines associated with CVD and inflammation.
Delta‑tocotrienol was shown to support antioxidative status and healthy inflammation in a dose‑dependent manner, with optimal results at a dose of 250 mg daily. Decreases in serum CRP, NO, malondialdehyde (MDA), gamma‑glutamyl transferase (GGT), total cholesterol, LDL‑c, and triglyceride levels were observed. Study observations also included the downregulation of plasma inflammatory microRNAs including miR‑101a, miR‑125a, miR‑155, miR‑223, and miRNA‑146a in the presence of delta‑tocotrienol supplementation. Elevated levels of miRNA‑146a have been associated with cellular senescence.89
Overall study conclusions indicated that daily supplementation with 250 mg of delta‑tocotrienol provided the most significant improvement in certain biomarkers associated with oxidative stress, inflammation, and cardiovascular health. The treatment group receiving 250 mg daily of delta‑tocotrienol showed improvements of 40% in CRP and NO, 34% in MDA, and 22% in GGT. Improvements of approximately 15% of the proinflammatory cytokines IL‑1, IL‑12, and interferon‑gamma were also reported in this group.89
Clinical studies have also demonstrated the potential benefits of tocotrienol supplementation on individuals with metabolic syndrome (MetS).90 Combining tocotrienols with other nutrients that promote healthy inflammatory responses, such as quercetin, resveratrol, and B vitamins, may synergistically affect metabolic health, as observed in clinical trials.91 In a 24‑week RCT with 82 patients with MetS, groups consuming 500 mg/day of delta‑tocotrienols and 300 mg/ day of resveratrol for 24 weeks exhibited a significant reduction in blood pressure, mean fasting plasma glucose, and serum triglyceride, and an increase in HDL cholesterol.90 A decrease in hs‑CRP, IL‑6, TNF‑α, and MDA, as well as an increase in total antioxidant capacity, was also observed compared to the placebo group.90
Stilbenes: Resveratrol and Pterostilbene
Stilbenes are a class of bioactive molecules that support antioxidative status, a normal inflammatory response, cellular health, and cardiovascular function. Resveratrol is the most well‑known of the stilbenes.
Resveratrol
Resveratrol is a polyphenolic compound found in grape skin, dark berries, and peanuts. It acts to support antioxidative status by targeting the transcription factor Nrf2 and helping support antioxidant protein expression, mitochondrial coupling, and astrocyte activity.92 It also helps support the attenuation of monocyte adhesion to endothelial cells.17 In addition, resveratrol helps support the suppression of NF‑κB.17 It may also help promote vascular health by helping protect the endothelial glycocalyx.93 Resveratrol may also help modulate cyclooxygenase (COX)‑1, COX‑2, prostaglandins, leukotrienes, IL‑1β, and NF‑κB.94 It may also help modulate autophagy. The autophagy of senescent cells may help restore homeostatic conditions during the aging process.20 Resveratrol may also help support normal activity of iNOS and the inhibition of ROS production.20
A systematic review and meta‑analysis investigated resveratrol’s role in CVD and inflammatory status. The study aggregated data from six RCTs that explored the efficacy of resveratrol in
individuals with CVD. The RCTs assessed inflammatory status by using the biomarkers CRP, TNF‑α, and IL‑6.17
Among the RCTs assessed, daily resveratrol amounts spanned from 8.1 mg to 500 mg lasting between 28 and 360 days. Sample sizes per study were between 20 and 30 participants. Subgroup analysis revealed that resveratrol amounts above 15 mg daily had a significant effect on CRP and TNF‑α levels in individuals with CVD. CRP and TNF‑α were not affected significantly in the subgroup analysis of consumption of resveratrol below 15 mg daily.17
The authors reported overall significant decreases in CRP and TNF‑α in the presence of resveratrol supplementation in individuals with CVD.17 Resveratrol has also been shown to influence the Firmicutes to Bacteroidetes (F/B) ratio in the gut microbiome.95 Higher F/B ratios have been associated with increases in the occurrence of certain diseases. Resveratrol and other stilbenoids have been shown in studies to decrease the relative abundance of Firmicutes resulting in decreases in the F/B ratio.95
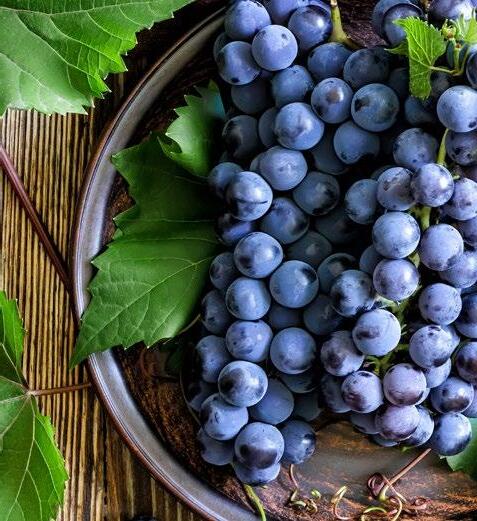
Animal studies have also indicated that resveratrol may influence the population of SCFA‑producing bacteria.96 One study involved supplementation with resveratrol for eight weeks and reported increases in Lachnospiraceae, Blautia, and Dorea populations which are known to produce butyrate, which potentially may have a downstream beneficial effect on cardiovascular health.96
Through in vitro and in vivo experimental models, resveratrol has been shown to promote mitochondrial biogenesis in various cell types, including those found in the liver, cardiovascular system, brain/neurons, adipose tissues, and muscles.97 Resveratrol may promote mitochondrial function during metabolic dysfunction, such as unhealthy glucose metabolism.97 Furthermore, resveratrol may support mitochondrial mass and DNA content, potentially supporting the constituents of the electron transport chain and factors related to mitochondrial biogenesis.98 It may also support SIRT1‑dependent nitric oxide production, which may help promote mitochondrial biogenesis.98 Promoting the number and efficiency of mitochondria supports energy production, enhanced endothelial function, and improved redox balance, ultimately supporting optimal cardiac function and vascular health.
Pterostilbene
Pterostilbene (trans‑3,5‑dimethoxy‑4‑hydroxystilbene) is a molecule found in blueberries that is structurally similar to resveratrol. Animal studies indicate that pterostilbene may have a comparatively high bioavailability.99 It has been shown to support antioxidative status, a healthy inflammatory response, and cellular health.99 Pterostilbene may also modulate certain age‑related biochemical processes including telomere attrition, cellular senescence, and sirtuin
activity.16 It also increases superoxide dismutase and glutathione activation through the Nrf2 signaling pathway.16
The aging process involves many biochemical pathways that affect cellular health. Pterostilbene has been shown to influence many processes to support cellular regeneration and healthy aging. It may play a protective role in high glucose‑induced oxidative injuries through the activation of Nrf2 in hippocampal neuronal cells.16 Animal and laboratory studies indicate that pterostilbene may modulate certain proinflammatory agents including IL‑1β, IL‑6, IL‑18, TNF‑α, and vascular endothelial growth factor.16 It has also been shown to help attenuate hypoxia‑reoxygenation injury in cardiomyocytes.16
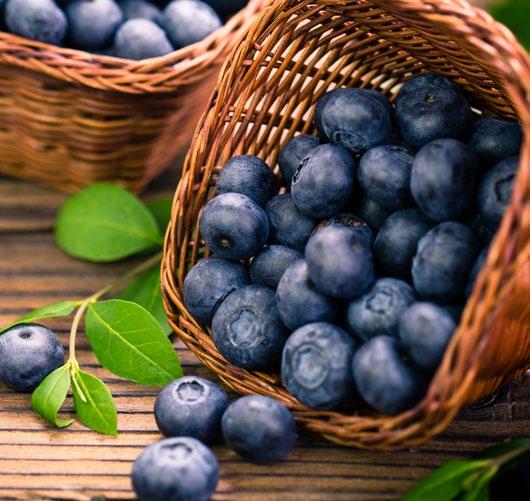
Cardiovascular studies have shown that pterostilbene may help play a protective role against atherosclerosis in vascular smooth muscle cells and vascular endothelial cells.99 It may also support intercellular communication, liver health, and healthy metabolism.99 It has been shown in animal studies to help support healthy glucose metabolism, antioxidative status, and a healthy response to inflammation.99
In a laboratory study, the administration of pterostilbene was shown to help reduce MDA and other biomarkers associated with oxidative stress.16 A clinical trial reported a significant increase in serum total antioxidant status (TAS) in healthy older individuals in the presence of pterostilbene administration for four weeks.16
Berberine
Berberine is an extract from several botanicals including goldenseal, Oregon grape, and barberry that has been shown to support healthy blood sugar and lipid metabolism and insulin sensitivity.100 It has also been shown to help decrease the intestinal absorption of cholesterol and helps promote the synthesis of bile acids.101 It has also been reported to impact the secretion of glucagon‑like peptide‑1 (GLP‑1), a hormone involved in glucose metabolism and insulin release, and the sensitivity of GLP‑1 receptors.
In a three‑month randomized placebo‑controlled clinical trial assessing the effects of 300 mg daily berberine supplementation on individuals with mild hyperlipidemia, total cholesterol and LDL‑c levels decreased.101 A systematic review and meta‑analysis including data from over 1,300 participants examined berberine’s therapeutic role in hyperlipidemia and found that berberine significantly reduced total cholesterol and LDL‑c levels when compared with placebo.102 A separate meta‑analysis reported similar findings and included berberine’s ability to lower triglyceride levels.101
Micronutrients to Support Cardiovascular Health
A systematic review and meta‑analysis included 44 randomized controlled trials and 4,606 participants who were investigated for the effects of berberine supplementation for patients who had CVD (who were taking the supplement alone or in combination with statins).103 The patients receiving berberine alone displayed numerous clinical benefits, including significantly reduced National Institute of Health Stroke Scale (NIHSS) scores, hs‑CRP, TNF‑α, and intima‑media thickness.103
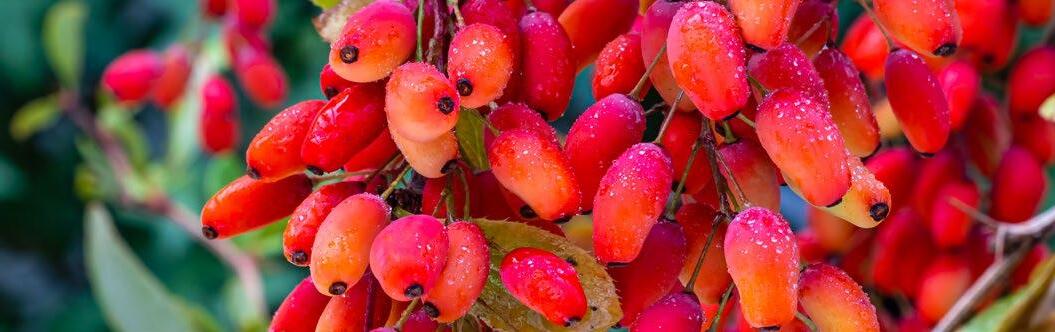
In an in‑depth review of 49 clinical studies, the participants who were administered berberine supplementation exhibited improved lipid status (triglyceride, total cholesterol, and LDL‑c), blood sugar metabolism, and systolic blood pressure.104 Specifically related to blood sugar metabolism, the review observed significantly reduced fasting blood glucose, insulin, HbA1C, and HOMA‑IR (homeostatic model assessment for insulin resistance) in participants receiving berberine supplements. The researchers pointed out that berberine may be clinically beneficial to those with impaired metabolic health, in particular.104
Studies with rodent models suggest that berberine may also promote a healthy gastrointestinal (GI) microbial environment. A healthy GI microbial environment may further promote healthy inflammatory responses and support healthy lipid status and blood sugar metabolism.104
Fish oil
Fish oil contains polyunsaturated fatty acids (PUFAs), which are fatty acids with two or more carbon‑to‑carbon double bonds.105 Their nomenclature is based on the position of where the first double bond is located. The category of omega‑3 (n‑3) PUFAs primarily comprises eicosapentaenoic acid (EPA) and docosahexaenoic acid (DHA), both demonstrated to promote a healthy response to inflammation, antioxidative status, and various aspects of cardiovascular health.106
Notably, alpha‑linolenic acid (distinct from alpha‑lipoic acid, which is addressed elsewhere in this document) is classified as an n‑3 PUFA; however, it is poorly converted (<5%) into EPA and DHA in the body.106
Alternatively, omega‑6 (n‑6) PUFAs participate in pro‑inflammatory processes in the body. Typical western diets contain a higher amount of n‑6 than n‑3 PUFAs.105 Recent research suggests that the ratio between n‑6/n‑3 PUFAs in the diet may be a useful predictor of
inflammatory status and other parameters related to cardiovascular health. Low n‑6/n‑3 ratios (implying greater intake of n‑3 PUFAs) have been associated with the downregulation of CRP expressions and reductions in aortic plaque lesions.106
Omega‑3 PUFAs are thought to support the body’s response to inflammation through many pathways, including competition with the formation of eicosanoids from arachidonic acid.107 EPA and its metabolites have not only been shown to mitigate the infiltration of polymorphonuclear leukocytes (PMNs) but also to enhance phagocytosis‑induced PMN apoptosis, diminish fibroblast activation, promote the production of anti‑inflammatory metabolites of the COX‑2 enzyme, and decrease levels of pro‑inflammatory molecules such as NF‑κB and IL‑12.107,108
DHA is a structural molecule stored in phospholipid cell membranes and is particularly enriched in mitochondria, endoplasmic reticulum, and synaptic terminals.109 The amount of DHA in these membrane structures supports improvement in membrane fluidity, neurotransmitter release, signal transduction, lipid raft function, transmembrane receptor function, myelination, gene expression, neuronal differentiation and growth, while reducing neuroinflammation.109,110
Specific to cardiovascular health, EPA has been shown to support normal cholesterol metabolism and the promotion of a healthy inflammatory response.106 DHA has been shown to support proper maintenance of normal triglyceride metabolism and blood pressure.106 Together, as n‑3 PUFAs, they have been shown to help reduce very low‑density lipoprotein and triglyceride levels by decreasing the activity of sterol receptor element binding protein‑1c.106 They have also been shown to help protect vascular endothelial cells and support ventricular structural remodeling.106 EPA and DHA may also help inhibit thrombosis formation through the modulation of the TXA2 and PGH2 platelet receptors. They may also help suppress the synthesis of TXA2.106
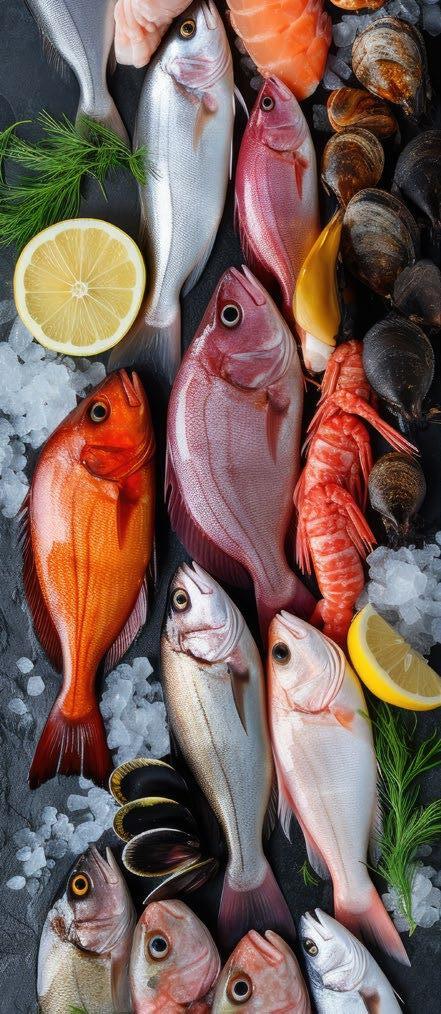
Laboratory studies have indicated that n‑3 PUFAs may help support normal heart rhythm and help stabilize electrical activity. They may also help modulate sarcolemmal ion channels and may help influence autonomic nerve function and improve vagal tone.106 n‑3 PUFAs may also help prevent the accumulation of plaque and other atherosclerotic changes.106
Micronutrients to Support Cardiovascular
In animal studies, diets rich in n‑3 PUFAs have observed increases in NO production and endothelial NO synthase expression. Increases in glutathione, superoxide dismutase, catalase, and guaiacol peroxidase activity has also been observed in the presence of n‑3 PUFAs.106
Omega‑3 PUFAs have been studied extensively in the clinical setting for their potential ability to help improve certain cardiac‑related parameters. In a clinical trial involving over 56,000 participants with a follow‑up between 3 and 20 years, the risk of developing hypertension was 27% lower among the normotensive individuals who consumed the highest amounts of n‑3 PUFAs.106 A large‑scale meta‑analysis of 70 RCTs reported that the combination of EPA+DHA helped reduce systolic blood pressure (SBP) by ‑1.52 mm Hg and diastolic blood (DBP) pressure by ‑0.99 mm Hg when compared to placebo, with the greatest improvements observed among those with untreated hypertension.106
Fish oil may also help support coronary artery health. One study involving 1.86 g of EPA and 1.5 g of DHA daily reported improvements in the progression of fibrous coronary artery plaque.106 A clinical trial involving over 18,000 individuals with hypercholesterolemia explored the potential efficacy of 1.8 g of EPA plus statin therapy when compared to statin therapy alone. After 4.6 years, reductions in major coronary events of 18% in those without a history of coronary artery disease were observed in the EPA+statin group. In another meta‑analysis that included data from over 45,000 individuals, DHA intake was linked with a lower risk of fatal coronary heart disease.106
Clinical studies have also found that n‑3 PUFAs may help reduce the risk of ventricular arrhythmia, atrial fibrillation, hospitalization among those with heart failure, and mortality.106 Regarding overall general cardiovascular outcomes, a meta‑analysis involving 13 RCTs reported that supplementation with n‑3 PUFAs helped lower the risk of myocardial infarction, coronary heart disease, and deaths related to CVD.106
Magnesium
Magnesium is the fourth‑most abundant mineral in the body and is critical to hundreds of biochemical processes.111 It is necessary for muscle contraction, energy production, nerve conduction, and the gating of calcium channels.111 It also plays a key role in nucleic acid synthesis, DNA repair, and genome maintenance.112 Pathways related to genome maintenance are essential for optimal cellular function.112
Current medical research has shown a link between magnesium deficiency and CVD. Chronic magnesium deficiency has been associated with an increased risk of hypertension, atherosclerosis, changes in lipid metabolism, stroke, and cardiac arrhythmias.113
Analysis of eight prospective cohort studies with information on 74,422 individuals and over 5,884 CVD events shows that the participants in the highest category of serum magnesium concentration had approximately 20% lower risk of total CVD events, as compared to individuals in the lowest serum magnesium concentration category.114
Magnesium acts as a cardiovascular regulator by maintaining electrical, metabolic, and vascular homeostasis, as well as supporting a healthy inflammatory response and protecting against oxidative stress.115 In animal studies, increases in oxidative stress markers have been observed in rats with magnesium deficiencies. Additionally, the severity of myocardium injury in magnesium‑deficient animals was reduced with antioxidant treatment.116
Micronutrients to Support Cardiovascular Health
Magnesium may also promote cardiovascular health and healthy BP by stimulating local vasodilator mediators, including NO, and modulating endothelium‑dependent and endothelium‑independent vasodilation.113,117 Systematic reviews and meta‑analyses have demonstrated an inverse association between magnesium intake and cardiovascular risk, including promoting healthy BP.117–119
Magnesium has shown to support the reduction of platelet stimulating factors and support platelet inhibiting factors in animal and laboratory studies.120,121 Magnesium is important in the function of many ion channels including sodium, potassium, and calcium. Hypomagnesemia has been shown to weaken the mechanism in potassium‑proton exchange and alters the concentration of sodium and calcium concentrations.115
A review explored the potential link between magnesium status and certain aspects of cardiometabolic function including endothelial integrity.112 Magnesium was shown to help support blood vessel integrity through its ability to help support calcium transport into contractile smooth muscle cells. It may also help inhibit the release of certain vasoconstrictive neurohormones and help increase NO production.112
