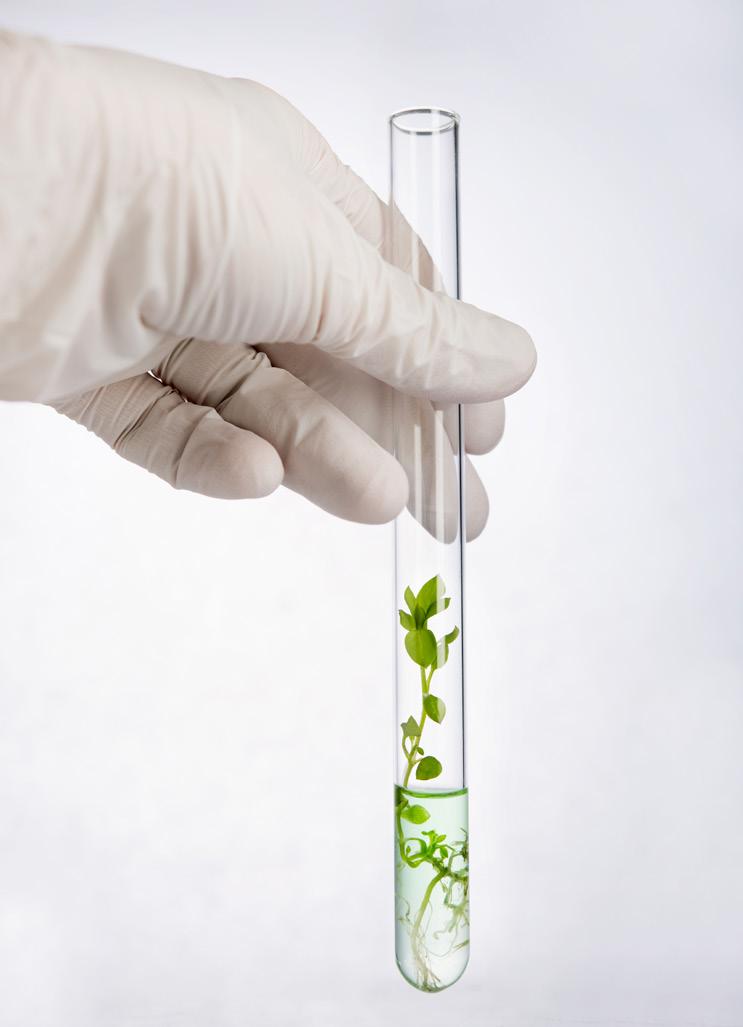
40 minute read
WORLDWIDE NEWS
Scientists have published the first complete, gapless sequence of a human genome two decades after the Human Genome Project produced the first draft human genome sequence.
Advertisement
The complete sequence, reported this April by the Telomere-to-Telomere (T2T) consortium, includes more than 3 billion base pairs across 23 chromosomes. The T2T consortium further used this newly completed genome sequence as a reference to discover more than 2 million additional genomic variants, opening doors to a comprehensive view of how human genomes vary, as well as for investigating how these newly discovered variants influence health and disease.
The T2T consortium includes leadership from researchers at the National Human Genome Research Institute (NHGRI), part of the National Institutes of Health; University of California, Santa Cruz; and University of Washington, Seattle. NHGRI was the primary funder for the study.
“Generating a truly complete human genome sequence represents an incredible scientific achievement, providing the first comprehensive view of our DNA blueprint,” said NHGRI director Eric Green, M.D., Ph.D.
The full sequencing adds to the Human Genome Project, which mapped about 92% of the genome. That last 8% includes numerous genes and repetitive DNA, comparable in size to an entire chromosome. Researchers generated the complete genome sequence using a human cell line with only one copy of each chromosome, unlike most human cells, which carry two copies of each chromosome. The researchers noted that most of the newly added DNA sequences were near the repetitive telomeres (long, trailing ends of each chromosome) and centromeres (dense middle sections of each chromosome).
The cost of sequencing a human genome using “shortread” technologies, which provide several hundred bases of DNA sequence at a time, is only a few hundred dollars, having fallen significantly since the end of the Human Genome Project. However, using these short-read methods alone still leaves some gaps in assembled genome sequences. The massive drop in DNA sequencing costs comes hand-inhand with increased investments in new DNA sequencing technologies to generate longer DNA sequence reads without compromising the accuracy.
Over the past decade, two new DNA sequencing technologies emerged that produced much longer sequence reads. The Oxford Nanopore DNA sequencing method can read up to 1 million DNA letters in a single read with modest accuracy, while the PacBio HiFi DNA sequencing method can read about 20,000 letters with nearly perfect accuracy. Researchers in the T2T consortium used both DNA sequencing methods to generate the complete human genome sequence.
New research from the University of California San Francisco has shown that a genomic variant in DNA linked with physical differences between sexes also points to disease risk. Some physical traits that differ between sexes are known to be linked to certain single nucleotide polymorphisms (SNPs) in chromosomes other than the X and Y chromosomes, with each SNP representing a difference in a certain DNA building block in a particular stretch of DNA. The researchers previously found that
SNPs associated with certain differences in physical traits between men and women—such as waist-hip ratio and basal metabolic rate—may also affect the biology of autism spectrum disorder and other complex diseases. Now, they have built on that work, leveraging two publicly available genome-wide statistical datasets to identify an updated list of 2,320 sex-heterogeneous SNPs.
STUDY FINDS CELLS TAKE OUT THE TRASH BEFORE THEY DIVIDE
MIT researchers have discovered that before cells start to divide, they do a little clean-up by tossing out molecules they may to need anymore. Using a new method they developed for measuring the dry mass of cells, the researchers found that cells lose about 4% of their mass as they enter cell division. The researchers believe this emptying of trash helps cells to give their offspring a fresh start without the accumulated junk of the parent cell.
Measuring the dry mass of a cell—the weight of its contents not including the water—is commonly done using a microscopy technique called quantitative phase microscopy. This technique can measure cell growth, but it does not reveal information about the molecular content of the dry mass, and it is difficult to use with cells that grow in suspension. To overcome the challenges, the researchers relied on a technique that measures the cells’ buoyancy as they float in normal water and then in heavy water. But because heavy water is toxic to cells, they designed a system in which cells could be measured repeatedly with minimal exposure to heavy water. The approach enabled them for the first time to track not just the dry mass of a cell, which is what others do using microscopic methods, but also the density of the dry mass, which gives information on the cell’s biomolecular composition. In the study, the team measured three types of cancer cells, which are easier to observe because they divide more frequently than healthy cells.
