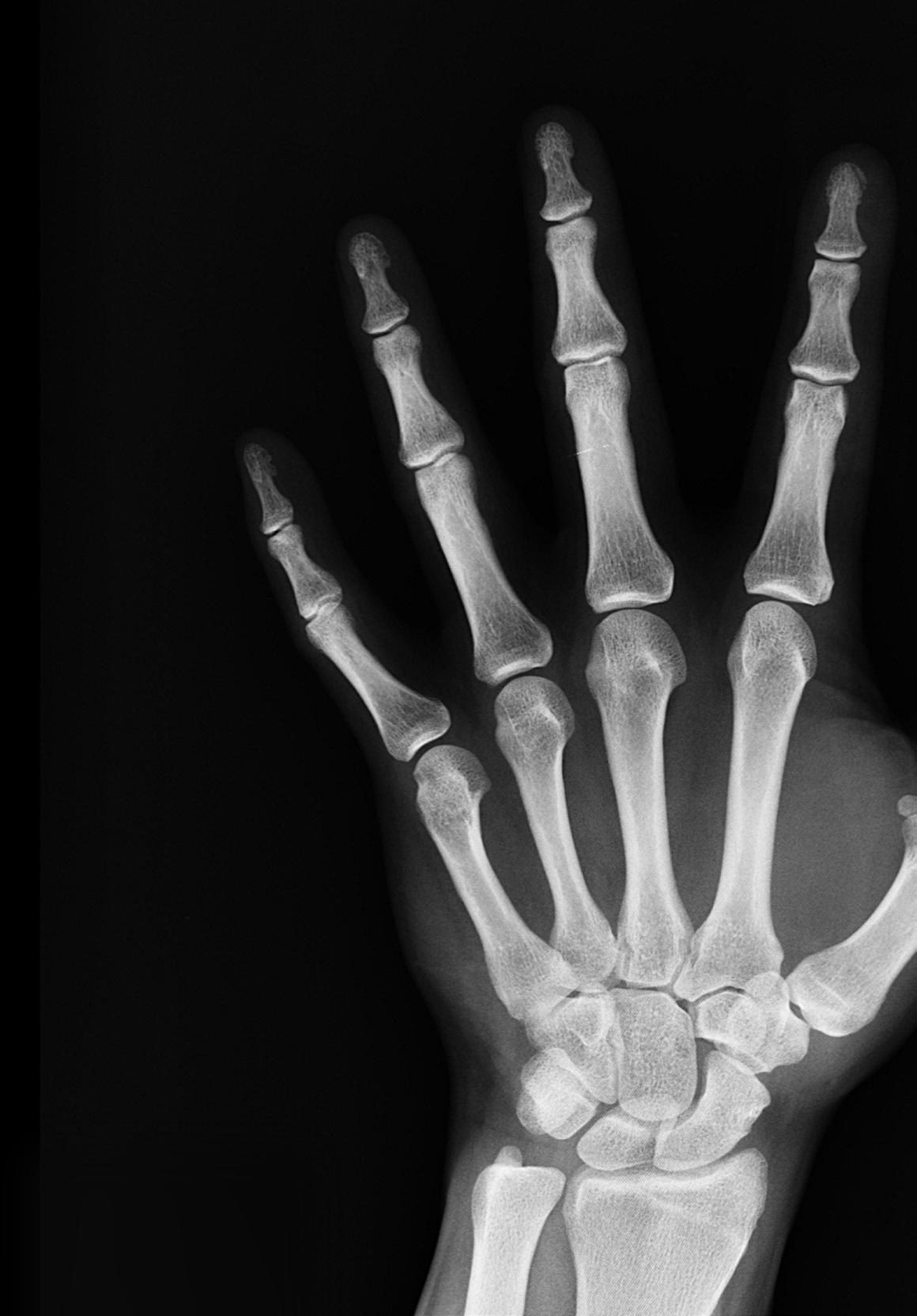
9 minute read
SYNCHROTRON RADIATION Sayuri (LVI
SYNCHROTRON RADIATION
Sayuri, LVI
In our life, we are supported by a wide variety of things from vehicles to oxygen that we inhale to make our life comfortable. All these matters are made of nano-scale particles that we cannot see. However, to develop our knowledge and solve problems that we still face, whether it is environmental impacts or social impacts, researchers are always seeking a new solution and discovery.