Optimal endothelial function is related to antioxidative status and a healthy inflammatory response.112 Magnesium is involved in the synthesis of glutathione and may help protect against oxidative stress, a known contributor to atherogenesis and other CVDs.122 Evidence suggests it may also help support a normal inflammatory response through its ability to help modulate NF‑κB and the expression of certain pro‑inflammatory cytokines.115 Deficiencies in magnesium have been linked to increases in levels of IL‑1, IL‑6, TNF‑α, and VCAM.112
In addition, magnesium may also help promote extracellular matrix (ECM) health. It is critical to ECM structure and is required for the enzyme hyaluronan synthase. It also helps support critical proteins in the regulation of intracellular signaling cascades in vascular smooth muscle to help support stability and normal adhesion.112
A meta‑analysis of studies exploring the relationship between magnesium supplementation and hypertension observed decreases in both systolic and diastolic blood pressure after 11 to 12 weeks of supplementation with 368 mg to 410 mg of magnesium daily.112 A meta‑analysis of prospective cohort studies reported improvements in the risk of heart failure after dietary increases in magnesium of 100 mg daily.112 Research suggests that magnesium levels in the body may take 20 to 40 weeks to achieve homeostasis, so more longer‑term clinical studies are needed.112
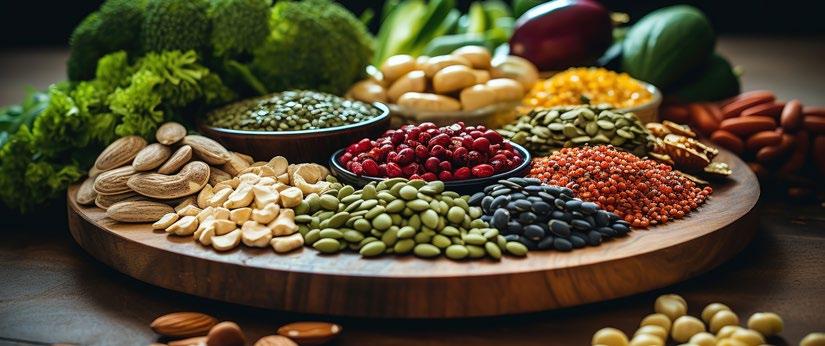
Magnesium orotate is a form of magnesium that has been shown to be particularly supportive in cardiovascular health. Magnesium orotate contains two components: magnesium and orotic acid. Orotic acid (OA) behaves as a transporter in helping magnesium enter cells.123
Additionally, OA may help improve the energy status of injured myocardium through stimulation of synthesis of glycogen and ATP.124,125 In an RCT, participants with severe congestive heart failure (NYHA IV) under optimal medical cardiovascular treatment were randomized to either receive magnesium orotate or placebo.126 After about one year, the survival rate of participants receiving magnesium orotate was 75.7%, as compared to 51.6% under placebo. The clinical symptoms of those receiving magnesium orotate improved 38.5%, as compared to those receiving placebo whose symptoms deteriorated 56.3%.126
A pilot study of individuals with coronary heart disease and left‑ventricular dysfunction showed statistically significant improvement in exercise tolerance after receiving magnesium orotate.127
Magnesium may also play a protective role in the risk of diabetes. Intracellular magnesium is a modulator of insulin action.128 In over 40 cohort studies involving more than one million participants with follow‑up ranging from four to thirty years, dietary magnesium intake was associated with a reduction in relative risk of T2DM by almost 20%.128 Supplementation with magnesium has been shown to improve insulin sensitivity and metabolic control. Clinical studies involving magnesium supplementation have observed favorable effects on several biomarkers related to T2DM.128
Dietary sources of magnesium include green leafy vegetables, whole grains, nuts, and seeds.
Vitamin D and Vitamin K
Although vitamin D is classified as a vitamin, it more closely resembles and functions like a steroid hormone with broad influences on physiology.129 Vitamin D receptors (VDRs) are found throughout the body and have been shown to influence the expression of thousands of genes.130,131 Vitamin D is well‑known for its supportive role in bone, mood, and immune health. Evidence suggests it also plays a role in cardiovascular and metabolic health and may help promote antioxidative status and a normal inflammatory response.132,133 VDRs are also present on the vascular endothelium, which suggests that vitamin D may also help regulate vascular endothelial function.132
Additionally, vitamin D influences many critical physiological processes related to cardiovascular health. It is involved in the RAAS system; evidence suggests that vitamin D supplementation may help to modulate RAAS activity and help improve certain parameters related to excessive RAAS activity.132 Evidence suggests vitamin D may also help support endothelial function, and may also support arterial integrity, although more research is needed on this topic.132
Vitamin D deficiency or insufficiency is common, and epidemiological findings indicate that almost 30% of the U.S. adult population is deficient in this vitamin with another 40% who are insufficient.132 Vitamin D deficiency has been linked to certain autoimmune and heart diseases.132,134,135 Evidence from clinical and epidemiological studies suggests a potential connection between suboptimal vitamin D status and hypertension.136,137 Evidence indicates that vitamin D3 (cholecalciferol) is far more effective in raising and maintaining serum 25(OH)D3 concentration, and D2 should not be considered an equivalent.138,139
Micronutrients to Support Cardiovascular Health
Several RCTs have explored the potential efficacy of vitamin D on hypertension and the RAAS pathway. One study involving high amounts of vitamin D reported significant reductions in SBP after 8 weeks when compared with placebo.132 Another study involved daily intake of 2,000 IU (50 mcg) of vitamin D for 6 months and reported significant decreases in 24‑hour SBP and DBP.132
A systematic review and meta‑analysis of RCTs published in 2019 showed that vitamin D supplementation, which included D3 and D2, was not statistically significant in lowering mortality. Yet, when D3 was studied as a subgroup, all‑cause mortality was significantly lower than in trials with D2.134
Deficiencies in vitamin D status have also been linked to T2DM.133 Hypovitaminosis D is thought to influence intracellular calcium levels and the function of Glucose Transporter Type 4 (GLUT4), which can impact insulin secretion.133
An RCT explored the potential supportive role of the combination of aerobic training and supplementation with vitamin D in individuals with T2DM.133 The study lasted for eight weeks and involved 48 men between 35 and 50 years of age. There were four groups: aerobic training (AT) plus vitamin D, AT plus placebo, vitamin D only, and control plus placebo. AT consisted of aerobic exercise for 20 to 40 minutes at 60% to 75% heart rate maximum at a frequency of three sessions each week. Vitamin D supplementation involved 50,000 IU once per week for eight weeks. Biomarkers assessed include inflammatory cytokines in peripheral blood mononuclear cells and markers related to antioxidative status, glucose metabolism, and vitamin D status.133
Excluding the group receiving control plus placebo, all groups receiving an intervention (either AT, vitamin D, or combined) showed improvements in biomarkers related to antioxidative status including total glutathione, superoxide dismutase, glutathione peroxidase, and total antioxidant capacity.133 All treatment arms also showed improvements in inflammatory markers including hs‑CRP, IL‑1β, and NF‑κB.133 Improvements in markers related to glucose metabolism also were observed including fasting blood glucose and HOMA‑IR.133
The treatment arm involving the combination of both AT and vitamin D reflected the largest improvements in inflammatory cytokines including IL‑1β, TNF‑α, and NF‑κB. The authors postulated that both AT and vitamin D may act on the body through different physiologic mechanisms and influence multiple downstream targets.133
Preliminary evidence suggests that vitamin D may also work synergistically with vitamin K to support arterial health. Vitamin K is a fat‑soluble vitamin critical to the carboxylation of osteocalcin in bone and helps support healthy bone density status, blood coagulation, and vascular health.140 In population studies, concurrent lowered vitamin D and K status has been associated with increased incidences of hypertension and arterial stiffness.141
A prospective clinical study investigated the potential effect that combined low vitamin D and K status may have on mortality risk.141 The study involved over 4,700 individuals; approximately 20% of participants had a combined lowered vitamin D and K status. After data analysis, the researchers determined that a combined lowered vitamin D and K status was associated with increased all‑cause mortality risk and a non‑significant risk for cardiovascular events and mortality.141 For an in‑depth review of vitamin K’s role in cardiovascular health and normal physiology, please refer to the Vitamin K chapter in the Textbook of Natural Medicine
Probiotics
Certain probiotics may help support microbial homeostasis, modulate pro inflammatory cytokines, and the production of certain microbial metabolites including SCFAs.1 Clinical studies have correlated the role of probiotic supplementation in support of certain atherogenic changes and other cardiovascular functions. A randomized placebo‑controlled twelve‑week trial involving species from the Bifidobacterium, Lactobacillus, Lactococcus, Streptococcus, and Enterococcus genera reported reductions in TNF‑α, SBP, and IL‑6 with greater effect at the higher amount of 10 billion colony forming units (CFUs) daily.1
Animal studies have explored the potential efficacy of probiotic supplementation on parameters related to atherosclerotic changes. Decreases in TNF‑α, LDL‑c, total cholesterol, and overall atherosclerotic preventive effects in the presence of Bifidobacterium adolescentis, Lactobacillus acidophilus ATCC4356, and L. paracasei were observed.1
Clinical studies reported similar results in the potential supportive role of probiotics in relation to atherogenic changes. A study involving B. animalis subs. Lactis reported an increase in endothelial function and decreases in triglycerides, total cholesterol, and C‑reactive protein.1 Another study involving the combined administration of L. acidophilus, L. reuteri, L. fermentum, and B. bifidum reported increased total antioxidant capacity and total glutathione.1
The authors conclude that probiotics may help support cardiovascular health and help mitigate certain aspects of atherogenic risk factors. This may be due to the potential for probiotics to help modulate certain proinflammatory cytokines, help support microbiota homeostasis, and macrophage polarization.1
In animal studies, spontaneously hypertensive rodents had a significant decrease in microbial diversity, suggesting a correlation between hypertension and the gut microbiome.142 Another study showed a significant decrease in SBP in animals fed sour milk.143 Additionally, salt‑sensitive hypertension has been shown in animal studies to be ameliorated by supplementation of Lactobacillus murinus through the modulation of T‑helper 17 cells.144
A systematic review and meta‑analysis was performed to explore the effect of probiotic supplementation on blood pressure, pooling data from over two thousand human participants.145 Significant decreases in both SBP (by ‑3.05 mmHg) and DBP (by ‑1.51 mmHg) were reported when probiotics were consumed by patients with certain conditions such as hypertension.145 Probiotics have been shown in a different meta‑analysis to improve lipid levels, blood pressure, and inflammation indicators in patients receiving probiotics for eight weeks.146
Certain probiotics may also help support the body’s response to hydrogen peroxide‑induced oxidative stress. Lactobacillus rhamnosus GG was shown to help promote free radical scavenging potential in a laboratory study.7 Another study found that L. casei and L. acidophilus exhibited the highest activity to promote antioxidative status when compared with 11 other Lactobacillus strains.7
An enzyme derived from Lactobacillus plantarum AKU1009a was shown in a laboratory study to help shield certain cells from hydrogen peroxide‑induced cytotoxicity.7 Other strains of L. plantarum have also helped modulate apoptosis, MDA levels, and protect certain cell lines from oxidative damage in the laboratory setting.7 In addition, increases in mitochondrial membrane potential and the modulation of glutathione and superoxide dismutase levels were observed.7
Bergamot
Bergamot (Citrus bergamia Risso) is a citrus plant from the Calabrian region of southern Italy. It is different from other citrus fruits because it has a high content of flavonoids and other bioactive phenolic compounds, including neoeriocitrin, neohesperidin, naringin, rutin, and neodesmin.147–149 Bergamot helps support cardiovascular health, normal lipid metabolism, and a healthy response to inflammation.149
In clinical studies, amounts of bergamot ranging from 200 to 1,500 mg daily were shown to help support certain parameters related to lipid metabolism and cardiovascular health. These include the inhibition of HMG‑CoA reductase, activation of AMPK, increases in fecal excretion of bile acids, and radical scavenging activity alongside improvements in LDL‑c.148,150
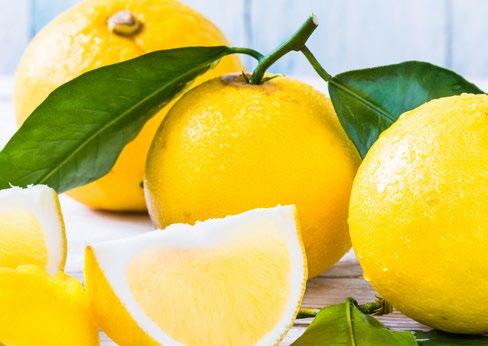
In individuals with moderate hyperlipidemia, a systematic review reported improvements in lipoprotein profiles and reductions in plasma lipids in the presence of bergamot supplementation in studies that lasted between one and six months.151
A six‑month prospective clinical trial (n=80) involved supplementation with BergaVit®, bergamot standardized to contain 150 mg of flavonoids. Decreases in total cholesterol, triglycerides, and LDL‑c, alongside increases in HDL‑c, were observed. The most significant improvements were observed in the individuals with the highest baseline cholesterol. Carotid intima‑media thickness was also reported to decrease by 25%.152
A systematic review investigated the potential of bergamot to influence lipid metabolism in humans.148 Of the 12 studies that met full eligibility criteria, 75% reported a significant decrease in lipid parameters, including total cholesterol, triglycerides, and LDL‑c cholesterol levels. Eight studies indicated an increase in HDL cholesterol levels.148
A placebo‑controlled study assessed the effects of 500 mg or 1,000 mg of daily supplementation with an extract of bergamot in individuals who had been diagnosed with hyperlipidemia for 30 days.147 Significant reductions were reported for total and LDL cholesterol levels, and for triglyceride levels. Flow‑mediated vasodilation was shown to increase significantly in both treatment arms.147
Bergamot may also improve certain parameters related to atherosclerosis.148 This is thought to be due to its supportive role for antioxidative status and the inflammatory response.148
In animal studies, ingestion of the constituents of bergamot, namely naringin and naringenin, have been exhibited in the animals with anti‑atherogenic qualities.148 Citrus flavonoids, such as those in bergamot, have been shown to potentially modulate oxidative stress through the increase of superoxide dismutase and catalase activity, and to protect plasma vitamin E.147 They also may modulate the overproduction of ROS in the vascular wall, which may modulate endothelial function. Naringenin has also been shown to significantly increase the uptake of glucose in liver and muscle cells.147 Additionally, newer research is exploring bergamot’s potential to support neurological health and other functions.153
D‑ribose
D‑ribose is a pentose carbohydrate that acts as a building block for many essential molecules in the human body including nucleotides, NADPH, nucleic acids, coenzymes, and ATP, the primary source of energy used by all cells to maintain normal health and function.154 D‑ribose synthesizes adenine nucleotides, which are required by cardiac muscle and other tissue to make ATP.154
D‑ribose has been shown in studies to support ATP recovery and function.52,154 Modern daily diets generally contain low levels of D‑ribose.154
In animal studies, supplementation with D‑ribose has been shown to elicit an increased rate of adenine nucleotide synthesis after ischemia.52 It has also been shown to improve recovery in myocardial ATP levels and functional recovery post‑ischemia. D‑ribose was also shown to improve left ventricular contractility after acute myocardial infarction in murine populations.52 One animal study assessed the effect of supplementation with D‑ribose prior to infarction and found a significant reduction in the area of infarct and significant improvement in left ventricular function six hours after the cardiac event.52
In clinical studies involving individuals with Class II‑III ischemic congestive heart failure (CHF), supplementation with D‑ribose was shown to improve diastolic dysfunction and atrial contribution to left ventricular filling.52 D‑ribose may also help individuals with Class II‑III CHF with left ventricular dysfunction improve ventilatory efficiency, maintain a maximal volume of oxygen, and improve quality of life parameters.52
Alpha‑lipoic acid
Alpha‑lipoic acid (ALA) is a molecule that acts as a cofactor for some enzymatic reactions related to blood sugar metabolism and cellular energy. It is found in the mitochondria of cells and has been shown to help support antioxidative status, a normal inflammatory response, and healthy metabolism.155
In preclinical studies, ALA has been shown to help promote healthy insulin signaling pathways. It may help support the activity of signaling molecules in the insulin/insulin‑like growth factor‑1 (IGF‑1) pathway and certain proteins such as insulin receptor substrate 1, phosphatidylinositide 3 kinase (PI3K), and protein kinase B. ALA has been shown to modulate the activity of certain pro‑inflammatory cytokines such as NF‑κB.156 It can scavenge ROS and exhibit chelating activity for certain metals.156
ALA may also help support CV health through its ability to promote antioxidative status. ALA has been shown to promote healthy levels of glutathione and Nrf2 activity.157 It also plays an important role in redox reactions related to glutathione and vitamins C and E and helps to modulate eNOS expression, which is primarily expressed in the cardiovascular system. The activity of eNOS is thought to play a role in vascular homeostasis, vasodilation, and the
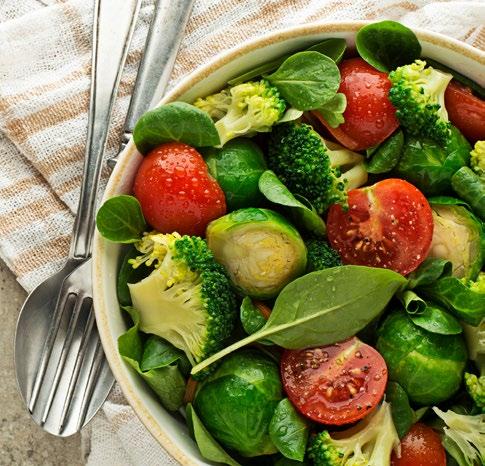
production of nitric oxide.157 Several animal studies involving hypertensive murine models have reported that ALA helped prevent increases in blood pressure.157
ALA may also support cognitive function, a healthy response to neuroinflammation, and brain health. It has been shown to increase levels of certain neurotransmitters in the brain.158
Dietary sources of ALA are fruits and vegetables such as spinach, broccoli, brussels sprouts, and tomatoes.