To their surprise, the researchers found that the dry mass of cells actually decreases when they enter the cell division. Additional experiments revealed that as cells enter mitosis, they ramp up the activity of a process called lysosomal exocytosis. Lysosomes are cell organelles that break down or recycle cellular waste products, and exocytosis is the process they use to jettison any molecules that aren’t needed anymore.
REVVING THE ENGINES OF CANADA’S GENOMIC MACHINE
A sampler of 10 Canadian genomic initiatives to watch
BY JANA MANOLAKOS
Despite a slower pace than other countries, Canada’s genomic research landscape is expanding, powering up Canada’s contributions to genetics and biotechnology in health, agriculture and the environment.
From precision health, which harnesses the potency of genome sequencing to diagnose and determine treatment of genetic conditions, to the barcoding of species, public and private sector organizations are pushing new horizons in genomics often as partners, unravelling the complexities of an organism’s genes in an effort to discover new therapies and applications.
Among these, Genome Canada, a government agency launched in 2000, spearheads a national strategy along with a network of six regional centres on the West Coast (Genome BC), the Prairies (Genome Alberta and Genome Prairie), central Canada (Ontario Genomics and Génome Québec), and the Maritimes (Genome Atlantic). Then there’s CGEn, a federally funded national platform for genome sequencing and analysis with nodes in Toronto (The Centre for Applied Genomics at The Hospital for Sick Children), Montréal (McGill Genome Centre at McGill University), and Vancouver (Canada’s Michael Smith Genome Sciences Centre).
Private sector start-ups have also staked their claim. Take Deep Genomics, which applies artificial intelligence in searching for life-saving genetic therapies; Precision Nanosystem, which is focused on gene function in neurons and glia cells; and DalCor Pharmaceuticals, which is genetically targeting patients that will benefit from precision treatments for cardiovascular disease.
Among an impressive array of genomic initiatives, here are 10 game changers working to put Canada on the map.
CANADA’S LARGEST HUMAN GENOME SEQUENCING INITIATIVE
Led by CGEn, the HostSeq databank will include the full set of DNA of 10,000 Canadians, with the goal of understanding how their genetics influenced their individual responses to COVID-19. The HostSeq databank also includes linked standardized clinical information collected at multiple clinical sites across Canada over the past two years of the pandemic. “The need for a national genomics databank grew very quickly early on in the pandemic,” explains Dr. Naveed Aziz, chair of the HostSeq Implementation Committee and Chief Executive Officer, CGEn. “As soon as we started to observe differences of disease manifestation and symptoms in people infected with SARS-CoV-2 virus, we realized that there was an urgent need to collect and analyze populationwide host genetic data.” The initiative, funded through Genome Canada’s Canadian COVID-19 Genomics Network (CanCOGeN), is accessible to Canadian and international researchers.
GENE MUTATIONS MAY HOLD THE KEY TO OVARIAN CANCER
Ovarian cancer affects about 3,000 women every year in Canada. Although most cases are sporadic, there are hereditary forms of ovarian cancer that are largely attributed to mutations in the BRCA1 and BRCA2 genes, which are also involved in breast cancer. However, some families with no known cancerpredisposing genes develop multiple cases of ovarian cancer, suggesting that mutations in unknown genes remain to be discovered.
With this in mind, scientists at the Research Institute of the McGill University Health Centre (RI-MUHC) set out to investigate new genes that could be associated with a risk of ovarian cancer. Their findings show that a mutation in a specific protein gene known as FANCI (FA Complementation Group I) is more common in ovarian cancer patients with a family history of ovarian cancer than in cancer-free people, suggesting that it may influence the risk of developing that cancer. Dr. Patricia Tonin, principal investigator of the study and senior scientist in the Cancer Research Program at the RI-MUHC, explains: “This study emphasizes the importance of pursuing variants during the gene discovery phase, especially when plausible gene candidates are revealed by analyses of defined cancer families.”
The researchers performed genetic testing on two sisters who had ovarian cancer and a family history of ovarian cancer. Next-generation sequencing showed that both subjects carried a mutation in the FANCI gene. The researchers then sequenced familial cases of ovarian cancer and compared variant frequencies in people with and without ovarian cancer in a population pool of French Canadians from Québec. This population has a unique genetic landscape inherited from common ancestors that arrived in Québec from Western Europe as early as the 1600s.
The study authors also point out that other genetic, environmental or host factors could affect the risk of ovarian cancer in carriers of FANCI variants. Replication studies are needed to confirm these results in larger groups worldwide. In addition, studies to clarify the cancer risk conferred by FANCI variants are required to inform medical genetic screening practices for managing risk and ovarian cancer.
NEW NATIONAL PLATFORM ADVANCES CLINICAL GENOMIC TESTING
Canadians struggling to get a diagnosis will now have access to state-of-the-art genome-wide sequencing thanks to a new pan-Canadian All for One precision health partnership led by Genome Canada. Already deployed across six implementation projects serving nine provinces, the $39-million All for One initiative includes $13 million in federal investment through Genome Canada and $26 million in co-funding from industry, health-care organizations, provincial governments, and other partners brought in through six regional Genome Centres.
CANSEQ150
To commemorate Canada’s sesquicentennial in 2017, CGEn and its partners embarked on the Canada 150 Sequencing Initiative (CanSeq150) aimed at sequencing 150 new genomes to support sequence-based genomics research in Canada in biodiversity and conservation, applications in breeding and biomedicine, and technology development. More than 100 species were selected for sequencing through the CanSeq150 program, providing a platform for biologists, ecologists, population geneticists and other scientists to work with genomic scientists. CanSeq150 has now joined the Canadian arm of the Earth BioGenome project (featured in the spring issue of BioLab Business), which will identify an additional 400 species important to Canadian wildlife conservation, recovery and monitoring.
BRIDGING THE GENOMIC DIVIDE FOR INDIGENOUS CHILDREN
Although the rapid development of genomic technologies is advancing precision medicine, it is also widening the health inequity gap for Indigenous populations that often have little or no access to genomic technologies and the research that drives them. This prevents accurate diagnosis because of the absence of the reference data needed for precise genetic diagnosis. Working with Genome BC, Genome Canada last year launched Silent Genomes, a partnership with First Nations, Inuit and Métis peoples to enable access to diagnosis and treatment of genetic disease for their children. The project will establish processes for Indigenous governance of biological samples and genomic data, lead to policy guidelines and best practice models for bringing genomic testing to Indigenous children, and develop an Indigenous Background Variant Library of genetic variation from a diverse group of First Nations.
