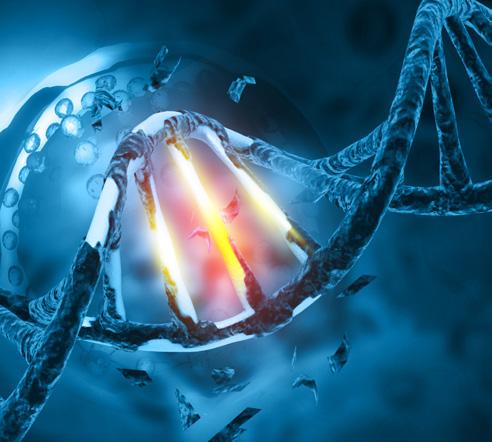
6 minute read
FEATURE
Seeing through crystal
John Pascal is making a career for himself as a man who can see the cellular response to DNA damage
Advertisement
BY ROBERT PRICE
How do cells cope with DNA damage?
Nobody knows. Yet. But the pieces of the puzzle are waiting to be found.
One person sorting the puzzle pieces—and getting closer to the big picture all the time—is John Pascal, a structural biologist at the Université de Montréal. The inventive work happening in his lab is helping to make the picture crystal clear.
GETTING TO KNOW THE FAMILY
Pascal has been investigating DNA repair for a long time. As a graduate student, he worked in a structural biology lab that used x-ray crystallography to study protein structures. And during his post-doc training, he joined a lab focused on DNA damage repair. There, he studied DNA ligases, enzymes that fix breaks in the structure of DNA. When he set up his own lab, he turned his attention to a family of enzymes called PARP, or poly (ADP-ribose) polymerases.
PARP is involved in virtually every cellular process imaginable. Currently, there’s not a lot known at the level of mechanism and structure—how PARP works and how these enzymes repair DNA. What is known is that some members of the PARP family are heavily involved in the DNA damage response by detecting DNA damage and making sure it’s repaired in a reasonable timeframe.
In PARP, Pascal found a fascinating structural puzzle: when PARP detects DNA damage, they become catalytically activated, but how they become activated and how they detect damage wasn’t understood.
“PARP enzymes are probably the fastest proteins among those that detect DNA breaks,” he explains. “So, ligases were acting at the end of the pathway when damage is being repaired, and the PARPs were sort of at the beginning of the pathway. This really caught my attention.”
Beyond the fascinating structural puzzle waiting to be solved, PARP offers huge potential in delivering something new to researchers, in particular in the area of inhibitor design.
“And there’s been a lot of interest, not just from people like me who enjoy understanding mechanism structural biology, but also the medical community,” says Pascal. “They perceive greater insights into these enzymes that they’re targeting with drugs.”
ZOOMING IN
PARP is good at fixing things. The PARP enzyme known as PARP-1, in particular, is very good at binding DNA breaks. When it encounters a DNA break, it binds to the break and produces poly (ADP-ribose), a chemical polymer that’s synthesized at the site of the DNA break. This polymer highlights where DNA damage is in the cell. It’s an alarm screaming for help.
What Pascal finds interesting is the way the enzymes work. Prior to binding to DNA breaks, PARP-1 is essentially inactive. But when it binds to DNA breaks, it becomes activated a thousandfold to make poly (ADP-ribose).
How it happens, until now, might have been best described as magic.
“We were really curious from a sort of mechanism point of view. How does PARP-1 bind DNA breaks, and how does that get communicated to another part of the enzyme? Because it’s a pretty big protein.”
The other intriguing problem was the structure of the activity. Most of the DNA binding happens on one end of the DNA, while the catalytic activity happens on the opposite end. As Pascal explains, there has to be some kind of communication between those two events. Magic can’t be the answer.
It wasn’t until Pascal and his team got a good look at the activity when researchers finally began to see what happens.
FINDING THE GOVERNOR
Over the years, Pascal’s lab had shown how PARP recognizes breaks and highlighted the pathway of communication between the DNA binding and the synthesis of this poly (ADP-ribose).
Pascal’s research team knew PARP-1 underwent conformational changes when it activated, but they hadn’t been able to isolate it in the active stage—the stage when it’s ready to make poly (ADP-ribose).
The key finding came when Pascal and his team discovered the helical domain, the dynamic movement that allows these enzymes to do their work to repair broken DNA.
The helical domain is, as Pascal describes it, “the governor of the active site.” In the off-state, the helical domain blocks the active site and prevents the enzyme from accessing any of the precursor nicotinamide adenine dinucleotide (NAD) it needs to make poly (ADP-ribose). When PARP-1 becomes activated—when alarm bells ring—the helical domain distorts, allowing access to NAD.
Getting to that stage—like so many breakthroughs—took some creative engineering to capture that active state of the PARP-1 enzyme. Up until this point, the structures were crystalized with PARP-1 in the inactive state; the active state was a mystery and difficult to image. PARP-1 is a large protein, and the bigger the protein and the more flexible it is, the harder it is to crystalize often.
Pascal’s solution was to chop up a mutant of PARP-1 and reconstitute the most stable parts.
“We don’t have the full protein. We only have what we can classify as the important parts, and we mix those together.”
It isn’t easy to chop up a protein and reconstitute the activity of that protein. But it worked with PARP-1.
Using x-ray crystallography, Pascal crystalized the chemicals, shot these crystals with x-rays to determine the electron density map of the chemicals, and built an atomic model to fit that electronic density map of PARP-1 while active. Essentially, he took a photo of the protein in action. And what he discovered was that a large conformational change occurs with PARP-1, with the active site having a large rotation. This rotation within the protein allows a new chemical group to enter the vicinity of the inhibitor binding site.
This understanding changes the landscape of what designer inhibitors can look like.
“Why it’s meaningful is that previous structures hadn’t really seen what the active site looked like when it’s fully active, the state you would want to inhibit,” says Pascal. “This would give them a different sort of viewpoint of how to modify inhibitors to maximize interaction.”
And as it turns out, a lot of the newer inhibitors are being designed for PARP-1 in a way that prevents them from hitting other PARP family members. These inhibitors will be able to take advantage of the helical domain because it’s unique to PARP-1, a development made possible by Pascal’s ability to image the helical movements of the active PARP-1.
John Pascal—Professor with the Department of Biochemistry Doctoral candidate Élise Rouleau-Turcotte
REASONS FOR WORKING
While many chase the big discoveries—curing cancer or sending people to Mars—Pascal relishes the close study of nature’s intricate machinery.
“For me, it’s always been the basic mechanism of PARP-1,” he says. “At the chemical level, how is this thing operating?”
A North Carolinian who now works and lives in Montreal, and who started his education as an engineer, Pascal says he’s always enjoyed thinking about mechanics and how things work. The x-ray crystallography/biology work he does at Université de Montréal is a good mix of wet lab biochemistry and draws heavily on Pascal’s knowledge of computation. He says the work fits his interests and talents, and the fact that he contributes incremental knowledge to a larger project—uncovers another piece of the puzzle—is a bonus.
“It’s been rewarding that that’s been helpful, or instructive for a better understanding of inhibitors. But the driving force for me has always been the basic mechanism, and just knowledge at that level has always been appealing to me.”