Selenium
Selenium is a trace mineral that supports antioxidative status and many aspects of cardiovascular health. Selenoenzymes such as glutathione peroxidase and cytosolic thioredoxin reductase help support the body’s ability to protect against oxidative damage.75 Deficiencies in selenium levels have been associated with the incidence of certain CVDs.75 Supplementation with selenium has been shown to help support total antioxidant capacity, glutathione levels, and lipid metabolism.159
A prospective randomized double‑blind placebo‑controlled trial explored the potential role of certain micronutrients in support of cardiovascular health. This study included data from 221 older adults. The treatment arm consisted of 200 mg of CoQ10 and 200 µg of selenium daily for 48 months. Quality of life and parameters related to antioxidative status and cardiovascular health were assessed. In particular, the amounts of albumin‑adjusted serum free thiols were evaluated throughout points in the study. Free thiols have been used as a biomarker for cardiovascular‑related health.75
Significant improvements in cardiovascular mortality risk and parameters related to systemic oxidative stress were observed at the study terminus. Improvements in serum free thiol amounts were reported and were correlated inversely with cardiovascular‑related risk. In particular, improvements related to antioxidative status and free thiol levels were observed to be greater in older individuals with lower selenium levels at baseline.75
Dietary sources of selenium include brazil nuts, organ meats, certain fish, grains, and dairy products.
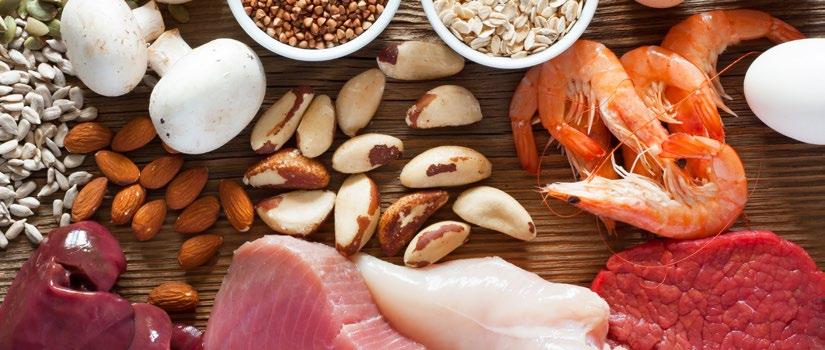
Taurine
Taurine is a conditionally essential amino acid that supports overall metabolic health.160 As the endogenous synthesis of taurine can be relatively low, dietary intake is the primary way to maintain healthy taurine status.160 However, taurine only occurs naturally in animal‑based foods like dairy, eggs, meat, and seafood.161 While plant‑based diets are recognized to help promote metabolic health, vegetarians and vegans are commonly at risk of having suboptimal taurine intake.162 Epidemiological studies reveal that a low dietary intake of taurine is associated with higher cardiovascular risks.160 Consequently, taurine supplementation may benefit the general population, particularly those adhering to a primarily plant‑based diet.
Taurine promotes metabolic health by regulating glucose and lipid metabolism, energy production, and supporting exercise performance.163 Taurine also displays antioxidant and anti‑inflammatory properties, and it is required for calcium homeostasis, osmoregulation, cell membrane stabilization, and bile acid conjugation.164
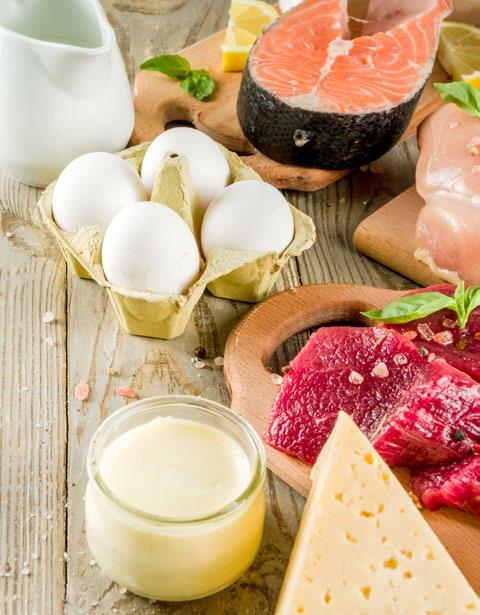
Many animal models demonstrate that taurine can help promote normal cholesterol and triglycerides status, blood glucose metabolism, and healthy blood pressure.164 Due to taurine’s widespread function in metabolic homeostasis, recent research has examined the potential clinical benefits of taurine in supporting those with metabolic syndrome, CVD, and diabetes.163–165
A worldwide epidemiological WHO‑CARDIAC study surveyed 3,960 individuals from 41 populations.166 The survey results concluded that the dietary intake of taurine had a significantly inverse relationship to the values of blood pressure, total cholesterol, and atherogenic index. Other studies have observed that patients with type 2 diabetes have significantly lower plasma taurine levels compared to non‑diabetic patients, where one study observed a 25% decrease.163,167
Furthermore, a meta‑analysis of 12 peer‑reviewed studies concluded that patients with liver or metabolic dysfunction (diabetes, hepatitis, fatty liver, cystic fibrosis, chronic alcoholism, and cardiac surgery) who received taurine supplementation for 15 days to six months displayed significantly lower SBP, DBP, total cholesterol, and triglycerides163.
A systematic review and meta‑analysis found beneficial results regarding taurine’s effect on supporting healthy glucose metabolism. The researchers analyzed five randomized clinical trials with 209 patients with diabetes.163 Compared to the control groups, the experimental groups who received taurine supplementation exhibited significantly reduced HbA1C, fasting blood sugar, and HOMA‑IR.163 However, further studies are warranted due to the small number of trials and small sample sizes.
Micronutrients to Support Cardiovascular Health
One proposed way taurine may promote optimal glucose regulation is through its demonstrated antioxidant and anti‑inflammatory properties.164 High glucose levels or hyperglycemia are associated with increased production of ROS, which can play a vital role in the adverse consequences of diabetes. Rodent models reveal that taurine can help mitigate the effects of oxidative stress by reducing ROS production and activity. This is likely due to taurine’s role in increasing the antioxidant activity of superoxide dismutase, glutathione peroxidase, and catalase, and helping mitigate lipid peroxidation.164 Likewise, inflammation plays a role in the development of diabetes. Taurine has been shown to promote healthy inflammatory responses by helping reduce the secretion of inflammatory mediators like TNF α, prostaglandin E2, and IL‑6.164 Supporting optimal taurine status through animal‑based food or supplementation may help promote healthy aging and cardiovascular health.168
Nicotinamide riboside
Nicotinamide riboside (NR) is a precursor of vitamin B3 (niacin) and is a building block for NAD+.56,169 NAD+ is a coenzyme involved in more than 500 biochemical reactions including those related to cellular energy production, DNA repair, mitochondrial function, and stress response. Recent research indicates that supplementation with NR may promote healthy NAD+ status and cellular energy. NR may also help support normal sirtuin function and cardiovascular health during the aging process.56,169
A randomized crossover controlled clinical trial explored the efficacy of supplementation with NR on sixty individuals between 55 and 79 years of age.170 A preliminary treatment period consisted of 500 mg twice‑daily supplementation with NR as Niagen® for six weeks. NR was shown to increase NAD+ levels in peripheral blood mononuclear cells by approximately 60% as compared to placebo. Increases in metabolites related to cellular energy production and metabolism such as ATP were also reported.170
Changes related to cardiovascular function were also observed. In individuals with stage 1 hypertension, the NR treatment group was shown to have a mean 9 mmHg lower SBP when compared to similar individuals receiving placebo.170 NR was also shown to help reduce mean carotid‑femoral pulse wave velocity, particularly in individuals with higher baseline blood pressure.170 No serious adverse effects were reported in the trial. NR was also reported to be well‑tolerated and no niacin‑related flushing was observed.
NR has been shown to promote healthy respiratory function, help reduce mitochondrial ROS production, and reduce IL‑1β levels in peripheral blood mononuclear cells.171 NR has also been shown to influence IL‑6 levels; upregulated IL‑6 levels are associated with chronic heart failure (HF) and acute myocardial infarction.171 A clinical trial involving 19 patients with stage D HF and a mean left ventricular ejection fraction of 20% and 19 healthy individuals explored the efficacy of NR supplementation. In five hospitalized patients, doses of NR escalated from 250 mg twice daily to 1,000 mg twice daily starting on day three. The study lasted from five to nine days. Study results indicated that supplementation with NR helped support decreased levels in IL‑6 and IL‑1β and helped support mitochondrial respiration in the hospitalized patients with HF.171
NR may also support cellular respiration and mitochondrial function. A four‑week animal study assessed the efficacy of NR supplementation on Sco2 KIKO mice.172 Sco2 is a protein essential for the functioning of cytochrome c oxidase, an enzyme involved in the mitochondrial
respiratory chain.173 The administration of NR resulted in increases in NAD+ and the activation of Sirt1. The transcription of genes related to oxidative phosphorylation were increased along with increases in mitochondrial respiratory chain activity and oxidative metabolism.172
Carnitine
L‑carnitine is an amino acid derivative found in most tissues in the human body.54 Although it can be obtained from dietary sources, it is considered a conditionally essential nutrient under conditions when intracellular levels are low, such as in the elderly population, premature infants, and in the presence of certain genetic conditions, mitochondrial dysfunction, and diabetes.54 Short‑ and medium‑chain fatty acids can enter mitochondria by diffusion, but long‑chain fatty acids require the carnitine shuttle to facilitate fatty acid transportation.54 The carnitine shuttle is critical for the prevention of the buildup of potentially harmful long‑chain substrates and acyl‑coenzyme A.53
The acetyl derivative of L‑carnitine, ALC, participates in glycogen synthesis, glucose metabolism modulation, increasing plasma ATP concentration, and neurological function.174 In particular, ALC facilitates cholinergic neurotransmission both directly and by providing an acetyl group for acetylcholine synthesis.53
Carnitine helps support the transference of toxic compounds out of the mitochondria.53 It can also support brain health; ALC passes through the blood‑brain barrier at greater efficiency than L‑carnitine.174 ALC has been shown in research to support the body’s response to oxidative stress and inflammation.174 Supplementation with L‑carnitine has been shown to activate the Nrf2 pathway, increase the activity of enzymes related to antioxidative status, and help support the body’s response to certain CV events related to myocardial ischemia.175,176
A systematic review and meta‑analysis involving data from over 1,500 participants reported improvements in total cholesterol, LDL‑c, HDL‑c, fasting blood glucose, HbA1c, and HOMA‑IR values in the presence of L‑carnitine supplementation, particularly when daily therapeutic amounts were at or above 1,500 mg daily.177 A similar study reported improvements in plasma lipoproteins related to atherogenic qualities.178
Carnitine is thought to have a protective effect against muscle disruption after strenuous exercise.55 It may help attenuate the release of creatine kinase and myoglobin.55 It may also help improve oxidative status and the protein signaling important for exercise recovery.55
A clinical trial involving supplementation with 4 g of carnitine daily for two weeks in competitive walkers reported improvements in certain exercise‑related parameters including the maximum rate of oxygen consumption (VO2max).55 Other studies involving high‑performance athletes produced similar results.55 One study in junior athletes involved 1 g of carnitine supplementation for six weeks and reported higher athletic performances. In untrained athletes, study participants experienced slight improvements in exercise performance in a study involving 2 g of carnitine daily for two weeks.55
It’s important to note that conflicting results may exist among emerging clinical evidence regarding carnitine’s potential impact on cardiovascular health. More evidence is needed before clinical conclusions can be made; caution and additional research are recommended to the general practitioner.