NEW NON-HUMAN GENETIC DATA BANK LEVERAGES ARTIFICIAL INTELLIGENCE
This past April, Génome Québec and Université Laval launched Genovalia, the first centre in Québec dedicated to the production, processing, and application of non-human genomic data in agri-food, forestry and environmental sciences. Bolstered by Université Laval’s artificial intelligence experts, Genovalia aims to contribute to standardization in data collection, increase information sharing, grow computational capacity and develop better analytical tools.
KEEPING FEEDER FISH AND THE SALMON INDUSTRY HEALTHY
Sea lice, which feed on the skin of living salmons, are a big problem for Canada’s commercial Atlantic salmon industry, costing producers $18 million last year alone in lost stock. To mitigate the problem, feeder fish like lumpfish are introduced to salmon tanks to eat the tiny crustaceans. However, lumpfish are highly susceptible to Vibrio anguillarum, a bacterial pathogen which causes haemorrhagic septicaemic disease in the fish. Keeping them healthy is the focus of genomic research at Memorial University’s Ocean Science Centre (OSC) in St. John’s, Nfld., where a research team, led by Javier Santander, a marine microbiologist and Associate Professor in the Department of Ocean Sciences at Memorial, has been sequencing lumpfish and cunner genomes. Santander believes the team is close to solving the lumpfish’s high susceptibility to the pathogen. “We have an effective vaccine that has been tested several times and soon will be evaluated in the field,” he said. His research group is now processing the data for transcriptomic profiling of the lumpfish response to the effective immunization.
FOUR GENOMICS AND ENGINEERING BIOLOGY TEAMS WIN FUNDING FOR CELLULAR AGRICULTURE INITIATIVES
Cellular agriculture encompasses several innovative approaches that use cell cultures, tissue-engineering, or precision fermentation-based techniques that push beyond conventional production. This past May, four genomics and engineering biology projects won more than $900K in funding from AcCELLerate-ON, Canada’s first regional cellular agriculture competition in support of food innovation in Ontario. Ardra Inc. was named for its development of fermentation-based production of heme, a natural flavour ingredient that mimics the taste of meat. Cell Ag Tech won for scaling up the manufacturing of fish muscle stem cells from a 2D to 3D culture system with proteomic assessments of the cells. The third recipient, Evolved, won for creating cultivated pork belly that is identical to conventional pork belly. The University of Toronto’s Dr. Michael Garton, in collaboration with MyoPalate, shared in the prize for establishing the foundational tools for cultivated pork production. The funding comes from Ontario Genomics and the Canadian Food Innovation Network.
KEEPING AHEAD OF GLOBAL COMPETITORS WITH SPECIALIZED LENTILS
Canada grows more than 3 million tonnes of lentils annually, more than half of the world’s total supply. Nearly all (about 95%) of Canada’s lentil crops, which produced almost $3 billion in export revenue in 2021, are grown in Saskatchewan. But global competition is heating up, so researchers working on the EVOLVES (Enhancing the Value of Lentil Variation for Ecosystem Survival) project at the University of
Saskatchewan are looking at growing specialized lentils.
A significant part of the project is improving the genetic variability, phenotyping and gene sequencing of both cultivated and wild lentils. EVOLVES also takes an ‘omics (combining genomics and phenomics) approach so traits that are prized by exporters like greater nutritional content, flavour and climate resistance can be zeroed in on to produce specific varieties. Field trials will begin this summer in Saskatchewan and southern Italy. The initiative is supported by Genome Prairie, Saskatchewan Pulse Growers, Western Grains Research Foundation, and Agriculture and Agri-Food Canada.
GENOMIC TOOLS WILL HELP SUPPORT BETTER MITE RESISTANCE
Honey bee colony collapse is a significant problem for both Canada and the world because of the insect’s vital importance to pollination and food production. Unfortunately, the mite Varroa destructor feeds on bees at adult and juvenile stages, weakening them and transmitting deadly viral infections that can lead to colony collapse. Recently, a new acaricide that is poisonous to mites like the Varroa but does not harm bees and vertebrates was introduced to the industry. A Genome BC-funded project aims to discover the efficacy of this new compound. Researchers are using proteomics tools to identify the molecular target and determine how, when and where the compound can be used. The project will run until September 2023 so that early learnings can be applied to the next cycle of field testing.
GETTING TO THE ROOT OF THE TREE OF LIFE
The Canadian BioGenome Project is an ambitious undertaking that aims to map the complete genome of plants and animals
BY SEAN TARRY
When it comes to scientific pursuits, there aren’t many that match the ambition, breadth or significance of the Canadian BioGenome Project. Established as part of the larger Earth BioGenome Project, it’s an undertaking that aims to map the genomes of all plants, animals, fungi and other microbial life — an effort to, in effect, catalogue the complete genetic diversity of Canada's plants and animals through genomic sequencing. The sheer magnitude of the project is not lost on Steven Jones, Co-Director of Vancouver’s Genome Sciences Centre and CoLead Investigator for the Canada BioGenome Project. However, he says equal to the amount of work that will be required to complete this endeavour is its importance to our ability to further our collective understanding of life on Earth.
“What we’ve just started in our attempt to sequence the genomes of Canada’s plants and animals presents an enormous challenge, which will require a great deal of work,” he says. “It will also require a tremendous amount of collaboration between individuals within the science community in order for us to achieve our objective. It’s a massive project that poses huge potential benefits in the way of enhancing our understanding of the evolution of life and uncovering fundamental genetic principles of health and disease that will help populations everywhere.”
COLLABORATIVE EFFORT
Beginning as a consortium of sequencing laboratories across the country, which includes the Genome Sciences Centre in Vancouver, Toronto’s Hospital for Sick Children, and McGill University in Montréal, the project has grown to rely on a host of experts whose insights have been sought in order to guide and direct the research and work being conducted. There are just over an estimated 80,000 species of plants and animals that are known to inhabit Canada from coast to coast to coast. Jones and the Genome Project are starting with the sequencing of between 400 and 800 species. Experts, Jones says, are helping to narrow the project’s focus.
“We’ve got a group of a few hundred or so scientists located right across the country who are all experts concerning very specific types of animals and plants, like conifers or crustaceans,” he says.
“They’re helping us to identify the species that are high priority for sequencing. We can’t possibly sequence every species of plant and animal across the entire country right away. So, we’ve got to choose wisely to start. It really comes down to understanding and being aware of the parts of the tree of life that are underexplored, identifying the plants and animals for which no genetic understanding exists and prioritizing them as such.”
SUPPORTING TECHNOLOGY
Jones goes on to explain that in addition to filling out the tree of life, there are also other factors considered when prioritizing the sequencing of species, including scientifically interesting plants or animals that may interact with humans and possess unique properties, as well as species that have become endangered as a result of climate change.
In addition, he suggests that it may also be just as important to sequence and study the prey of the endangered species to further understand why the numbers of some predators are diminishing. In fact, there is a whole web of criteria that inform decisions made by scientists feeding into the Canada BioGenome Project. Supporting much of the human effort, Jones explains, is cutting-edge technology that is serving to elevate and improve the work being conducted.
“The major type of technology that we use is obviously related to DNA sequencing,” he says. “It’s recently become a much more critical and useful tool. Its potency and power have increased, and the prices have come down significantly since the first human genome was sequenced at the turn of the century. It is rapidly improved technology that is now much more accessible for this type of work, allowing us to do more, quicker, faster, cheaper and more efficiently.”
THE POWER OF ARTIFICIAL INTELLIGENCE
It is estimated that the sequencing of the first human genome took in excess of 15 years and cost somewhere in the range of US$2.7 billion. Today, Jones and his team of scientists can routinely sequence genomes in a matter of days for a little more than CDN$1,000. And, in addition to the vastly improved technology, advancements related to artificial intelligence are going a long way toward supporting efficiencies and breakthroughs within the study and research of genomics and
This poses huge potential benefits in the sequencing of species. the way of enhancing In particular, Jones points to the recent introduction of AlphaFold, our understanding of an artificial intelligence program the evolution of life and developed by Google which, informed by deep learning, presents the ability uncovering fundamental to perform predictions concerning genetic principles of protein structure, among other things. “One of the greatest advancements health and disease. over the course of the past 24 months or so is the AI that’s coming out of Google’s AlphaFold project,” he asserts. “If we can sequence the genes, Google’s AI will then predict the threedimensional structure of the protein that the gene creates. It represents a huge step forward, allowing us to, for instance, sequence the genome of a bee, and with a couple weeks of computation will be able to produce the three-dimensional structure of every protein in that bee. It helps us better understand things like how the bee is interfacing with its environment and perhaps design a new insecticide that doesn’t impact the bee population. It’s quite incredible that we are now, through sequencing, able to generate a parts list of each plant and animal, and through Google’s technology can understand the precise shape of each part. It’s going to continue to be a key technology that we use going forward.”
OPEN DATA ECOSYSTEM
Google’s technology will also be key in helping Jones and his team of scientists fulfill one of the mandates of the Earth BioGenome Project, which is to freely share with the public all of the data and information that’s generated through sequencing. To do this, data is submitted to collaborators in Europe who annotate it in a way that’s consistent with all of the other genomes being created around the world as part of the project. It is an approach that allows everyone involved to operate within an open data ecosystem, enabling its full access and use to anyone on the planet, posing a multitude of potential future benefits. It is one of the core concepts of the project, says Jones, and one of its aspects that makes it such an exciting one to work on. “It really is quite an incredible project to be a part of. And only a few months in, our work is only now beginning in earnest. The initial phase of the project is scheduled to be a duration of four years. We’re hoping that in that time we’re able to, through our work, show significant progress in our sequencing efforts. In addition, over this time, we’re anticipating further technological advances that will make the use of these tools even more accessible and financially viable, allowing us to continue deepening our understanding of the vast Canadian ecosystem.”
In a Canadian first,
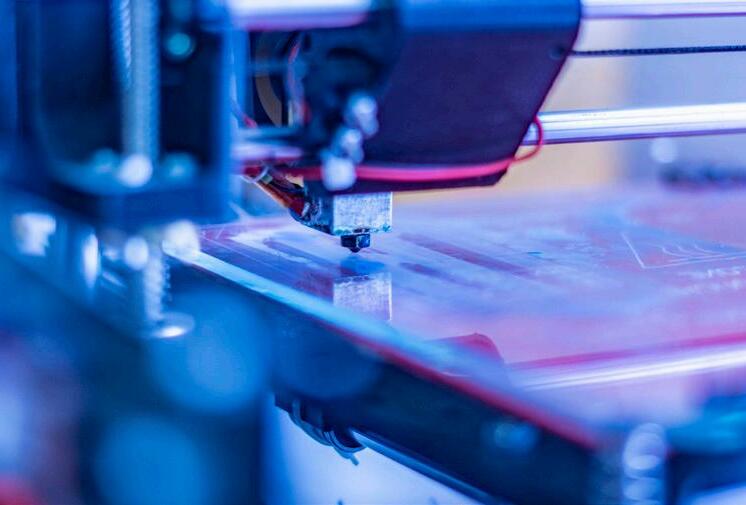
UBC scientists 3D print human sperm
Science has come a long way since the first blood vessels were bioprinted in 2010, successfully printing cartilage, bone, cardiac, nervous, liver, and vascular tissues. Recently, scientists at the University of British Columbia added to that list with 3D bioprinted human testicular cells, a Canadian first that offers hope for men with a severe form of infertility known as non-obstructive azoospermia (NOA).
According to UBC, the scientists, led by UBC urology assistant professor Dr. Ryan Flannigan, suggest that the technique may one day offer a solution for people living with currently untreatable forms of male infertility.
“Infertility affects 15% of couples, and male factors are a contributing cause in at least half of those cases,” said Dr. Flannigan, whose lab is based at the Vancouver Prostate Centre at Vancouver General Hospital.
“We’re 3D printing these cells into a very specific structure that mimics human anatomy, which we think is our best shot at stimulating sperm production. If successful, this could open the door to new fertility treatments for couples who currently have no other options.”
Within human testicles, sperm is produced by tiny tubes known as seminiferous tubules. In NOA, no sperm is found in ejaculate due to diminished sperm production within these structures.
While in some cases doctors can help NOA patients by performing surgery to find extremely rare sperm, Dr. Flannigan says this procedure is only successful about half the time. “Unfortunately, for the other half of these individuals, they don’t have any options because we can’t find sperm for them.”
Those are the patients Dr. Flannigan’s team is hoping to help.
For the recent study, the researchers performed a biopsy to collect stem cells from the testicles of a patient living with NOA. The cells were then grown and 3D printed onto a petri dish inside a hollow tubular structure that resembles the sperm-producing seminiferous tubules.
“3D bioprinting is a highly customizable platform that gave us control on the largest number of variables to facilitate
favourable cell-cell interactions and recapitulate in vivo cytoarchitecture like we see naturally in the human body,” explains Dr. Flannigan.
The team used a 3D printer manufactured by Aspect Biosystems, which also supplied the proprietary bioink in which the cells were mixed. To determine the precise amount of biomaterial for the ink, as a starting point they relied on previously published data on cell density required for 3D bioprinting, explains Dr. Flannigan, and “then performed several series of optimization experiments of cell concentration, and printing parameters to achieve the structure achieved in this publication.”