Nattokinase
Nattokinase is a proteolytic enzyme found in natto, a Japanese fermented soy product known for its protective effects on the cardiovascular system.179 Proteolytic enzymes are a class of enzymes that break down proteins into individual amino acids and are found in every body system.102 Research suggests that nattokinase may help support the body’s ability to break down and remove damaged cellular debris and specific protein structures that are produced during the natural inflammatory process in response to trauma or injury.102
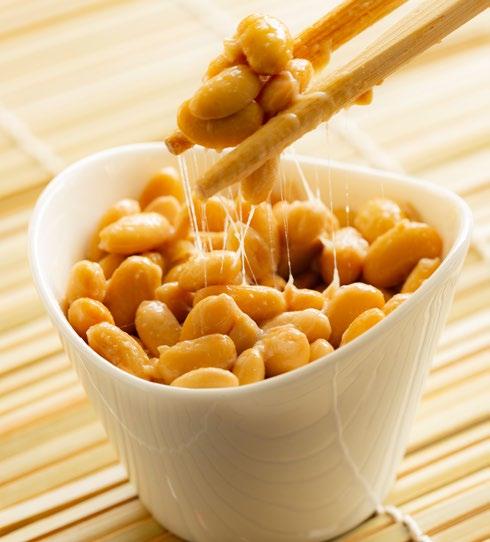
Nattokinase has been shown to act in multiple pathways in the coagulation cascade and directly help degrade fibrin and dissolve thrombi.102 It may help inhibit plasminogen activator‑inhibitor‑1 (PAI‑1) and increase the activity of tissue plasminogen activator (t‑PA), helping to drive the conversion of plasminogen to plasmin and prourokinase to urokinase.179 Plasmin and urokinase are enzymes that play important roles in the fibrinolytic system, which is crucial for maintaining cardiovascular health. Nattokinase also helps support the inhibition of platelet aggregation by blocking thromboxane formation.180 It has also been shown to help suppress intimal thickening of blood vessel walls through its support of antioxidative status and anti‑apoptotic effects.179 It has also been shown to help support lipid metabolism through the inhibition of LDL‑c oxidation and the reduction of lipid peroxidation.179
A twelve‑month clinical study involving 1,062 participants explored the potential role of nattokinase in individuals with elevated lipid levels and atherosclerotic changes.102 At the end of the treatment period, the group that received 10,800 FU of nattokinase daily showed significant improvements in triglycerides (15.3%), LDL‑c (18.1%), and total cholesterol (15.9%). Significant reductions in common carotid artery‑intima media thickness (CCA‑IMT) and carotid plaque sizes were also observed. Synergistic effects were observed in the coadministration of vitamin K2 and nattokinase.102
A subgroup of participants received 3,600 FU nattokinase daily. Nattokinase alone at this amount was not found to statistically improve lipid amounts or atherosclerotic progression. The study designers considered lifestyle factors in their statistical analysis. They observed greater improvements in lipid status and atherosclerotic changes in smokers and individuals who regularly consume alcohol. In addition, comparatively larger improvements in cardiovascular changes were observed in individuals who exercised regularly.102
Flavonoids
Flavonoids are a diverse group of natural compounds belonging to the polyphenol family. In the human diet, flavonoids are commonly consumed through fruits, vegetables, tea, cocoa, and other plant‑based foods.181 They are an important component of many diets featuring a high intake of plant‑based food, including the Mediterranean diet. Flavonoids are categorized into different subclasses including flavonols, flavones, flavanones, flavan‑3‑ols, anthocyanins, and isoflavones based on their chemical structure. They have been shown to support many aspects of human health including the body’s response to oxidative stress.181 Other recent studies have explored the role of flavonoids in cardiovascular and neuronal health, cognitive function, and mood support.181
Dark berries
As mentioned in the following section “Synergistic nitric oxide support”, fruits such as blueberries, bilberries, and cherries may help promote antioxidative status, nitric oxide homeostasis, and other parameters related to cardiovascular health.32,33,182,183 Dark berries contain anthocyanins, a group of flavonoids that give plants their dark blue or purple pigments, which have been shown to support cellular health, antioxidative status, and mitochondrial health.184 Clinical studies have suggested that daily consumption of blueberries and other dark berries may help promote healthy blood pressure and arterial elasticity.182,183 Results from an RCT indicate that daily supplementation with blueberry for one month increased certain parameters related to endothelial function including flow‑mediated dilation.185 Improvements in other biomarkers related to cardiovascular health were also observed.185
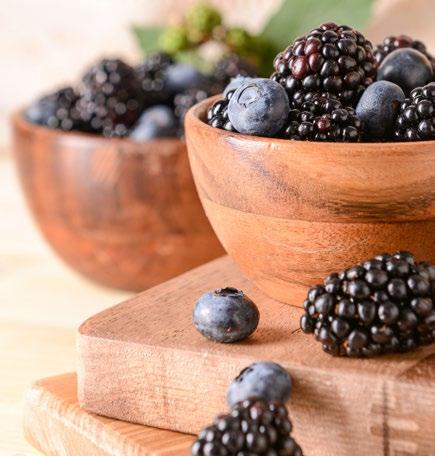
The phenolic compounds in dark berries are also thought to act as prebiotics.186 Prebiotics are compounds that help stimulate the activity of the GI microbiome.187 Research indicates that blueberries may significantly alter the gut microbial composition.186 They may also help support the function of SCFA‑producing bacteria. In a laboratory study assessing the potential impact of eight types of berries on the gut microbiome, blueberries demonstrated the highest abundance of anthocyanins.186 Blueberries were also shown to help increase the populations of SCFA‑producing bacteria including Prevotella and Barnesiella genera.186 Of note, the authors of the laboratory study report that the eight types of berries tested all had different impacts on the microbiome.186
Cocoa
Cocoa extracts have been a topic in recent research because of their relatively high flavanol content and for their potential to support cardiovascular health.188 They have been shown to support the inflammatory response, platelet activation, and vascular function.188 A randomized double‑blind placebo‑controlled clinical trial explored the efficacy of supplementation with
Micronutrients to Support Cardiovascular Health
cocoa extract and multivitamins on outcomes related to CVD.188 The trial involved daily supplementation with 500 mg of cocoa flavanols including 80 mg of epicatechin. Over twenty thousand individuals over the age of 60 were randomized to this four‑arm study: cocoa extract plus a multivitamin, cocoa extract plus placebo, multivitamin plus placebo, and placebo‑placebo. The median follow‑up for this study was 3.6 years. Outcomes related to cardiovascular events such as myocardial infarction, stroke, cardiovascular death, carotid artery disease, and unstable angina were assessed.188 The authors conclude that supplementation with cocoa extract did not significantly reduce the total amount of cardiovascular events among older adults. However, when compared to placebo, cocoa extract was shown to reduce the primary outcome of total cardiovascular events among compliant individuals.188
Synergistic nitric oxide support
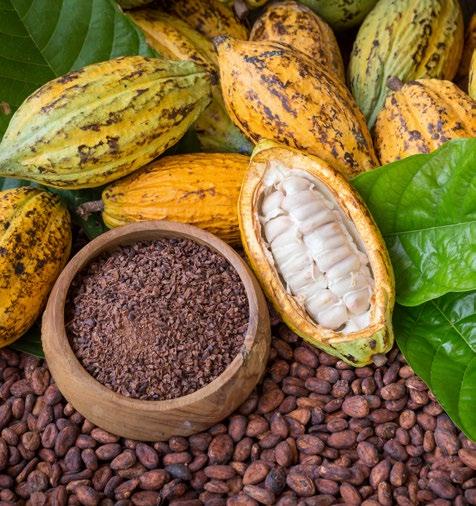
This section features the latest research on the synergy between the phytonutrients, vitamins, and minerals that comprise the novel formula Vascanox HP®.* This comprehensive proprietary formula combines beet extract and black garlic extract (sources of dietary nitrates and hydrogen sulfide), along with various berry extracts, vitamins, and minerals. It is designed to support multiple NO pathways in the body, particularly the enterosalivary pathway, which promotes NO production, storage, and bioavailability.* Although NO status may be supported through a nitrate‑rich diet filled with beetroot and leafy greens, modern dietary habits and the variable nutrient density of fruits and vegetables may make Vascanox HP® an ideal adjunct to healthy eating and lifestyle habits.*
The body utilizes two major pathways that work in tandem to maintain healthy NO homeostasis: the endogenous pathway and the enterosalivary pathway.23 The micronutrients featured in Vascanox HP® complement the endogenous NO production by providing comprehensive support for the enterosalivary pathway.23 While the endogenous pathway can decline with age, the enterosalivary route does not and can be supported by dietary or supplemental intake of nitrates.27
An open‑label clinical trial with 12 hypertensive participants aged 52 to 73 examined the effects of Vascanox HP® (2 capsules per day for four weeks) on NO bioavailability and BP.23 Salivary NO2‑, a surrogate of NO bioavailability, and NO3‑ were assessed routinely using saliva NO test strips. The BP of participants was measured at baseline, two weeks, and four weeks. After four weeks of Vascanox HP® supplementation, all participants exhibited a decrease in SBP by ~11 mmHg (p < 0.001) at two weeks, which persisted until the end of the trial. DBP decreased up to 11 mmHg (p < 0.01) in the patients with elevated DBP, but no decrease was observed in the participants with normotensive DBP. All 12 participants had consistent, rapid increases
of salivary NO2‑ concentrations (from fourfold to 44‑fold times higher than baseline) at two hours post‑administration, which slowly decayed but remained above baseline in all participants after 24 hours.23 This suggests that Vascanox HP® may promote sustained, healthy NO status potentially up to 24 hours a day for longer‑lasting clinical benefits.* However, these are preliminary results, and further research is required on this subject.
Various clinical trials have further investigated the general clinical benefits of dietary nitrates to promote healthy BP.28,189 A meta‑analysis of 16 RCTs with 254 participants examined the effects of dietary nitrate (between 157 mg and 1,488 mg per day) on BP. The researchers concluded that inorganic nitrate and beetroot juice consumption was associated with a statistically significant 4.4 mmHg reduction in SBP, with a non‑significant, modest 1.1 mmHg decrease in DBP.189
Other essential nutrients that may synergistically help support normal BP include magnesium, zinc, potassium, vitamin C, vitamin D, and B vitamins.* One systematic review suggests that vitamin D may synergistically work with magnesium to promote a healthy BP in individuals.190
Vitamin D and vitamin K may also work synergistically, as covered in their section above.
B vitamins, including thiamine and vitamin B12, may support endothelial health and normal BP.191,192 Thiamine may modulate eNOS‑dependent NO production.191,192 In rodent models, a thiamine deficiency led to reduced NO production and increased vascular dysfunction.192 A systematic review and meta‑analysis of 9 RCTs observed that individuals receiving zinc supplementation had reduced SBP compared to a control.193
Polyphenolic fruits, such as blueberries and bilberry, may serve as a source of eNOS‑activating compounds and promote antioxidant status.32,33 Preliminary evidence suggests that berries may also help promote NO production in the stomach and help support the enterosalivary pathway.31–33 More research is needed before clinical conclusions can be made.
Vitamin C may help support vascular health and work synergistically to support NO homeostasis.194–196 Vitamin C is necessary for collagen synthesis, stimulating endothelial proliferation, inhibiting apoptosis, and scavenging free radicals.194 As a potent antioxidant, vitamin C may help attenuate the oxidative stress and inflammation associated with vascular dysfunction.194 Moreover, vitamin C helps the conversion of nitrite to NO in the enterosalivary pathway in the stomach, thus helping to promote NO production, recycling through the oral microbiome, and bioavailability.195,196 Vitamin C, either alone or in combination with nitrate consumption, has been shown in clinical trials to support NO production, healthy BP, and endothelial health in older adults and in individuals with hypertension, type 2 diabetes mellitus, or hypercholesterolemia.195–198
Potassium in the form of potassium nitrate may also support NO homeostasis.* Low potassium intake may be associated with unhealthy BP, especially when combined with high sodium intake.199–201 A meta‑analysis of 15 RCTs (n = 917) concluded that both normotensive and hypertensive individuals receiving potassium supplementation had improved BP, with the effect being more pronounced in those with hypertension. Reduced BP was correlated significantly with decreased urinary sodium‑to‑potassium ratio and increased urinary potassium.199 Similar findings were observed in a separate meta‑analysis of 23 RCTs (n = 1,213), which found that groups who supplemented with potassium experienced a modest but significant reduction in BP in patients with primary hypertension.200 The effects may be more pronounced in subjects whose baseline dietary potassium intake is low.201
Recent literature has explored the role of hydrogen sulfide (H2S), a gaseous transmitter, in promoting healthy NO homeostasis and vasodilation. H2S can be produced endogenously or
obtained dietarily. Compounds in garlic, including allicin and S‑allyl‑L‑cysteine, act as H2S donors, which is why black garlic extract is featured in Vascanox HP®. H2S may promote NO synthesis through eNOS‑dependent and enterosalivary pathways to maintain elevated cGMP levels in several ways. First, it may promote eNOS function and the bioavailability of NO‑derived cGMP. Second, H2S may promote the conversion of nitrite into NO by shifting the activity of xanthine oxidoreductase (XOR) enzymes to nitrite reductases to form NO. Third, H2S may also interact with oxidized forms of NO to produce stable intermediates that serve as reservoirs for NO, such as in the muscles.23
Due to the integral role of certain commensal oral bacteria in the enterosalivary pathway by reducing salivary nitrate to nitrite, researchers have found an association between an unhealthy oral microbial environment and the potential influence for suboptimal NO.23, 202‑205 In the last decade, various studies have explored the effects of antibacterial mouthwashes on NO production and, subsequently, healthy blood pressure.204,205 Chlorhexidine is the most widely studied antibacterial agent in mouthwashes.204 Some researchers postulate that, as approximately 99% of mouthwash contains an antibacterial agent, any type/brand can potentially harm nitrate‑reducing bacteria.202 However, further research is needed on other over‑the‑counter mouthwashes as their effects are unknown.204
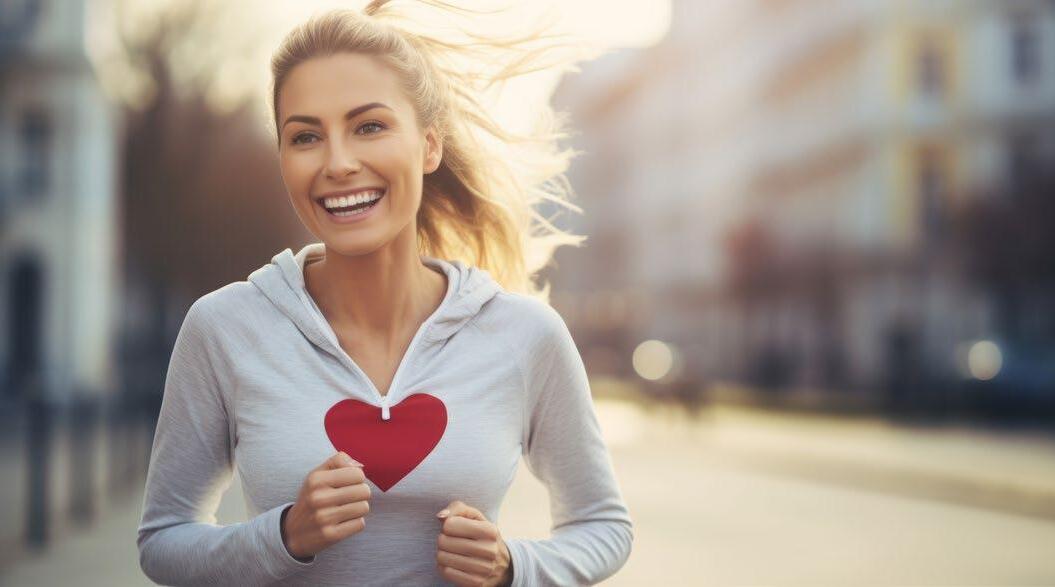
Diet and Lifestyle Recommendations
The importance of dietary and lifestyle interventions for the prevention of cardiovascular disease has been well established in research and cannot be overstated. While there exist many dietary interventions, this section will highlight those with a considerable amount of research related to the support of cardiovascular health. Also included in this section are general lifestyle recommendations and considerations related to toxin exposure.