To shape the 3D printed cells, they used a coaxial needle that consisted of a sacrificial core material (polyvinyl alcohol–PVA 6%) with cells situated in AGC10 bioink in the outer shell to achieve the tubular structure.
Twelve days after printing, the team found that the cells had survived. Not only that, they had also matured into several of the specialized cells involved in sperm production, and they were showing a significant improvement in spermatogonial stem cell maintenance — both early signs of sperm-producing capabilities. The results of the study were recently published in Fertility and Sterility Science.
“It’s a huge milestone, seeing these cells survive and begin to differentiate. There’s a long road ahead, but this makes our team very hopeful,” said Dr. Flannigan.
The team is now working to “coach” the printed cells into producing sperm. To do this, they’ll expose the cells to different nutrients and growth factors, and fine-tune the structural arrangement to facilitate cell-to-cell interaction.
If they can get the cells to produce sperm, those sperm could potentially be used to fertilize an egg by in vitro fertilization, providing a new fertility treatment option for couples.
Dr. Flannigan’s research program has also been shedding new light on the genetic and molecular mechanisms that contribute to NOA. They’ve been using various single-cell sequencing techniques to understand the gene expression and characteristics of each individual cell, then applying computational modelling of this data to better understand the root causes of the condition and to identify new treatment options. The work has been highly collaborative, involving UBC researchers across computer science, mathematics and engineering, as well as international collaborations.
“Increasingly, we’re learning that there are likely many different causes of infertility and that each case is very patient-specific,” said Dr. Flannigan. “With that in mind, we’re taking a personalized, precision medicine approach. We take cells from a patient, try to understand what abnormalities are unique to them, and then 3D print and support the cells in ways that overcome those original deficiencies.”
This research is supported by Michael Smith Health Research BC, the Canada Foundation for Innovation, Canadian Institute of Health Research, Vancouver Coastal Health Research Institute, American Society of Reproductive Medicine, Canadian Urologic Association Scholarship Foundation and the Vancouver Prostate Centre.
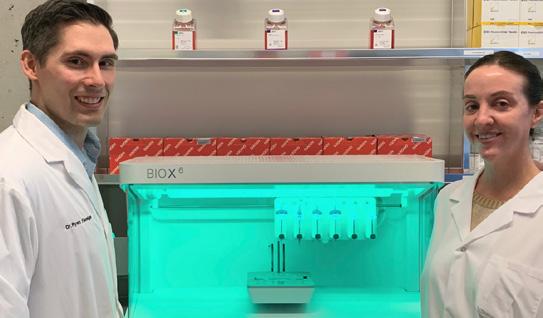
Dr. Ryan Flannigan and research assistant Meghan Robinson with the bioprinter they’re using to 3D print copies of a patient’s testicular cells. Photo Courtesy: University of British Columbia
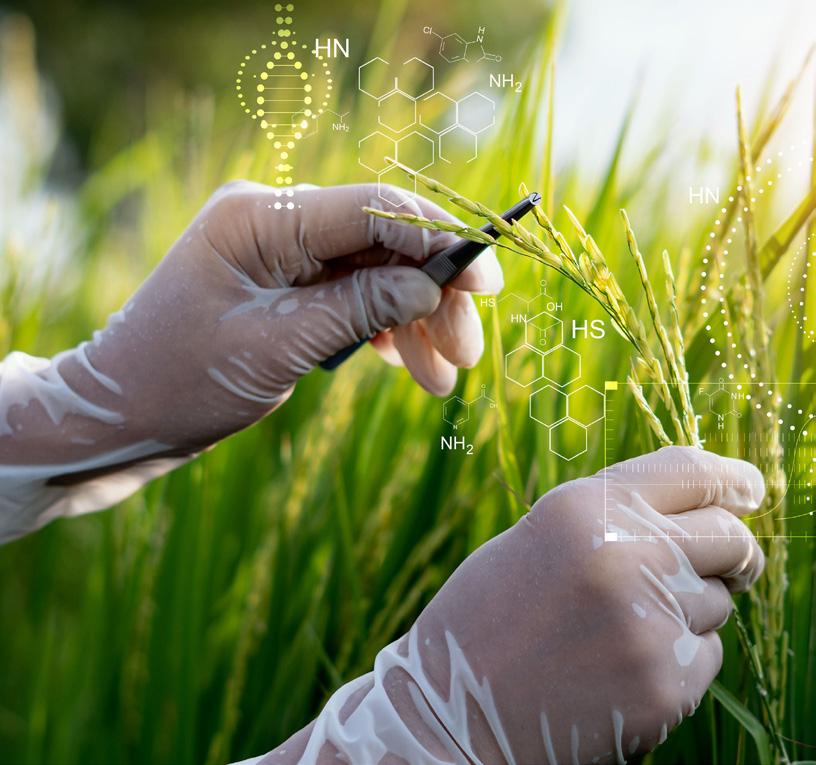
MORE THAN NOAH’S ARK
PLANT GENE RESOURCES OF CANADA: A LIVING LIBRARY
BY ROBERT PRICE
Axel Diederichsen has a scientist’s sense of humour. A research scientist and curator at Plant Gene Resources of Canada (PGRC), he recalls one of the more thrilling moments in his career. While studying cultivated flax, a plant only known to have blue flowers, he found a wild relative with white flowers. “Botanists can get excited about these things,” he laughs.
As a curator at PGRC—a government agency tasked with preserving and enhancing “the genetic diversity of cultivated plants of importance for Canadian agriculture and their wild relatives”—Diederichsen stays close to the excitement found in watching the grass grow.
It’s quiet work. It’s also enormous importantly work. As a repository for the seeds, tubers and cuttings of Canadian agriculture reaching back to the 1960s, the PGRC might look a bit like Noah’s Ark—a life raft carrying the sum of genetic
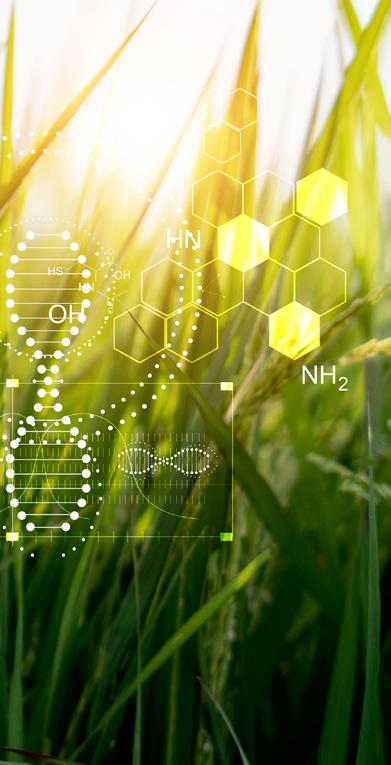
diversity produced by farmers. But such a comparison wouldn’t capture the whole story. As Diederichsen explains, the PGRC is “more than Noah’s Ark.”