Mediterranean‑style diet
Several diets have been studied extensively for their supportive role in CVD: the DASH diet, the OmniHeart diet, and the Mediterranean‑style diet. Of these, the Mediterranean‑style diet will be featured here due to the considerable amount of research on this topic. Evidence indicates that the Mediterranean diet helps support antioxidative status, the body’s response to inflammation, cardiovascular function, immune health, the gut microbiome, and the body’s detoxification pathways.206‑208
The Mediterranean diet reflects food patterns from regions of Greece, especially Crete, and southern Italy. It has been recognized by UNESCO for its cultural heritage and because of the innate environmental responsibility of its agricultural and dietary practices.208 The diet consists mainly of a high intake of plant foods including fruits and vegetables, minimally processed grains, fish, nuts, and extra virgin olive oil, and is typically low in saturated fats. In addition to phytonutrients, the diet also includes a high intake of dietary fiber – a key nutrient to support
Diet and Lifestyle Recommendations
cardiovascular health – and flavonoids. The Mediterranean diet also features seasonally fresh and locally grown foods, and a social component to eating patterns.208
The impact of the social component to the Mediterranean diet is currently not well known in research. An animal study explored the potential effect of the Mediterranean diet (ingredients alone) on sympathetic activity and the response to psychological stress when compared to the Western diet. The Mediterranean diet was shown to help support more rapid recovery from acute psychological stress. Delays in age‑related increases in sympathetic activity and cortisol responses were observed in the presence of the Mediterranean diet. This suggests that the ingredients in the Mediterranean diet may help support normal autonomic regulation and the ability to rebound from acute stress.209
The evidence specific to the Mediterranean diet’s impact on cardiovascular health is vast. An umbrella review of meta‑analyses reported improvements in inflammatory status including reductions in CRP and IL‑6 alongside improvements in total cholesterol and increases in HDL‑c in individuals on the Mediterranean diet when compared to controls.208 Another meta‑analysis involving data from over 7,000 participants reported reductions in SBP and DBP in individuals on the Mediterranean diet for at least 1 year.208
An RCT involving almost 1,000 participants explored the Mediterranean diet’s potential to reduce the progression of atherosclerosis in individuals with coronary heart disease. The control diet consisted of a low‑fat diet. After five years, reductions in intima‑media thickness of both common carotid arteries (IMT‑CC) were observed in the presence of the Mediterranean diet. These reductions were maintained at the seven‑year‑mark. No reductions in IMT‑CC were observed in the controlled low‑fat diet.210
The Mediterranean diet correlates with the longevity observed in the Blue Zones found in Sardinia and the Greek island of Ikaria. However, diet is only one contributing factor to the longevity of those living in Blue Zones.211 Evidence suggests that food patterns such as the use of local foods, the combination of certain ingredients, and increased physical activity may promote longevity in Blue Zone regions.211 For more information, a compelling review written by Pes and colleagues further elucidates research on this topic.211
Other CV‑related diets
DASH
DASH stands for “Dietary Approach to Stop Hypertension.” The diet was designed by the National Institutes of Health and intended as an accessible, easy‑to‑follow plan for most Americans to help support healthy blood pressure. It consists of a diet rich in fruits, vegetables, whole grains, and low‑fat dairy foods. It limits red meat, sugar‑sweetened foods, and added fats, while increasing the essential electrolyte minerals potassium, magnesium, and calcium. Reductions in SBP and DBP have been observed in clinical studies related to the DASH diet. One controlled study reported reductions of SBP by 2.8 mm Hg and DBP by 1.1 mm Hg when compared with the control diet.212 However, some limitations to this diet exist, which include a relatively high amount of carbohydrate and dairy intake. The DASH diet is still being researched for its potential to support additional functions within the body. For example, a recent study explored its potential to help lower serum urate among adults with hyperuricemia.213
OmniHeart
The OmniHeart diet is a variation of the DASH diet featuring a higher intake of protein and a lower carbohydrate intake. Clinical evidence suggests it may be as or more efficacious than the DASH diet to support healthy blood pressure and lipid metabolism.214‑216
Sodium intake
The DASH and OmniHeart diets are not exclusively sodium‑restrictive diets. Clinical evidence suggests that a combination of DASH plus sodium restriction may have a synergistic effect.217
Lifestyle recommendations
Smoking and vaping
Smoking is considered to be the number one cause of cardiovascular diseases, and smoking cessation is associated with improved prognosis of CAD.50,218 E‑cigarette use (vaping) is popular with never‑smokers and is the most commonly used tobacco product in youth.3 A 2020 survey indicated that almost 20% of US high school students and 4.7% of middle school students reported the use of electronic cigarettes.219 In another study, ever‑use of e‑cigarettes was almost 30% in high school students, and 7% in middle schoolers.3 In adults, current e‑cigarette use was reported to be almost 4%, with the highest prevalence among individuals ages 18 to 24 (9.4%).3
Vaping has been shown to be detrimental to many cardiovascular structures and processes; it causes oxidative stress, damage to the cardiac endothelium, and many other potential harmful downstream effects still being elucidated as vaping and its users age.219 A review by Echeagaray and colleagues titled “Cardiovascular consequences of vaping” is a comprehensive resource on the subject and is highly recommended for more information.219
Physical activity
Physical inactivity is a major risk factor for many CVDs. A comprehensive meta‑analysis of 43 studies reported a median relative risk of coronary artery disease associated with physical inactivity of 1.9 (range: 1.5 to 2.4).220 Physical activity is critical to maintaining cardiovascular health; a vast body of research supports the recommendation to engage in regular exercise for the prevention of CVD.38,220
A clinical study reported that resistance exercise (as strength training) promotes decreases in total cholesterol, triglycerides, and LDL‑c levels while increasing HDL‑c concentrations.221 As reported in the vitamin D and K section of this document, aerobic training, either alone or in combination with vitamin D, may support a normal inflammatory response, healthy glucose metabolism, and antioxidative status.133
Research indicates that physical activity may also support mitochondrial health. Endurance exercise has been shown to help support mitochondrial biogenesis in brain tissue and skeletal
muscle through the activation of the peroxisome proliferator‑activated receptor‑γ (PPAR‑γ) coactivator 1α (PGC‑1α), and other biochemical pathways.222
Because every person has individual needs and abilities, no one exercise program can be recommended. However, general recommendations for able‑bodied individuals include a combination of both aerobic and strength training exercises.38 For more information on this topic, a 2022 review by Franklin and colleagues explores in detail research pertaining to cardiorespiratory fitness, goal intensities for exercise training, and the needs of special patient populations.220
Stress reduction
Stress management techniques, such as meditation, yoga, or similar mindfulness practices may help promote certain aspects of cardiovascular health. Acute and chronic stress may trigger acute cardiac events or worsen heart function and metabolism in CAD.51,223 In addition, research indicates that autonomic imbalances and sympathetic overactivation may play a role in the pathogenesis of hypertension.224
Research suggests that poor heart rate variability (HRV) may be an indicator of sympathetic overactivation. The monitoring of HRV may help support healthy stress management and normal cardiac function.224 As mentioned previously, the Mediterranean diet may help support the body’s response to psychological stress and may help modulate autonomic imbalances.209
For more information on autonomic imbalances and HRV, consider exploring the following books: The Polyvagal Theory by Stephen W. Porges, PhD, and The Clinical Handbook of Biofeedback by Inna Z. Khazan, PhD.
Toxin exposure
Exposure to environmental toxins including heavy metals such as lead, manganese, and chromium, as well as substances like bisphenol A (BPA) and other polycarbonates, may negatively influence cardiovascular function.225‑227 For example, adult BPA exposure has been linked to a more frequent diagnosis of certain cardiovascular diseases including angina, coronary heart disease, and hypertension. Elevated urinary BPA levels have been significantly associated with the prevalence of peripheral artery disease.227 BPA exposure has also been linked to abnormalities in HRV.227 BPAs are also associated with the incidence of diabetes; elevated total urinary BPA has been significantly associated with T2DM.227
Evidence indicates that heavy metal exposure has been associated with the incidence of hypertension.225,226 A population‑based cohort study involving over 4,000 individuals found significantly higher SBP and DBP and increased prevalence of hypertension in individuals with blood lead in the fourth quartile.226 In another study, the risk of hypertension in individuals with the highest levels of chromium exposure was 1.85 times higher than those with the lowest chromium exposure.225
The research above only begins to uncover the intricacies and downstream effects of toxin exposure. For additional insights, The Toxin Solution by Joseph Pizzorno, ND, is a significant resource on this topic and comes highly recommended for practitioners seeking to support cardiovascular health and beyond.
Conclusion
In the arc of clinical research specific to cardiovascular health and functional medicine, there exist some important gaps that are worth addressing. Currently, many clinical studies on phytonutrients tend to be on a small scale and contain data from homogeneous demographics (either in age, race, socioeconomic status, gender, geolocation, or a combination of these). In addition, many studies on the micronutrients discussed in this document contain differences in treatment arms (either therapeutic amounts or combinations of ingredients), placebos, and other critical aspects of study design. This makes the aggregation of data through systematic review and meta‑analysis challenging if not impossible.
By nature, it is difficult and costly to design, recruit for, and run clinical research, and these data gaps are often a reflection of this. However, one strength of functional medicine to overcome this is its thorough consideration of the individual. Personalized recommendations are the backbone of good health care. Through this document, we hope you have gained the knowledge to further your ability to meet the needs of your patients on an individualized level.
Appendices
References
ATP: Adenosine triphosphate
CFU: Colony forming unit
CRP: C‑reactive protein
DBP: Diastolic blood pressure
DHA: Docosahexaenoic acid
EGX: Endothelial glycocalyx
eNOS: Endothelial nitric oxide synthase
EPA: Eicosapentaenoic acid
HDL‑c: High‑density lipoprotein‑cholesterol
HOMA‑IR: Homeostatic Model Assessment for Insulin Resistance
hsCRP: High‑sensitivity C‑reactive protein
IL: Interleukin
iNOS: Inducible nitric oxide synthase
LDL‑c: Low‑density lipoprotein‑cholesterol
MDA: Malondialdehyde
NAD+: Nicotinamide adenine dinucleotide
NADPH: Nicotinamide adenine dinucleotide phosphate
NF‑κB: Nuclear factor κ‑B
NO: Nitric oxide
NOX: NADPH oxidase
NR: Nicotinamide riboside
Nrf2: Nuclear factor erythroid 2–related factor 2
PUFA: polyunsaturated fatty acid
RAAS: Renin‑angiotensin‑aldosterone system
RCT: Randomized controlled trial
ROS: Reactive oxygen species
SBP: Systolic blood pressure
T2DM: Type 2 diabetes mellitus
TNF‑α: Tumor necrosis factor‑α
Bibliography
1. Zhai T, Wang P, Hu X, Zheng L. Probiotics bring new hope for atherosclerosis prevention and treatment. Oxid Med Cell Longev. 2022;2022:3900835. doi:10.1155/2022/3900835
2. Steven S, Frenis K, Oelze M, et al. Vascular inflammation and oxidative stress: major triggers for cardiovascular disease. Oxid Med Cell Longev. 2019;2019:7092151. doi:10.1155/2019/7092151
3. Tsao CW, Aday AW, Almarzooq ZI, et al. Heart disease and stroke statistics—2023 update: a report from the American Heart Association. Circulation. 2023;147(8):e93-e621. doi:10.1161/CIR.0000000000001123
4. Shaito A, Aramouni K, Assaf R, et al. Oxidative stressinduced endothelial dysfunction in cardiovascular diseases. Front Biosci Landmark Ed. 2022;27(3):105. doi:10.31083/j. fbl2703105
5. Andrés CMC, Pérez de la Lastra JM, Andrés Juan C, Plou FJ, Pérez-Lebeña E. Superoxide anion chemistry — its role at the core of the innate immunity. Int J Mol Sci 2023;24(3):1841. doi:10.3390/ijms24031841
6. Papaconstantinou J. The role of signaling pathways of inflammation and oxidative stress in development of senescence and aging phenotypes in cardiovascular disease. Cells. 2019;8(11):1383. doi:10.3390/cells8111383
7. Kumar H, Dhalaria R, Guleria S, et al. Anti-oxidant potential of plants and probiotic spp. in alleviating oxidative stress induced by H2O2 Biomed Pharmacother. 2023;165:115022. doi:10.1016/j.biopha.2023.115022
8. Minich DM, Brown BI. A review of dietary (phyto)nutrients for glutathione support. Nutrients. 2019;11(9):2073. doi:10.3390/nu11092073
9. Iskusnykh IY, Zakharova AA, Pathak D. Glutathione in brain disorders and aging. Molecules. 2022;27(1):324. doi:10.3390/molecules27010324
10. Raghu G, Berk M, Campochiaro PA, et al. The multifaceted therapeutic role of N-acetylcysteine (NAC) in disorders characterized by oxidative stress. Curr Neuropharmacol. 2021;19(8):1202-1224. doi:10.2174/157015 9X19666201230144109
11. Mardani N, Mozafarpoor S, Goodarzi A, Nikkhah F. A systematic review of N-acetylcysteine for treatment of acne vulgaris and acne-related associations and consequences: focus on clinical studies. Dermatol Ther 2021;34(3). doi:10.1111/dth.14915
12. Frijhoff J, Winyard PG, Zarkovic N, et al. Clinical relevance of biomarkers of oxidative stress. Antioxid Redox Signal 2015;23(14):1144-1170. doi:10.1089/ars.2015.6317
13. Ayala A, Muñoz MF, Argüelles S. Lipid peroxidation: production, metabolism, and signaling mechanisms of malondialdehyde and 4-hydroxy-2-nonenal. Oxid Med Cell Longev. 2014;2014:360438. doi:10.1155/2014/360438
14. Ashrafizadeh M, Ahmadi Z, Mohammadinejad R, Farkhondeh T, Samarghandian S. Curcumin activates the Nrf2 pathway and induces cellular protection against oxidative injury. Curr Mol Med. 2020;20(2):116-133. doi:10.21 74/1566524019666191016150757
15. Ma Q. Role of Nrf2 in oxidative stress and toxicity. Annu Rev Pharmacol Toxicol. 2013;53:401-426. doi:10.1146/ annurev-pharmtox-011112-140320
16. Li YR, Li S, Lin CC. Effect of resveratrol and pterostilbene on aging and longevity. Biofactors. 2018;44(1):69-82. doi:10.1002/biof.1400
17. Teimouri M, Homayouni-Tabrizi M, Rajabian A, Amiri H, Hosseini H. Anti-inflammatory effects of resveratrol in patients with cardiovascular disease: a systematic review and meta-analysis of randomized controlled trials. Complement Ther Med. 2022;70:102863. doi:10.1016/j. ctim.2022.102863
18. Maselli Del Giudice A, La Mantia I, Barbara F, et al. Use of nutraceuticals in elderly to fight inflammation and immunosenescence: a randomized case-control study. Nutrients 2022;14(17):3476. doi:10.3390/nu14173476
19. Calder PC, Ortega EF, Meydani SN, et al. Nutrition, immunosenescence, and infectious disease: an overview of the scientific evidence on micronutrients and on modulation of the gut microbiota. Adv Nutr. 2022;13(5):S1S26. doi:10.1093/advances/nmac052
20. Hang R, Wang J, Tian X, et al. Resveratrol promotes osteogenesis and angiogenesis through mediating immunology of senescent macrophages. Biomed Mater 2022;17(5). doi:10.1088/1748-605X/ac80e3
21. Yousefzadeh MJ, Zhu Y, McGowan SJ, et al. Fisetin is a senotherapeutic that extends health and lifespan. EBioMedicine. 2018;36:18-28. doi:10.1016/j. ebiom.2018.09.015
22. Csekes E, Račková L. Skin aging, cellular senescence and natural polyphenols. Int J Mol Sci. 2021;22(23):12641. doi:10.3390/ijms222312641
23. Houston M, Chen C, D’Adamo CR, Papathanassiu AE, Green SJ. Effects of s-allylcysteine-rich garlic extract and dietary inorganic nitrate formula on blood pressure and salivary nitric oxide: an open-label clinical trial among hypertensive subjects. Cureus. 2023;15(9):e45369. doi:10.7759/ cureus.45369
24. Shiraseb F, Asbaghi O, Bagheri R, Wong A, Figueroa A, Mirzaei K. Effect of L-arginine supplementation on blood pressure in adults: a systematic review and dose-response meta-analysis of randomized clinical trials. Adv Nutr 2022;13(4):1226-1242. doi:10.1093/advances/nmab155
25. Sverdlov AL, Ngo DTM, Chan WPA, Chirkov YY, Horowitz JD. Aging of the nitric oxide system: are we as old as our NO? J Am Heart Assoc. 2014;3(4):e000973. doi:10.1161/ JAHA.114.000973
26. Guizoni DM, Vettorazzi JF, Carneiro EM, Davel AP. Modulation of endothelium-derived nitric oxide production and activity by taurine and taurine-conjugated bile acids. Nitric Oxide. 2020;94:48-53. doi:10.1016/j.niox.2019.10.008
27. Ashley CT, Mayank A, Nathan SB. Nitric oxide and geriatrics:implications in diagnostics and treatment of the elderly. J Geriatr Cardiol. 2012;8(4):230-242. doi:10.3724/ SP.J.1263.2011.00230
28. Kapil V, Khambata RS, Robertson A, Caulfield MJ, Ahluwalia A. Dietary nitrate provides sustained blood pressure lowering in hypertensive patients: a randomized, phase 2, double-blind, placebo-controlled study. Hypertension. 2015;65(2):320-327. doi:10.1161/ HYPERTENSIONAHA.114.04675
29. Melis MR, Argiolas A. Erectile function and sexual behavior: a review of the role of nitric oxide in the central nervous system. Biomolecules. 2021;11(12):1866. doi:10.3390/ biom11121866
30. Burnett AL. Novel nitric oxide signaling mechanisms regulate the erectile response. Int J Impot Res 2004;16(S1):S15-S19. doi:10.1038/sj.ijir.3901209
31. Rocha BS, Gago B, Barbosa RM, Laranjinha J. Dietary polyphenols generate nitric oxide from nitrite in the stomach and induce smooth muscle relaxation. Toxicology 2009;265(1-2):41-48. doi:10.1016/j.tox.2009.09.008
32. Waldbauer K, Seiringer G, Sykora C, Dirsch VM, Zehl M, Kopp B. Evaluation of apricot, bilberry, and elderberry pomace constituents and their potential to enhance the endothelial nitric oxide synthase (eNOS) activity. ACS Omega. 2018;3(9):10545-10553. doi:10.1021/ acsomega.8b00638
33. Najjar RS, Mu S, Feresin RG. Blueberry polyphenols increase nitric oxide and attenuate angiotensin II-induced oxidative stress and inflammatory signaling in human aortic endothelial cells. Antioxidants. 2022;11(4):616. doi:10.3390/ antiox11040616
34. Subedi L, Gaire BP, Kim SY, Parveen A. Nitric oxide as a target for phytochemicals in anti-neuroinflammatory prevention therapy. Int J Mol Sci. 2021;22(9):4771. doi:10.3390/ijms22094771
35. Naseem KM. The role of nitric oxide in cardiovascular diseases. Mol Aspects Med. 2005;26(1-2):33-65. doi:10.1016/j.mam.2004.09.003
36. Reitsma S, Slaaf DW, Vink H, Van Zandvoort MAMJ, Oude Egbrink MGA. The endothelial glycocalyx: composition, functions, and visualization. Pflüg Arch. 2007;454(3):345359. doi:10.1007/s00424-007-0212-8
37. Machin DR, Phuong TT, Donato AJ. The role of the endothelial glycocalyx in advanced age and cardiovascular disease. Curr Opin Pharmacol. 2019;45:66-71. doi:10.1016/j. coph.2019.04.011
38. Coronary Artery Disease (CAD). DynaMed. Accessed December 2, 2023. https://www.dynamed.com/condition/ coronary-artery-disease-cad#TOPIC_JV2_BF5_ZYB
39. CDC. Facts about hypertension. Centers for Disease Control and Prevention. Published July 6, 2023. Accessed November 30, 2023. https://www.cdc.gov/bloodpressure/ facts.htm
40. Hypertension. DynaMed. Accessed December 7, 2023. https://www.dynamed.com/condition/hypertension
41. Poznyak AV, Sadykhov NK, Kartuesov AG, et al. Hypertension as a risk factor for atherosclerosis: cardiovascular risk assessment. Front Cardiovasc Med 2022;9:959285. doi:10.3389/fcvm.2022.959285
42. Petrie JR, Guzik TJ, Touyz RM. Diabetes, hypertension, and cardiovascular disease: clinical insights and vascular mechanisms. Can J Cardiol. 2018;34(5):575-584. doi:10.1016/j.cjca.2017.12.005
43. CDC. About high cholesterol. Centers for Disease Control and Prevention. Published March 20, 2023. Accessed December 8, 2023. https://www.cdc.gov/cholesterol/about. htm
44. Nelson RH. Hyperlipidemia as a risk factor for cardiovascular disease. Prim Care Clin Off Pract 2013;40(1):195-211. doi:10.1016/j.pop.2012.11.003
45. Karr S. Epidemiology and management of hyperlipidemia Am J Manag Care. 2017;23(9 Suppl):S139-S148. https://www. ajmc.com/view/epidemiology-and-management-of-hyperlipidemiaarticle
46. Yao YS, Li TD, Zeng ZH. Mechanisms underlying direct actions of hyperlipidemia on myocardium: an updated review. Lipids Health Dis. 2020;19(1):23. doi:10.1186/s12944019-1171-8
47. Doggen CJM, Smith NL, Lemaitre RN, Heckbert SR, Rosendaal FR, Psaty BM. Serum lipid levels and the risk of venous thrombosis. Arterioscler Thromb Vasc Biol. 2004;24(10):1970-1975. doi:10.1161/01. ATV.0000143134.87051.46
48. García Raso A, Ene G, Miranda C, Vidal R, Mata R, Llamas Sillero MP. Asociación entre enfermedad tromboembólica venosa y dislipidemia. [Association between venous thrombosis and dyslipidemia.] Spanish. Med Clínica 2014;143(1):1-5. doi:10.1016/j.medcli.2013.07.024
49. De Caterina R, Martinez JA, Kohlmeier M, eds. Principles of Nutrigenetics and Nutrigenomics 1st ed. Academic Press; 2019.