It is, in fact, more like Borge’s magical library that collects every sequence of words ever written. Instead of words, PGRC catalogues plant genetics. PGRC is, Diederichsen says, a place “where you find exotic things”—all of it packed in tens of thousands of envelopes and waiting to be brought to bloom.
A living library
PGRC, a part of Agriculture and Agri-Food Canada, operates three genebanks. A bank in Saskatoon stores seeds and grains; a bank in Fredericton collects tubers for potato farming; and a bank in Harrow, Ont., houses materials needed to help Canada’s orchards and fruit patches thrive. In each location, scientists house and study seeds, cultivars of landraces (crops that have been improved through farming), obsolete cultivars and wild relatives of seed relevant for agricultural production. The scientists at PGRC regenerate plants, describe the basic botanical features of the seeds they have on file, and make these seeds available for research, breeding and education through online databases, publications and work the organization does with agricultural scientists.
PGRC has close to 114,000 accessions—plant material collected from a single location—with a strong focus on cereals, a key component of Canadian agriculture. Around 80% of the collection comes from cereals, including 40,000 different barleys (both cultivated and wild species) and 48,000 samples of oats. The genebank seeks to capture a complete portrait of cultivars bred in Canada and solicits donations from breeders who retire or give up all or part of their agricultural programs. “They have working collections with interesting material, some of it very difficult to find,” says Diederichsen.
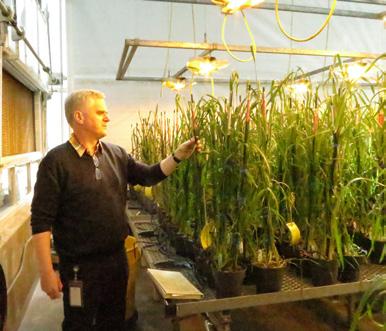
Images provided by Plant Gene Resources of Canada (PGRC), ©Her Majesty The Queen in Right of Canada, as represented by the Minister of Agriculture and Agri-Food, licensed under the Open Government Licence – Canada
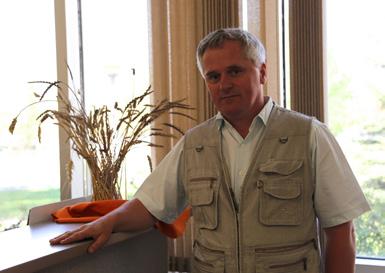
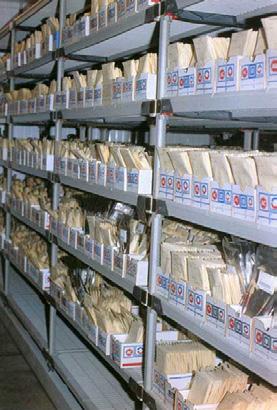
The donations will eventually find their way back to Canadian farms. Successful agriculture relies on genetic diversity—a value that must be constantly renewed since it’s so easy to lose. Three hundred years ago, for example, villages across Europe farmed different types of wheat, barley, or oat, each type adapted to local conditions. Over time, farmers singled out the most successful grains, and soon all farmers were cultivating these grains.
“So we lose the reservoir of diversity we depend on,” says Diederichsen.
PGRC works to buffer against this loss of diversity, by ensuring a diverse set of genetic diversity remains accessible to farmers and scientists, even if particular seeds aren’t currently used for agricultural purposes—and haven’t been planted in decades.
Genetic diversity is a strength
Genebanks have a long history. Medieval monasteries cultivated and collected cereals and medicinal plants, and in the 16th century Italian botanical gardens cultivated and traded exotic
plants. With the advent of modern plant breeding some 150 years ago, plant breeders quickly learned that if they wanted to create something new, they needed a diverse collection of seeds to breed and crossbreed. But, as Diederichsen recounts, as breeders became successful at creating new cultivars that produced higher yields, the older, locally adapted crops that farmers had used for hundreds of years started disappearing.
Plant breeders knew what the extinction of older crops meant: the loss of information and a loss of crop vitality.
“Plant breeders actually said we need living genebank collections [to preserve information],” Diederichsen says, “because even if it’s not suited today, it may carry traits we need for doing something.”
Genebanks like PGRC serve an important role in preserving—in living form—the genetic heritage of each crop. The genebank ensures the genetic integrity of each sample in the library—the material remains frozen in time, preserved in the state it was when it was collected. Unlike traditional farming that relied on a farmer’s experience with a crop, today’s geneticists can scour the genetic histories of individual crops— moving backwards in time, so to speak— to find older generations of a particular crop that might have resistance to specific diseases.
Nicolas Tinker, a senior research scientist at Agriculture and Agri-Food Canada who uses the collections at PGRC as part of his bioinformatics research, describes this ability to search the family trees of different plants as a primary driver for seed libraries that might, to uncreative minds, seem like esoteric lines on a balance sheet.
“In oat, in particular, there’s a disease called crown rust that sweeps through and defeats the genes that we have, and if we don’t keep going back to the genebank and finding new sources of resistance to that disease, we lose the crop,” says Tinker.
“We keep genebanks going because we don’t know what we’re going to get out of them in the future, and that’s the conundrum.”
Browsing other libraries
To extend the diversity of crops around the world, PGRC works with genebanks in other countries on international initiatives geared to preserving diversity in cultivated plants. These genebanks exist all over the world, each with a different focus. Norway’s Svalbard Global Seed Vault, for instance, holds more than 1 million seed samples of some 5,400 species in an underground storage facility. The International Rice Genebank in the Philippines, which is the largest bank of rice genetics in the world, holds more than 132,000 rice accessions.
Like PGRC, these genebanks push data about plant genetics into the public realm. PGRC has a sizeable database that allows clients to see what material the bank has available to the scientific community nationally and internationally. Scientists inside and outside the genebank research the PGRC’s holdings for certain traits and publish their findings, and with climate change threatening crops, study of the molecular composition of the collection intensifies as breeders look to adapt crops to changing environments. Currently, a research team is studying 30,000 accessions of PGRC’s genebank and will describe the genetic makeup of these individual accessions using the sequencing technologies.
Safety against the future
As the climate changes and food producing nations feel the pinch of inflation, fertilizer shortages, and political unrest, the cultural and political environment seem ripe for longer and deeper discussions of how organizations like PGRC can diversify agriculture and ensure crops remains resilient and plentiful.