50. Shahjehan RD, Bhutta BS. Coronary Artery Disease. In: StatPearls. StatPearls Publishing; 2023. Accessed November 21, 2023. http://www.ncbi.nlm.nih.gov/books/ NBK564304/
51. Malakar AK, Choudhury D, Halder B, Paul P, Uddin A, Chakraborty S. A review on coronary artery disease, its risk factors, and therapeutics. J Cell Physiol. 2019;234(10):1681216823. doi:10.1002/jcp.28350
52. Shecterle LM, Terry KR, St Cyr JA. Potential clinical benefits of d-ribose in ischemic cardiovascular disease. Cureus 2018;10(3):e2291. doi:10.7759/cureus.2291
53. Kepka A, Ochocinska A, Borzym-Kluczyk M, et al. Preventive role of L-Carnitine and balanced diet in Alzheimer’s Disease. Nutrients. 2020;12(7):1987. doi:10.3390/nu12071987
54. Ferreira GC, McKenna MC. L-carnitine and acetyl-Lcarnitine roles and neuroprotection in developing brain. Neurochem Res. 2017;42(6):1661-1675. doi:10.1007/s11064017-2288-7
55. Gnoni A, Longo S, Gnoni GV, Giudetti AM. Carnitine in human muscle bioenergetics: can carnitine supplementation improve physical exercise? Molecules 2020;25(1):182. doi:10.3390/molecules25010182
56. Mehmel M, Jovanović N, Spitz U. Nicotinamide riboside — the current state of research and therapeutic uses. Nutrients. 2020;12(6):1616. doi:10.3390/nu12061616
57. Yamamoto H, Schoonjans K, Auwerx J. Sirtuin functions in health and disease. Mol Endocrinol. 2007;21(8):1745-1755. doi:10.1210/me.2007-0079
58. Braidy N, Berg J, Clement J, et al. Role of nicotinamide adenine dinucleotide and related precursors as therapeutic targets for age-related degenerative diseases: rationale, biochemistry, pharmacokinetics, and outcomes. Antioxid Redox Signal. 2019;30(2):251-294. doi:10.1089/ars.2017.7269
59. Gomes AP, Price NL, Ling AJY, et al. Declining NAD+ induces a pseudohypoxic state disrupting nuclearmitochondrial communication during aging. Cell 2013;155(7):1624-1638. doi:10.1016/j.cell.2013.11.037
60. Satoh A, Brace CS, Rensing N, et al. Sirt1 extends life span and delays aging in mice through the regulation of Nk2 homeobox 1 in the DMH and LH. Cell Metab. 2013;18(3):416430. doi:10.1016/j.cmet.2013.07.013
61. Li Y, Wu Y, Wu L, Qin L, Liu T. The effects of probiotic administration on patients with prediabetes: a meta-analysis and systematic review. J Transl Med 2022;20(1):498. doi:10.1186/s12967-022-03695-y
62. CDC. Prediabetes — your chance to prevent type 2 diabetes. Centers for Disease Control and Prevention. Published December 30, 2022. Accessed November 20, 2023. http://bit.ly/2hMpYrt
63. Wang W, Zhu LJ, Leng YQ, et al. Inflammatory response: a crucial way for gut microbes to regulate cardiovascular diseases. Nutrients. 2023;15(3):607. doi:10.3390/ nu15030607
64. Richards EM, Pepine CJ, Raizada MK, Kim S. The gut, its microbiome, and hypertension. Curr Hypertens Rep 2017;19(4):36. doi:10.1007/s11906-017-0734-1
65. Pluznick JL, Protzko RJ, Gevorgyan H, et al. Olfactory receptor responding to gut microbiota-derived signals plays a role in renin secretion and blood pressure regulation. Proc Natl Acad Sci U S A. 2013;110(11):4410-4415. doi:10.1073/pnas.1215927110
66. Tokarek J, Budny E, Saar M, et al. Does the composition of gut microbiota affect hypertension? Molecular mechanisms involved in increasing blood pressure. Int J Mol Sci 2023;24(2):1377. doi:10.3390/ijms24021377
67. Suzuki K, Terasawa M. Biological activities of rhamnan sulfate extract from the green algae Monostroma nitidum (hitoegusa). Mar Drugs. 2020;18(4):228. doi:10.3390/ md18040228
68. Patil NP, Gómez-Hernández A, Zhang F, et al. Rhamnan sulfate reduces atherosclerotic plaque formation and vascular inflammation. Biomaterials. 2022;291:121865. doi:10.1016/j.biomaterials.2022.121865
69. Lo Sasso G, Schlage WK, Boué S, Veljkovic E, Peitsch MC, Hoeng J. The Apoe(−/−) mouse model: a suitable model to study cardiovascular and respiratory diseases in the context of cigarette smoke exposure and harm reduction. J Transl Med. 2016;14:146. doi:10.1186/s12967-016-0901-1
70. Terasawa M, Hiramoto K, Uchida R, Suzuki K. Antiinflammatory activity of orally administered Monostroma nitidum rhamnan sulfate against lipopolysaccharideinduced damage to mouse organs and vascular endothelium. Mar Drugs. 2022;20(2):121. doi:10.3390/ md20020121
71. Hargreaves I, Heaton RA, Mantle D. Disorders of human coenzyme q10 metabolism: an overview. Int J Mol Sci 2020;21(18):6695. doi:10.3390/ijms21186695
72. Sale ST, Parvez H, Yeltiwar RKR, Vivekanandan G, Pundir AJ, Jain P. A comparative evaluation of topical and intrasulcular application of coenzyme Q10 (Perio QTM) gel in chronic periodontitis patients: a clinical study. J Indian Soc Periodontol. 2014;18(4):461-465. doi:10.4103/0972124X.138690
73. Qu H, Guo M, Chai H, Wang WT, Gao ZY, Shi DZ. Effects of coenzyme q10 on statin-induced myopathy: an updated meta-analysis of randomized controlled trials. J Am Heart Assoc. 2018;7(19):e009835. doi:10.1161/JAHA.118.009835
74. Samuel TY, Hasin T, Gotsman I, et al. Coenzyme Q10 in the treatment of heart failure with preserved ejection fraction: a prospective, randomized, double-blind, placebocontrolled trial. Drugs RD. 2022;22(1):25-33. doi:10.1007/ s40268-021-00372-1
75. Dunning BJ, Bourgonje AR, Bulthuis MLC, et al. Selenium and coenzyme Q10 improve the systemic redox status while reducing cardiovascular mortality in elderly population-based individuals. Free Radic Biol Med 2023;204:207-214. doi:10.1016/j.freeradbiomed.2023.04.024
76. Pervez MA, Khan DA, Ijaz A, Khan S. Effects of deltatocotrienol supplementation on liver enzymes, inflammation, oxidative stress and hepatic steatosis in patients with nonalcoholic fatty liver disease. Turk J Gastroenterol. 2018;29(2):170-176. doi:10.5152/ tjg.2018.17297
77. Phang SCW, Ahmad B, Abdul Kadir K, M Palanisamy UD. Effects of tocotrienol-rich fraction supplementation in patients with type 2 diabetes: a systematic review and meta-analysis of randomized controlled trials. Adv Nutr Bethesda Md. 2023;14(5):1159-1169. doi:10.1016/j. advnut.2023.06.006
78. Pearce BC, Parker RA, Deason ME, Qureshi AA, Wright JJ. Hypocholesterolemic activity of synthetic and natural tocotrienols. J Med Chem. 1992;35(20):3595-3606. doi:10.1021/jm00098a002
79. Serbinova E, Kagan V, Han D, Packer L. Free radical recycling and intramembrane mobility in the antioxidant properties of alpha-tocopherol and alpha-tocotrienol. Free Radic Biol Med. 1991;10(5):263-275. doi:10.1016/08915849(91)90033-y
80. Ranasinghe R, Mathai M, Zulli A. Revisiting the therapeutic potential of tocotrienol. Biofactors Oxf Engl 2022;48(4):813-856. doi:10.1002/biof.1873
81. Nasir NAA, Sadikan MZ, Agarwal R. Modulation of NFκB signalling pathway by tocotrienol: a systematic review. Asia Pac J Clin Nutr. 2021;30(3):537-555. doi:10.6133/ apjcn.202109_30(3).0020
82. Qureshi AA. Impact of δ-tocotrienol on inflammatory biomarkers and oxidative stress in hypercholesterolemic subjects. J Clin Exp Cardiol. 2015;06(04). doi:10.4172/21559880.1000367
83. Muid S, Froemming GRA, Rahman T, Ali AM, Nawawi HM. Delta- and gamma-tocotrienol isomers are potent in inhibiting inflammation and endothelial activation in stimulated human endothelial cells. Food Nutr Res 2016;60:10.3402/fnr.v60.31526. doi:10.3402/fnr.v60.31526
84. Rahman TA, Hassim NF, Zulkafli N, Muid S, Kornain NK, Nawawi H. Atheroprotective effects of pure tocotrienol supplementation in the treatment of rabbits with experimentally induced early and established atherosclerosis. Food Nutr Res. 2016;60:10.3402/fnr. v60.31525. doi:10.3402/fnr.v60.31525
85. Newaz MA, Nawal NN. Effect of gamma-tocotrienol on blood pressure, lipid peroxidation and total antioxidant status in spontaneously hypertensive rats (SHR). Clin Exp Hypertens. 1999;21(8):1297-1313. doi:10.3109/10641969909070850
86. Newaz MA, Yousefipour Z, Nawal N, Adeeb N. Nitric oxide synthase activity in blood vessels of spontaneously hypertensive rats: antioxidant protection by gammatocotrienol. J Physiol Pharmacol. 2003;54(3):319-327.