“Ultimately, our food security nationally but also globally depends on having access to this diversity,” Diederichsen says.
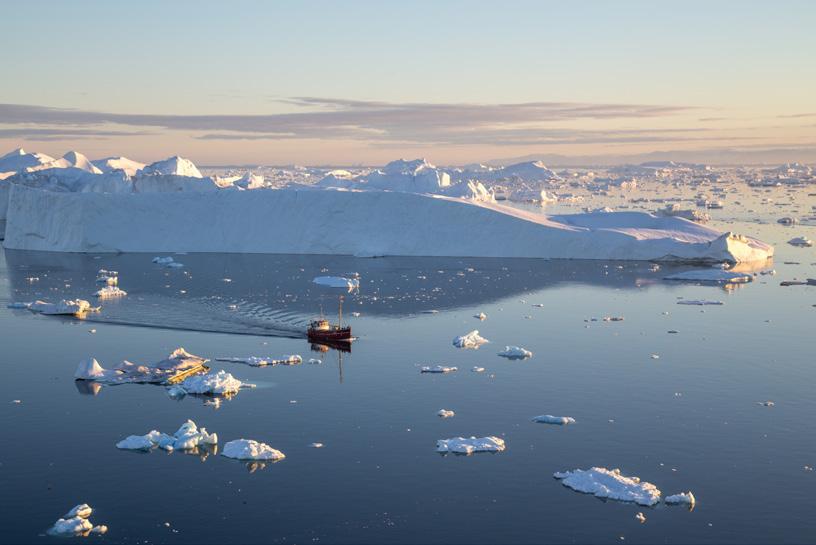
New DNA insights will transform Arctic marine biodiversity and fisheries management
BY BRIAN BURKE, NUNAVUT FISHERIES ASSOCIATION & CARON HAWCO, EDNATEC
Indigenous fishing enterprises are playing a key role in the application of genetics research to support the commercial fishery of Canada’s North. This has the potential to address the significant knowledge gaps that currently exist relating to Canada’s biodiversity in the North. Traditional environmental programs have typically been limited due to the challenges of operating in remote and harsh Arctic conditions.
The Nunavut Fisheries Association (NFA) and environmental genomics specialists at eDNAtec have partnered to bridge this knowledge gap. The focus is to build on their Canada’s Ocean Supercluster project, OceanDNA System, integrating Indigenous knowledge with eDNA research to transform commercial fisheries research by involving Indigenous fishers directly in the research process. In September 2020, eDNAtec, working with the NFA, Petroleum Research Newfoundland and Labrador (now Energy Research and Innovation Newfoundland and Labrador), and the federal Department of Fisheries and Oceans (DFO) launched the OceanDNA System project to assess, monitor and characterize the ocean. It was financed by Canada’s Ocean Supercluster to the tune of $2.2 million with additional funding of $2.7 million from the project partners.
All organisms shed environmental DNA (eDNA) into their surroundings. The eDNA in one water sample can reveal an abundance of data about the variety and number of organisms present in an ecosystem and can be completed in a fraction of the time required for conventional field sampling.
Through the OceanDNA System project, eDNA sample collection tools can now be easily deployed on operating fishing vessels where crews are trained to collect samples from marine ecosystems, which are then analyzed with eDNA sequencing systems. “We have been building capacity using environmental DNA (eDNA) water sampling techniques on our commercial and research vessels,” said NFA Executive Director Brian Burke. “This research, which is taking place in the harsh conditions of Canada’s Eastern Arctic, is being incorporated into NFA’s annual fisheries research and tracking programs to improve understanding of the marine environment and management of fishery resources.” Expected to be completed by the end of 2022, the OceanDNA System project developed eDNA workflows and procedures that supported the integration of eDNA technology with conventional biodiversity assays and Indigenous knowledge, providing an improved understanding of Northern ocean biodiversity through the following advancements: 1. Development of field protocols that are straightforward and easy to learn, with equipment that works reliably without requiring technical expertise, particularly when collecting water samples without introducing other contaminants, such as human DNA, which can complicate lab analysis.
2. Identification of targeted commercial species and
bycatch so their DNA is pre-identified, making for a quicker and more efficient analysis of samples. Target species included Greenland Halibut, Greenland Shark,
Arctic Skate, Arctic Cod, Narwhal, two species of Northern
Shrimp, two types of Redfish, and two types of Grenadier. 3. Abundance of target species. This research is based on work conducted in related fields, including medical genetics, to conclude not just what species are present, but the relative abundance of each species present.
4. Understanding genetic diversity within species.
Related to stock assessment, determining if populations of certain stocks are one distinct population that can be managed as one entity, or if there are discrete populations
By improving that are separate and don't interecosystem knowledge, mix. For example, Greenland Halibut is currently considered to be one Northern communities population that has a very broad can be more geographic distribution. We are now using environmental genomics to involved in directing the environmental determine if this species is actually represented by two or more distinct populations. stewardship of their 5. Ecosystem modeling. All coasts, including the information is brought together,
Marine Protected layered and applied to define species extent and density across the entire Areas. ecosystem area. This model provides a better understanding of how various factors, such as water depth, temperature and nearby structures affect the populations of target species. According to eDNAtec CEO Steve Barrett, eDNA sampling can now be easily done by non-scientists in remote communities and is proving to be safer, faster and less expensive than ever before. “This technology also aligns with Inuit philosophy that ecosystems must be viewed as a whole versus by individual species,” he added. “By improving ecosystem knowledge, northern communities can be more involved in directing the environmental stewardship of their coasts, including Marine Protected Areas.” “We are integrating Indigenous knowledge with eDNA to improve stock management, invasive species detection and biodiversity assessments,” said Burke. “This will build on our existing research and is not only important for NFA’s offshore fishery but also for assessing inshore fishery potential for communities in the Qikiqtani region and across Inuit Nunangat.” The Nunavut Fisheries Association NFA is a non-profit organization established to provide a unified industry voice for Nunavut’s fishing industry. NFA represents four Inuit-owned quota holders, including the Arctic Fishery Alliance, Baffin Fisheries, Qikiqtaaluk Corporation and the Pangnirtung Fisheries/Cumberland Sound Fisheries Partnership. Specialists in environmental genomics, eDNAtec is transforming how to assess, monitor, and characterize ecosystems through DNA sequencing, which enhances environmental stewardship of our planet’s marine and terrestrial biodiversity. eDNAtec works closely with the world’s ocean industries and organizations with an interest in them, including energy, fisheries, and the Government of Canada.
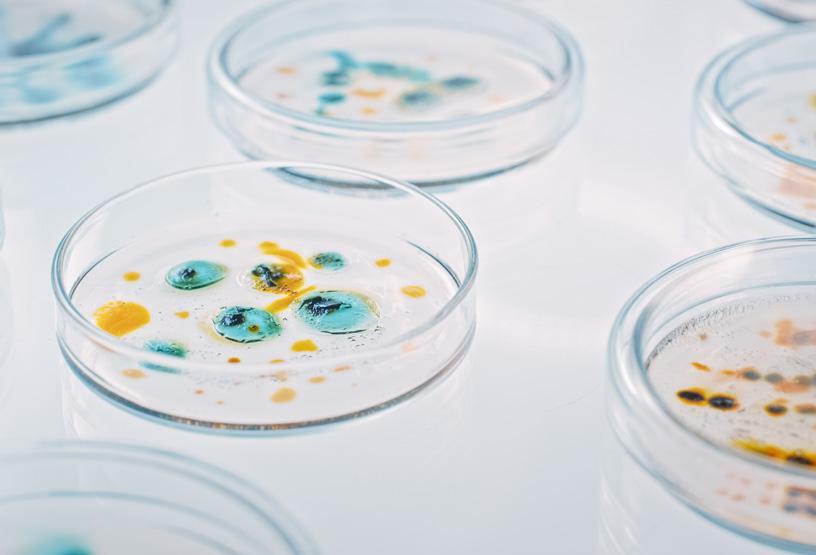
SENSING BIODIVERSITY:
VACUUMING MAMMAL DNA FROM THE AIR
A geneticist uses a simple pump to filter microscopic genetic samples from air. The approach is a novel way to monitor biodiversity, identify species interactions and assess changes in the ecosystem.
BY REHANA BEGG
Take a deep breath. Now consider the air you’ve inhaled…
Along with the oxygen, air contains nitrogen and carbon dioxide, as well as ozone, smoke, dust and pollen. A lesser-known fact is that air molecules could also contain trace amounts of animal DNA.
That’s according to Dr. Elizabeth Clare, geneticist and assistant professor at Toronto’s York University, who recently published a proof-of-concept study outlining a method for detecting the presence of animal DNA in air several hundred meters from the source.
Her research is not only a step toward validating the presence of hard-to-detect mammals but has the added benefit of being non-invasive — an essential factor when dealing with endangered species. “There is a risk associated with interfering with endangered populations or, if I catch one of them, it’s a stressful experience for both them and me,” says Clare.
Environmental DNA (eDNA) is any DNA not taken directly from an animal or a plant. This method for collecting DNA samples is growing, says Clare, who previously sequenced eDNA to monitor fish, iDNA in leeches to monitor invertebrates, and DNA in feces to
work out predator-prey and seed dispersal relationships. “We’re gradually moving away from catching the animals to capturing the thing that touches the animals and moving to going directly to the environment.”
For the “airDNA” study, 72 samples were collected at targeted locations at the Hamerton Zoo Park, a 25-acre conservation zoo in Huntingdonshire, UK. “We were even able to collect airDNA from animals that were hundreds of metres away from where we were testing without a significant drop in the concentration, and even from outside sealed buildings,” says Clare. “The animals were inside, but their DNA was escaping.”
After analyzing the samples, the team identified 25 species of mammals and birds. Seventeen were known species. Squirrels, ducks, and hedgehogs from the surrounding rural area were also detected in the count. The range of species detected, notes Clare, validates the potential use of airborne eDNA in detecting and monitoring biodiversity.
If there’s a downside to environmental sampling, then it has to be that one cannot collect a clean sample. “There’s lots of DNA in my sample, and we’re still working on how to do it with air,” explains Clare.
Samples in the experiments were also dispersed (as opposed to concentrated) and could not provide detail beyond identifying individual species. Exposure to ultraviolet light (such as that from the sun), temperature changes and age of the animal can cause degradation of the DNA.
Still, for Clare, the objective is largely to detect the diversity of species in a given ecosystem, rather than detecting a single species.
COLLECTING SAMPLES
Current sample collection methods are anything but foolproof and Clare’s team continues to work on perfecting a method to filter the DNA from the air. “We’re trying different things, but mostly, we suck air through very fine filters,” she says. “It’s a bit like filtering coffee. We’re trying to pull air through a filter and track particles, such as dead skin cells and bits of hair and free-floating DNA from the air sample.”
To collect airDNA samples, Clare uses a Geotech peristaltic pump, which moves air through a flexible tube. Air drawn from the outside moves through Sterivex-HV filters (Merck Millipore) at the intake end of the tube before moving into the hose. The soiled filters are then placed in sterile bags and frozen until DNA extraction. The samples are lysed overnight and centrifuged for extraction.
Once the DNA is extracted, a technique called polymerase chain reaction (PCR) is used to inspect the DNA sequences and compare the results to DNA databases. The raw DNA amplicon data is then deposited at the NCBI Sequence Read Archive, a large, publicly available repository of high- throughput sequencing data.
“This is just a natural process that we have co-opted in a lab, so that we can target and make copies of something very specific,” explains Clare. “I can design a test for elephant DNA and I can just assay for elephants. I can’t really distinguish which elephant, but I can probably design something that will amplify elephant DNA and nothing else.”
PUMP AIR
Rather than use a vacuum pump to collect the air samples, Clare uses a suction pump to siphon water through a filter. “Vacuums are loud and we were constrained by the noise,” explains Clare. “We were trying to be non-disturbing to the animals. Our pump was almost silent. It was not efficient, but it worked. All we were trying to show is that it was possible.”
Clare is currently testing more powerful vacuum systems. Since she carries her gear into rugged terrain, the pumps need to be lightweight, portable, battery-powered and affordable. One company offered her a commercial sampler at the cost of $15,000, she says. Given the prohibitive cost, Clare decided to engineer her own air-pumping device. “I’ve got seven different prototypes that I’m going to test,” she says. “I’m simply trying to make something that students and I can haul out in the middle of nowhere and fix when it goes wrong.”
As with most research, Clare’s team has a few unanswered questions. How far does it travel? How quickly does it accumulate? How quickly does it degrade?
Plans to address these questions are in the offing, as is finding better technology solutions. “We do have an engineering problem, which is trying to figure out how to sample large amounts of air efficiently,” muses Clare. “We haven’t gotten to that yet. This is very early in this type of technology.”
At the same time Clare was conducting the airDNA research, a second research team in Copenhagen was working on an identical question. Rather than run the risk of scooping each other, the teams co-ordinated their research and published the findings simultaneously. “We purposely didn’t talk about what we’re doing; we only talked about the process,” says Clare. The upshot, she says, is that the work was twice validated.