87. Mahjabeen W, Khan DA, Mirza SA, Pervez MA. Effects of delta-tocotrienol supplementation on glycemic control, oxidative stress, inflammatory biomarkers and miRNA expression in type 2 diabetes mellitus: a randomized control trial. Phytother Res 2021;35(7):3968-3976. doi:10.1002/ptr.7113
88. Suleman F, Khan DA, Pervez MA, Aamir M. Effects of delta-tocotrienol supplementation on glycaemic control in individuals with prediabetes: a randomized controlled study. J Pak Med Assoc. 2022;72(1):4-7. doi:10.47391/ JPMA.966
89. Qureshi AA. Tocotrienols: exciting biological and pharmacological properties of tocotrienols and other naturally occurring compounds, Part I. Ann Clin Case Rep 2022;7(1):2194. PMCID: PMC9794443
90. Fatima S, Khan DA, Aamir M, Pervez MA, Fatima F. δ-Tocotrienol in combination with resveratrol improves the cardiometabolic risk factors and biomarkers in patients with metabolic syndrome: a randomized controlled trial. Metab Syndr Relat Disord. 2023;21(1):25-34. doi:10.1089/ met.2022.0052
91. Qureshi AA, Khan DA, Mahjabeen W, Papasian CJ, Qureshi N. Suppression of nitric oxide production and cardiovascular risk factors in healthy seniors and hypercholesterolemic subjects by a combination of polyphenols and vitamins. J Clin Exp Cardiol. 2012;Suppl 5:008. doi:10.4172/2155-9880.S5-008
92. Martínez-Huélamo M, Rodríguez-Morató J, Boronat A, de la Torre R. Modulation of Nrf2 by olive oil and wine polyphenols and neuroprotection. Antioxidants (Basel) 2017;6(4):73. doi:10.3390/antiox6040073
93. Parsamanesh N, Asghari A, Sardari S, et al. Resveratrol and endothelial function: a literature review. Pharmacol Res 2021;170:105725. doi:10.1016/j.phrs.2021.105725
94. Karimi A, Azar PS, Kadkhodayi M, et al. A comprehensive insight into effects of resveratrol on molecular mechanism in rheumatoid arthritis: a literature systematic review. Int J Rheum Dis. 2022;25(8):827-843. doi:10.1111/1756-185X.14356
95. Jaimes JD, Jarosova V, Vesely O, et al. Effect of selected stilbenoids on human fecal microbiota. Mol Basel Switz 2019;24(4):744. doi:10.3390/molecules24040744
96. Hu Y, Chen D, Zheng P, et al. The bidirectional interactions between resveratrol and gut microbiota: an insight into oxidative stress and inflammatory bowel disease therapy. Biomed Res Int. 2019;2019:e5403761. doi:10.1155/2019/5403761
97. de Oliveira MR, Nabavi SF, Manayi A, Daglia M, Hajheydari Z, Nabavi SM. Resveratrol and the mitochondria: from triggering the intrinsic apoptotic pathway to inducing mitochondrial biogenesis, a mechanistic view. Biochim Biophys Acta. 2016;1860(4):727-745. doi:10.1016/j. bbagen.2016.01.017
98. Xia N, Daiber A, Förstermann U, Li H. Antioxidant effects of resveratrol in the cardiovascular system. Br J Pharmacol 2017;174(12):1633-1646. doi:10.1111/bph.13492
99. McCormack D, McFadden D. A review of pterostilbene antioxidant activity and disease modification. Oxid Med Cell Longev. 2013;2013:575482. doi:10.1155/2013/575482
100. Cai Y, Xin Q, Lu J, et al. A new therapeutic candidate for cardiovascular diseases: berberine. Front Pharmacol 2021;12:631100. doi:10.3389/fphar.2021.631100
101. Imenshahidi M, Hosseinzadeh H. Berberine and barberry (Berberis vulgaris): a clinical review. Phytother Res 2019;33(3):504-523. doi:10.1002/ptr.6252
102. Chen H, Chen J, Zhang F, et al. Effective management of atherosclerosis progress and hyperlipidemia with nattokinase: a clinical study with 1,062 participants. Front Cardiovasc Med. 2022;9:964977. doi:10.3389/ fcvm.2022.964977
103. Yang L, Zhu W, Zhang X, Zhou X, Wu W, Shen T. Efficacy and safety of berberine for several cardiovascular diseases: a systematic review and meta-analysis of randomized controlled trials. Phytomedicine. 2023;112:154716. doi:10.1016/j.phymed.2023.154716
104. Zamani M, Zarei M, Nikbaf-Shandiz M, Hosseini S, Shiraseb F, Asbaghi O. The effects of berberine supplementation on cardiovascular risk factors in adults: a systematic review and dose-response meta-analysis. Front Nutr 2022;9:1013055. doi:10.3389/fnut.2022.1013055
105. Oppedisano F, Bulotta RM, Maiuolo J, et al. The role of nutraceuticals in osteoarthritis prevention and treatment: focus on n-3 PUFAs. Oxid Med Cell Longev 2021;2021:4878562. doi:10.1155/2021/4878562
106. Liao J, Xiong Q, Yin Y, Ling Z, Chen S. The effects of fish oil on cardiovascular diseases: systematical evaluation and recent advance. Front Cardiovasc Med. 2022;8:802306. doi:10.3389/fcvm.2021.802306
107. Ishihara T, Yoshida M, Arita M. Omega-3 fatty acidderived mediators that control inflammation and tissue homeostasis. Int Immunol. 2019;31(9):559-567. doi:10.1093/ intimm/dxz001
108. Arita M, Bianchini F, Aliberti J, et al. Stereochemical assignment, antiinflammatory properties, and receptor for the omega-3 lipid mediator resolvin E1. J Exp Med 2005;201(5):713-722. doi:10.1084/jem.20042031
109. Weiser M, Butt C, Mohajeri M. Docosahexaenoic acid and cognition throughout the lifespan. Nutrients. 2016;8(2):99. doi:10.3390/nu8020099
110. Troesch B, Eggersdorfer M, Laviano A, et al. Expert opinion on benefits of long-chain omega-3 fatty acids (DHA and EPA) in aging and clinical nutrition. Nutrients 2020;12(9):2555. doi:10.3390/nu12092555
111. Schwalfenberg GK, Genuis SJ. The importance of magnesium in clinical healthcare. Scientifica 2017;2017:4179326. doi:10.1155/2017/4179326
112. Fritzen R, Davies A, Veenhuizen M, et al. Magnesium deficiency and cardiometabolic disease. Nutrients 2023;15(10):2355. doi:10.3390/nu15102355
113. Kostov K, Halacheva L. Role of magnesium deficiency in promoting atherosclerosis, endothelial dysfunction, and arterial stiffening as risk factors for hypertension. Int J Mol Sci. 2018;19(6):1724. doi:10.3390/ijms19061724
114. Qu X, Jin F, Hao Y, et al. Magnesium and the risk of cardiovascular events: a meta-analysis of prospective cohort studies. PloS One. 2013;8(3):e57720. doi:10.1371/ journal.pone.0057720
115. Severino P, Netti L, Mariani MV, et al. Prevention of cardiovascular disease: screening for magnesium deficiency. Cardiol Res Pract. 2019;2019:4874921. doi:10.1155/2019/4874921
116. Mazur A, Maier JAM, Rock E, Gueux E, Nowacki W, Rayssiguier Y. Magnesium and the inflammatory response: potential physiopathological implications. Arch Biochem Biophys. 2007;458(1):48-56. doi:10.1016/j.abb.2006.03.031
117. Zhang X, Li Y, Del Gobbo LC, et al. Effects of magnesium supplementation on blood pressure: a meta-analysis of randomized double-blind placebo-controlled trials. Hypertension. 2016;68(2):324-333. doi:10.1161/ HYPERTENSIONAHA.116.07664
118. Zhao L, Hu M, Yang L, et al. Quantitative association between serum/dietary magnesium and cardiovascular disease/coronary heart disease risk: a dose–response meta-analysis of prospective cohort studies. J Cardiovasc Pharmacol. 2019;74(6):516-527. doi:10.1097/ FJC.0000000000000739
119. Del Gobbo LC, Imamura F, Wu JH, De Oliveira Otto MC, Chiuve SE, Mozaffarian D. Circulating and dietary magnesium and risk of cardiovascular disease: a systematic review and meta-analysis of prospective studies. Am J Clin Nutr. 2013;98(1):160-173. doi:10.3945/ajcn.112.053132
120. Hwang DL, Yen CF, Nadler JL. Effect of extracellular magnesium on platelet activation and intracellular calcium mobilization. Am J Hypertens. 1992;5(10):700-706. doi:10.1093/ajh/5.10.700
121. Rukshin V, Shah PK, Cercek B, Finkelstein A, Tsang V, Kaul S. Comparative antithrombotic effects of magnesium sulfate and the platelet glycoprotein IIb/IIIa inhibitors tirofiban and eptifibatide in a canine model of stent thrombosis. Circulation. 2002;105(16):1970-1975. doi:10.1161/01.CIR.0000014612.88433.62
122. Office of Dietary Supplements. Magnesium: fact sheet for health professionals. National Institutes of Health. Updated June 2, 2022. Accessed November 29, 2023. https://ods. od.nih.gov/factsheets/Magnesium-HealthProfessional/
123. Zeana C. Magnesium orotate in myocardial and neuronal protection. Rom J Intern Med. 1999;37(1):91-97.
124. Classen HG. Magnesium orotate--experimental and clinical evidence. Rom J Intern Med. 2004;42(3):491-501.
125. Rosenfeldt FL. Metabolic supplementation with orotic acid and magnesium orotate. Cardiovasc Drugs Ther. 1998;12 Suppl 2:147-152. doi:10.1023/a:1007732131887
126. Stepura OB, Martynow AI. Magnesium orotate in severe congestive heart failure (MACH). Int J Cardiol 2009;134(1):145-147. doi:10.1016/j.ijcard.2009.01.047
127. Geiss KR, Stergiou N, Jester null, Neuenfeld HU, Jester HG. Effects of magnesium orotate on exercise tolerance in patients with coronary heart disease. Cardiovasc Drugs Ther. 1998;12 Suppl 2:153-156. doi:10.1023/a:1007796515957
128. Petroni ML, Brodosi L, Marchignoli F, et al. Nutrition in patients with type 2 diabetes: present knowledge and remaining challenges. Nutrients. 2021;13(8):2748. doi:10.3390/nu13082748
129. Sassi F, Tamone C, D’Amelio P. Vitamin D: nutrient, hormone, and immunomodulator. Nutrients 2018;10(11):1656. doi:10.3390/nu10111656
130. Tuoresmäki P, Väisänen S, Neme A, Heikkinen S, Carlberg C. Patterns of genome-wide VDR locations. PloS One 2014;9(4):e96105. doi:10.1371/journal.pone.0096105
131. Zhang Y, Wu S, Sun J. Vitamin D, vitamin D receptor and tissue barriers. Tissue Barriers. 2013;1(1):e23118. doi:10.4161/ tisb.23118
132. Trehan N, Afonso L, Levine DL, Levy PD. Vitamin D deficiency, supplementation, and cardiovascular health. Crit Pathw Cardiol. 2017;16(3):109-118. doi:10.1097/ HPC.0000000000000122
133. Hoseini R, Rahim HA, Ahmed JK. Concurrent alteration in inflammatory biomarker gene expression and oxidative stress: how aerobic training and vitamin D improve T2DM. BMC Complement Med Ther. 2022;22(1):165. doi:10.1186/ s12906-022-03645-7
134. Zhang Y, Fang F, Tang J, et al. Association between vitamin D supplementation and mortality: systematic review and meta-analysis. BMJ. 2019;366:l4673. doi:10.1136/bmj.l4673
135. Yamamoto E, Jørgensen TN. Immunological effects of vitamin D and their relations to autoimmunity. J Autoimmun. 2019;100:7-16. doi:10.1016/j.jaut.2019.03.002
136. Mehta V, Agarwal S. Does vitamin D deficiency lead to hypertension? Cureus. 2017;9(2):e1038. doi:10.7759/ cureus.1038
137. Ullah MI, Uwaifo GI, Nicholas WC, Koch CA. Does vitamin D deficiency cause hypertension? Current evidence from clinical studies and potential mechanisms. Int J Endocrinol 2010;2010:1-11. doi:10.1155/2010/579640
138. Logan VF, Gray AR, Peddie MC, Harper MJ, Houghton LA. Long-term vitamin D3 supplementation is more effective than vitamin D2 in maintaining serum 25-hydroxyvitamin D status over the winter months. Br J Nutr. 2013;109(6):10821088. doi:10.1017/S0007114512002851
139. Martineau AR, Thummel KE, Wang Z, et al. Differential effects of oral boluses of vitamin D2 vs vitamin D3 on vitamin D metabolism: a randomized controlled trial. J Clin Endocrinol Metab. 2019;104(12):5831-5839. doi:10.1210/ jc.2019-00207
140. Office of Dietary Supplements. Vitamin K: fact sheet for health professionals. National Institutes of Health. Updated March 29, 2021. Accessed December 1, 2023. https://ods. od.nih.gov/factsheets/VitaminK-HealthProfessional/
141. van Ballegooijen AJ, Beulens JWJ, Kieneker LM, et al. Combined low vitamin D and K status amplifies mortality risk: a prospective study. Eur J Nutr. 2021;60(3):1645-1654. doi:10.1007/s00394-020-02352-8
142. Yang T, Santisteban MM, Rodriguez V, et al. Gut dysbiosis is linked to hypertension. Hypertension. 2015;65(6):1331-1340. doi:10.1161/HYPERTENSIONAHA.115.05315
143. Nakamura Y, Yamamoto N, Sakai K, Takano T. Antihypertensive effect of sour milk and peptides isolated from it that are inhibitors to angiotensin I-converting enzyme. J Dairy Sci. 1995;78(6):1253-1257. doi:10.3168/jds. S0022-0302(95)76745-5
144. Wilck N, Matus MG, Kearney SM, et al. Salt-responsive gut commensal modulates TH17 axis and disease. Nature 2017;551(7682):585-589. doi:10.1038/nature24628
145. Qi D, Nie XL, Zhang JJ. The effect of probiotics supplementation on blood pressure: a systemic review and meta-analysis. Lipids Health Dis. 2020;19(1):79. doi:10.1186/ s12944-020-01259-x
146. Liang T, Wu L, Xi Y, et al. Probiotics supplementation improves hyperglycemia, hypercholesterolemia, and hypertension in type 2 diabetes mellitus: an update of meta-analysis. Crit Rev Food Sci Nutr. 2021;61(10):16701688. doi:10.1080/10408398.2020.1764488
147. Mollace V, Sacco I, Janda E, et al. Hypolipemic and hypoglycaemic activity of bergamot polyphenols: from animal models to human studies. Fitoterapia 2011;82(3):309-316. doi:10.1016/j.fitote.2010.10.014
148. Lamiquiz-Moneo I, Giné-González J, Alisente S, et al. Effect of bergamot on lipid profile in humans: a systematic review. Crit Rev Food Sci Nutr. 2020;60(18):3133-3143. doi:10.1080/ 10408398.2019.1677554
149. Giglio RV, Patti AM, Nikolic D, et al. The effect of bergamot on dyslipidemia. Phytomedicine. 2016;23(11):1175-1181. doi:10.1016/j.phymed.2015.12.005
150. Cheung B, Sikand G, Dineen EH, Malik S, Barseghian ElFarra A. Lipid-lowering nutraceuticals for an integrative approach to dyslipidemia. J Clin Med. 2023;12(10):3414. doi:10.3390/jcm12103414
151. Mannucci C, Navarra M, Calapai F, Squeri R, Gangemi S, Calapai G. Clinical pharmacology of Citrus bergamia: a systematic review. Phytother Res. 2017;31(1):27-39. doi:10.1002/ptr.5734
152. Toth PP, Patti AM, Nikolic D, et al. Bergamot reduces plasma lipids, atherogenic small dense LDL, and subclinical atherosclerosis in subjects with moderate hypercholesterolemia: a 6 months prospective study. Front Pharmacol. 2015;6:299. doi:10.3389/fphar.2015.00299
153. Scuteri D, Rombolà L, Morrone LA, et al. Neuropharmacology of the neuropsychiatric symptoms of dementia and role of pain: essential oil of bergamot as a novel therapeutic approach. Int J Mol Sci. 2019;20(13):3327. doi:10.3390/ijms20133327
154. Seifert JG, Brumet A, St Cyr JA. The influence of D-ribose ingestion and fitness level on performance and recovery. J Int Soc Sports Nutr. 2017;14:47. doi:10.1186/s12970-0170205-8
155. Salehi B, Berkay Yılmaz Y, Antika G, et al. Insights on the use of α-lipoic acid for therapeutic purposes. Biomolecules 2019;9(8):356. doi:10.3390/biom9080356
156. Tibullo D, Li Volti G, Giallongo C, et al. Biochemical and clinical relevance of alpha lipoic acid: antioxidant and antiinflammatory activity, molecular pathways and therapeutic potential. Inflamm Res. 2017;66(11):947-959. doi:10.1007/ s00011-017-1079-6
157. Amponsah-Offeh M, Diaba-Nuhoho P, Speier S, Morawietz H. Oxidative stress, antioxidants and hypertension. Antioxidants (Basel). 2023;12(2):281. doi:10.3390/ antiox12020281
158. de Sousa CNS, da Silva Leite CMG, da Silva Medeiros I, et al. Alpha-lipoic acid in the treatment of psychiatric and neurological disorders: a systematic review. Metab Brain Dis. 2019;34(1):39-52. doi:10.1007/s11011-018-0344-x
159. Dludla PV, Ziqubu K, Mabhida SE, et al. Dietary supplements potentially target plasma glutathione levels to improve cardiometabolic health in patients with diabetes mellitus: a systematic review of randomized clinical trials. Nutrients. 2023;15(4):944. doi:10.3390/nu15040944
160. Imae M, Asano T, Murakami S. Potential role of taurine in the prevention of diabetes and metabolic syndrome. Amino Acids. 2014;46(1):81-88. doi:10.1007/s00726-012-1434-4
161. Wójcik OP, Koenig KL, Zeleniuch-Jacquotte A, Costa M, Chen Y. The potential protective effects of taurine on coronary heart disease. Atherosclerosis. 2010;208(1):19. doi:10.1016/j.atherosclerosis.2009.06.002
162. McCarty MF. Sub-optimal taurine status may promote platelet hyperaggregability in vegetarians. Med Hypotheses. 2004;63(3):426-433. doi:10.1016/j. mehy.2002.11.007
163. Tao X, Zhang Z, Yang Z, Rao B. The effects of taurine supplementation on diabetes mellitus in humans: a systematic review and meta-analysis. Food Chem Mol Sci 2022;4:100106. doi:10.1016/j.fochms.2022.100106
164. Chen W, Guo J, Zhang Y, Zhang J. The beneficial effects of taurine in preventing metabolic syndrome. Food Funct 2016;7(4):1849-1863. doi:10.1039/c5fo01295c
165. Jong CJ, Sandal P, Schaffer SW. The role of taurine in mitochondria health: more than just an antioxidant. Molecules. 2021;26(16):4913. doi:10.3390/ molecules26164913
166. Yamori Y, Taguchi T, Mori H, Mori M. Low cardiovascular risks in the middle aged males and females excreting greater 24-hour urinary taurine and magnesium in 41 WHOCARDIAC study populations in the world. J Biomed Sci 2010;17(Suppl 1):S21. doi:10.1186/1423-0127-17-S1-S21
167. Merheb M, Daher RT, Nasrallah M, Sabra R, Ziyadeh FN, Barada K. Taurine intestinal absorption and renal excretion test in diabetic patients: a pilot study. Diabetes Care 2007;30(10):2652-2654. doi:10.2337/dc07-0872
168. Qaradakhi T, Gadanec LK, McSweeney KR, Abraham JR, Apostolopoulos V, Zulli A. The anti-inflammatory effect of taurine on cardiovascular disease. Nutrients 2020;12(9):2847. doi:10.3390/nu12092847
169. Airhart SE, Shireman LM, Risler LJ, et al. An open-label, non-randomized study of the pharmacokinetics of the nutritional supplement nicotinamide riboside (NR) and its effects on blood NAD+ levels in healthy volunteers. PloS One. 2017;12(12):e0186459. doi:10.1371/journal. pone.0186459
170. Martens CR, Denman BA, Mazzo MR, et al. Chronic nicotinamide riboside supplementation is welltolerated and elevates NAD+ in healthy middle-aged and older adults. Nat Commun. 2018;9(1):1286. doi:10.1038/ s41467-018-03421-7
171. Zhou B, Wang DDH, Qiu Y, et al. Boosting NAD level suppresses inflammatory activation of PBMCs in heart failure. J Clin Invest. 2020;130(11):6054-6063. doi:10.1172/ JCI138538
172. Cerutti R, Pirinen E, Lamperti C, et al. NAD(+)dependent activation of Sirt1 corrects the phenotype in a mouse model of mitochondrial disease. Cell Metab 2014;19(6):1042-1049. doi:10.1016/j.cmet.2014.04.001
173. Yang H, Brosel S, Acin-Perez R, et al. Analysis of mouse models of cytochrome c oxidase deficiency owing to mutations in Sco2. Hum Mol Genet. 2010;19(1):170-180. doi:10.1093/hmg/ddp477
174. Hoepner CT, McIntyre RS, Papakostas GI. Impact of supplementation and nutritional interventions on pathogenic processes of mood disorders: a review of the evidence. Nutrients. 2021;13(3):767. doi:10.3390/ nu13030767
175. Zhao T, Chen S, Wang B, Cai D. L-carnitine reduces myocardial oxidative stress and alleviates myocardial ischemia-reperfusion injury by activating nuclear transcription-related factor 2 (Nrf2)/Heme Oxygenase-1 (HO-1) signaling pathway. Med Sci Monit. 2020;26:e923251. doi:10.12659/MSM.923251
176. Li M, Xu S, Geng Y, et al. The protective effects of L-carnitine on myocardial ischaemia-reperfusion injury in patients with rheumatic valvular heart disease undergoing CPB surgery are associated with the suppression of NF-κB pathway and the activation of Nrf2 pathway. Clin Exp Pharmacol Physiol. 2019;46(11):1001-1012. doi:10.1111/14401681.13155
177. Asadi M, Rahimlou M, Shishehbor F, Mansoori A. The effect of L-carnitine supplementation on lipid profile and glycaemic control in adults with cardiovascular risk factors: a systematic review and meta-analysis of randomized controlled clinical trials. Clin Nutr. 2020;39(1):110-122. doi:10.1016/j.clnu.2019.01.020
178. Serban MC, Sahebkar A, Mikhailidis DP, et al. Impact of L-carnitine on plasma lipoprotein(a) concentrations: a systematic review and meta-analysis of randomized controlled trials. Sci Rep. 2016;6:19188. doi:10.1038/ srep19188
179. Li D, Hou L, Hu M, et al. Recent advances in nattokinaseenriched fermented soybean foods: a review. Foods 2022;11(13):1867. doi:10.3390/foods11131867
180. Chen H, McGowan EM, Ren N, et al. Nattokinase: a promising alternative in prevention and treatment of cardiovascular diseases. Biomark Insights 2018;13:1177271918785130. doi:10.1177/1177271918785130
181. Pannu A, Sharma PC, Thakur VK, Goyal RK. Emerging role of flavonoids as the treatment of depression. Biomolecules 2021;11(12):1825. doi:10.3390/biom11121825
182. Johnson SA, Figueroa A, Navaei N, et al. Daily blueberry consumption improves blood pressure and arterial stiffness in postmenopausal women with pre- and stage 1-hypertension: a randomized, double-blind, placebocontrolled clinical trial. J Acad Nutr Diet. 2015;115(3):369377. doi:10.1016/j.jand.2014.11.001
183. Kent K, Charlton KE, Jenner A, Roodenrys S. Acute reduction in blood pressure following consumption of anthocyanin-rich cherry juice may be dose-interval dependant: a pilot cross-over study. Int J Food Sci Nutr 2016;67(1):47-52. doi:10.3109/09637486.2015.1121472
184. Alappat B, Alappat J. Anthocyanin pigments: beyond aesthetics. Molecules. 2020;25(23):5500. doi:10.3390/ molecules25235500
185. Rodriguez-Mateos A, Istas G, Boschek L, et al. Circulating anthocyanin metabolites mediate vascular benefits of blueberries: insights from randomized controlled trials, metabolomics, and nutrigenomics. J Gerontol A Biol Sci Med Sci. 2019;74(7):967-976. doi:10.1093/gerona/glz047
186. Chen J, Shu Y, Chen Y, et al. Evaluation of antioxidant capacity and gut microbiota modulatory effects of different kinds of berries. Antioxidants. 2022;11(5):1020. doi:10.3390/antiox11051020
187. Davani-Davari D, Negahdaripour M, Karimzadeh I, et al. Prebiotics: definition, types, sources, mechanisms, and clinical applications. Foods. 2019;8(3):92. doi:10.3390/ foods8030092
188. Sesso HD, Manson JE, Aragaki AK, et al. Effect of cocoa flavanol supplementation for the prevention of cardiovascular disease events: the COcoa Supplement and Multivitamin Outcomes Study (COSMOS) randomized clinical trial. Am J Clin Nutr. 2022;115(6):1490-1500. doi:10.1093/ajcn/nqac055
189. Siervo M, Lara J, Ogbonmwan I, Mathers JC. Inorganic nitrate and beetroot juice supplementation reduces blood pressure in adults: a systematic review and meta-analysis. J Nutr. 2013;143(6):818-826. doi:10.3945/jn.112.170233
190. Cheung MM, Dall RD, Shewokis PA, et al. The effect of combined magnesium and vitamin D supplementation on vitamin D status, systemic inflammation, and blood pressure: a randomized double-blinded controlled trial. Nutrition. 2022;99-100:111674. doi:10.1016/j.nut.2022.111674
191. Alcázar-Leyva S, Alvarado-Vásquez N. Could thiamine pyrophosphate be a regulator of the nitric oxide synthesis in the endothelial cell of diabetic patients? Med Hypotheses. 2011;76(5):629-631. doi:10.1016/j. mehy.2011.01.015
192. Gioda CR, Capettini LSA, Cruz JS, Lemos VS. Thiamine deficiency leads to reduced nitric oxide production and vascular dysfunction in rats. Nutr Metab Cardiovasc Dis 2014;24(2):183-188. doi:10.1016/j.numecd.2013.06.010
193. Mousavi SM, Mofrad MD, Do Nascimento IJB, Milajerdi A, Mokhtari T, Esmaillzadeh A. The effect of zinc supplementation on blood pressure: a systematic review and dose–response meta-analysis of randomizedcontrolled trials. Eur J Nutr. 2020;59(5):1815-1827. doi:10.1007/s00394-020-02204-5
194. May JM, Harrison FE. Role of Vitamin C in the function of the vascular endothelium. Antioxid Redox Signal 2013;19(17):2068-2083. doi:10.1089/ars.2013.5205
195. Basaqr R, Skleres M, Jayswal R, Thomas DT. The effect of dietary nitrate and vitamin C on endothelial function, oxidative stress and blood lipids in untreated hypercholesterolemic subjects: a randomized doubleblind crossover study. Clin Nutr. 2021;40(4):1851-1860. doi:10.1016/j.clnu.2020.10.012
196. Ashor AW, Shannon OM, Werner AD, et al. Effects of inorganic nitrate and vitamin C co-supplementation on blood pressure and vascular function in younger and older healthy adults: a randomised double-blind crossover trial. Clin Nutr. 2020;39(3):708-717. doi:10.1016/j. clnu.2019.03.006
197. Boonthongkaew C, Tong-Un T, Kanpetta Y, Chaungchot N, Leelayuwat C, Leelayuwat N. Vitamin C supplementation improves blood pressure and oxidative stress after acute exercise in patients with poorly controlled type 2 diabetes mellitus: a randomized, placebo-controlled, cross-over study. Chin J Physiol. 2021;64(1):16. doi:10.4103/cjp. cjp_95_20
198. Cherukuri L, Birudaraju D, Kinninger A, et al. Effect of a plant-based bioequivalent inorganic nitrate (NO 3-) complex with vitamins, antioxidants and phytophenol rich food extracts in hypertensive individuals — a randomized, double-blind, placebo-controlled study. Clin Nutr ESPEN 2020;40:327-335. doi:10.1016/j.clnesp.2020.08.007
199. Binia A, Jaeger J, Hu Y, Singh A, Zimmermann D. Daily potassium intake and sodium-to-potassium ratio in the reduction of blood pressure: a meta-analysis of randomized controlled trials. J Hypertens. 2015;33(8):1509-1520. doi:10.1097/HJH.0000000000000611
200. Poorolajal J, Zeraati F, Soltanian AR, Sheikh V, Hooshmand E, Maleki A. Oral potassium supplementation for management of essential hypertension: a metaanalysis of randomized controlled trials. PLoS One 2017;12(4):e0174967. doi:10.1371/journal.pone.0174967
201. Filippini T, Violi F, D’Amico R, Vinceti M. The effect of potassium supplementation on blood pressure in hypertensive subjects: a systematic review and metaanalysis. Int J Cardiol. 2017;230:127-135. doi:10.1016/j. ijcard.2016.12.048
202. Joshipura K, Muñoz-Torres F, Fernández-Santiago J, Patel RP, Lopez-Candales A. Over-the-counter mouthwash use, nitric oxide and hypertension risk. Blood Press 2020;29(2):103-112. doi:10.1080/08037051.2019.1680270
203. Kapil V, Haydar SMA, Pearl V, Lundberg JO, Weitzberg E, Ahluwalia A. Physiological role for nitrate-reducing oral bacteria in blood pressure control. Free Radic Biol Med 2013;55:93-100. doi:10.1016/j.freeradbiomed.2012.11.013
204. Bondonno CP, Liu AH, Croft KD, et al. Antibacterial mouthwash blunts oral nitrate reduction and increases blood pressure in treated hypertensive men and women. Am J Hypertens. 2015;28(5):572-575. doi:10.1093/ajh/ hpu192
205. Zhurakivska K, Troiano G, Caponio VCA, et al. Do changes in oral microbiota correlate with plasma nitrite response? A systematic review. Front Physiol. 2019;10:1029. doi:10.3389/ fphys.2019.01029
206. Kieffer DA, Martin RJ, Adams SH. Impact of dietary fibers on nutrient management and detoxification organs: gut, liver, and kidneys. Adv Nutr. 2016;7(6):1111-1121. doi:10.3945/ an.116.013219
207. García-Montero C, Fraile-Martínez O, Gómez-Lahoz AM, et al. Nutritional components in western diet versus Mediterranean diet at the gut microbiota-immune system interplay. Implications for health and disease. Nutrients 2021;13(2):699. doi:10.3390/nu13020699
208. Guasch-Ferré M, Willett WC. The Mediterranean diet and health: a comprehensive overview. J Intern Med 2021;290(3):549-566. doi:10.1111/joim.13333
209. Shively CA, Appt SE, Chen H, et al. Mediterranean diet, stress resilience, and aging in nonhuman primates. Neurobiol Stress. 2020;13:100254. doi:10.1016/j. ynstr.2020.100254
210. Jimenez-Torres J, Alcalá-Diaz JF, Torres-Peña JD, et al. Mediterranean diet reduces atherosclerosis progression in coronary heart disease: an analysis of the CORDIOPREV randomized controlled trial. Stroke. 2021;52(11):3440-3449. doi:10.1161/STROKEAHA.120.033214
211. Pes GM, Dore MP, Tsofliou F, Poulain M. Diet and longevity in the Blue Zones: a set-and-forget issue? Maturitas 2022;164:31-37. doi:10.1016/j.maturitas.2022.06.004
212. Appel LJ, Moore TJ, Obarzanek E, et al. A clinical trial of the effects of dietary patterns on blood pressure. DASH Collaborative Research Group. N Engl J Med 1997;336(16):1117-1124. doi:10.1056/NEJM199704173361601
213. Castilla-Ojo N, Turkson-Ocran RA, Conlin PR, Appel LJ, Miller ER, Juraschek SP. Effects of the DASH diet and losartan on serum urate among adults with hypertension: results of a randomized trial. J Clin Hypertens 2023;25(10):915-922. doi:10.1111/jch.14721
214. Kim H, Lichtenstein AH, White K, et al. Plasma metabolites associated with a protein-rich dietary pattern: results from the OmniHeart Trial. Mol Nutr Food Res 2022;66(6):2100890. doi:10.1002/mnfr.202100890
215. Appel LJ, Sacks FM, Carey VJ, et al. Effects of protein, monounsaturated fat, and carbohydrate intake on blood pressure and serum lipids: results of the OmniHeart randomized trial. JAMA. 2005;294(19):2455-2464. doi:10.1001/jama.294.19.2455
216. Swain JF, McCarron PB, Hamilton EF, Sacks FM, Appel LJ. Characteristics of the diet patterns tested in the Optimal Macronutrient Intake Trial to Prevent Heart Disease (OmniHeart): options for a heart-healthy diet. J Am Diet Assoc. 2008;108(2):257-265. doi:10.1016/j.jada.2007.10.040
217. Sacks FM, Svetkey LP, Vollmer WM, et al. Effects on blood pressure of reduced dietary sodium and the Dietary Approaches to Stop Hypertension (DASH) diet. DASHSodium Collaborative Research Group. N Engl J Med 2001;344(1):3-10. doi:10.1056/NEJM200101043440101
218. Chen HY, Li SC, Chen LF, Wang W, Wang Y, Yan XW. The effects of cigarette smoking and smoking cessation on high-density lipoprotein functions: implications for coronary artery disease. Ann Clin Biochem Int J Lab Med 2019;56(1):100-111. doi:10.1177/0004563218788386
219. Echeagaray O, Savko C, Gallo A, Sussman M. Cardiovascular consequences of vaping. Curr Opin Cardiol. 2022;37(3):227-235. doi:10.1097/ HCO.0000000000000952
220. Franklin BA, Eijsvogels TMH, Pandey A, Quindry J, Toth PP. Physical activity, cardiorespiratory fitness, and cardiovascular health: a clinical practice statement of the American Society for Preventive Cardiology Part II: Physical activity, cardiorespiratory fitness, minimum and goal intensities for exercise training, prescriptive methods, and special patient populations. Am J Prev Cardiol 2022;12:100425. doi:10.1016/j.ajpc.2022.100425
221. Costa RR, Buttelli ACK, Vieira AF, et al. Effect of strength training on lipid and inflammatory outcomes: systematic review with meta-analysis and meta-regression. J Phys Act Health. 2019;16(6):477-491. doi:10.1123/jpah.2018-0317
222. Bottani E, Lamperti C, Prigione A, Tiranti V, Persico N, Brunetti D. Therapeutic approaches to treat mitochondrial diseases: “one-size-fits-all” and “precision medicine” strategies. Pharmaceutics. 2020;12(11):1083. doi:10.3390/ pharmaceutics12111083
223. Fioranelli M, Bottaccioli AG, Bottaccioli F, Bianchi M, Rovesti M, Roccia MG. Stress and inflammation in coronary artery disease: a review psychoneuroendocrineimmunology-based. Front Immunol 2018;9:2031. doi:10.3389/fimmu.2018.02031
224. Yu Y, Xu Y, Zhang M, Wang Y, Zou W, Gu Y. Value of assessing autonomic nervous function by heart rate variability and heart rate turbulence in hypertensive patients. Int J Hypertens. 2018;2018:1-9. doi:10.1155/2018/4067601
225. Qian H, Li G, Luo Y, et al. Relationship between occupational metal exposure and hypertension risk based on conditional logistic regression analysis. Metabolites 2022;12(12):1259. doi:10.3390/metabo12121259
226. Gambelunghe A, Sallsten G, Borné Y, et al. Low-level exposure to lead, blood pressure, and hypertension in a population-based cohort. Environ Res. 2016;149:157-163. doi:10.1016/j.envres.2016.05.015
227. Rochester JR. Bisphenol A and human health: a review of the literature. Reprod Toxicol. 2013;42:132-155. doi:10.1016/j. reprotox.2013.08.008