During my summer holiday, I had a wonderful opportunity to visit one of the most famous synchrotron radiation facilities, Spring-8 in Japan. Synchrotron radiation facility is one of the crucial scientific facilities for all sorts of research and experiments. It offers noticeable advantages to expand our vision. So, what is synchrotron radiation and why are synchrotron radiation facilities important?
In the simplest form, at a synchrotron radiation facility a very intense X-ray, also known as synchrotron radiation and synchrotron light, is produced to penetrate materials and reveal the inner structure of matter down to the level of atoms.
The most famous synchrotron radiation facilities, ESRF (shared with European countries, located in France), SPS (USA), and Spring-8 (Japan) are the only ones that the electrons reach above 6GeV. These facilities are often referred to as ‘hard or large-scale X-ray third-generation synchrotron radiation facilities’. In Spring-8, which was founded last out of three, the electrons are accelerated up to nearly the speed of light (3 x 10^8) with a maximum of 8 giga electron volt on electrons which is the highest in the whole world now. Therefore, it is named Spring- ‘8’. Anything between 1 and 5 GeV is classified as a 3GeV-third generation synchrotron radiation facility. This includes Diamond Light Source situated in Rutherford, UK.
The main characteristic of the X-ray produced at a synchrotron radiation facility is that it is very high in brilliance. Brilliance is a term used to describe how bright and angular the spread of the beam is. As the X-ray is bright and has great precision in diffraction, scientists can detect them clearly and it is easy to record them and to analyse the material being tested. This X-ray has more amazing characteristics which attract scientists in the study of various fields. For instance, having high energy permits the beam to penetrate deeper into the matter, and having a small wavelength can take us into the world of the nanoscale. Fun fact, the X-rays produced in ESRF are 10,000,000,000,000 times brighter than the X-ray that is used to take a picture of our bones in the hospital!
To produce this incredibly useful X-ray, electrons are accelerated and diffracted. In any facility, the process begins by emitting electrons out of an element. If you take Physics A Level, you might be familiar with this first step, electron gun. By heating a wire of an element, thermionic emission happens which is when the electrons in the element wire fire themselves out as they gain kinetic energy. The high potential difference is applied between the element wire and anode which turns the element into the cathode. Freed electrons accelerate towards the anode by gaining more kinetic energy which becomes a beam.
These electrons are sent to the next section of the facility, the Linear Accelerator (Linak)/Acceleration Tube. Here, the electrons are gradually accelerated using electric fields until they become close to the light speed. In the next section, athletics track shaped Booster Synchrotron adds extra energy to the electrons and accelerates to the final speed before they are sent to the storage ring. As electrons must bend to travel through this section, dipole bending magnets are used.
Finally, the storage ring. If you search up Synchrotron Radiation Facility, this is the section you often see. Here, the electrons that are close to the speed of light travel around and around until they lose their energy. To prevent electrons from hitting the wall of the tube, losing all their energy and dropping their speed down, the tube is under ultra-high vacuum conditions (around 10^-9 mbar). As they travel through the ring, the electrons are diffracted due to going through different types of magnets. As they are diffracted, they lose energy in the form of electromagnetic radiation known as ‘synchrotron radiation’ and emit the X-ray for good use. A few examples of magnets used are bending magnets, undulators and focusing magnets. Bending magnets simply force electrons to change direction. This leads to electrons emitting synchrotron light tangentially to the curved path of the electrons. The undulators are a collection of a series of small magnets with alternated polarity. Due to this, electrons are diffracted multiple times, producing a million times more intense and bright X-rays which are close to laser, compared to X-rays produced by electrons travelling through bending magnets. The focusing magnets make the electron beam as narrow as possible which could be as narrow as 1 micrometre (0.001mm).
Now that electrons are in the condition that they can produce synchrotron light, how does this reach each scientist for them to use? When the X-ray is emitted tangentially to the storage ring, it directly travels towards the individual beamlines where each group of scientists have their own space. In Spring-8, there are 62 beamlines around the storage ring with a circumference of 1435.95m. Most of these individual beamlines are used by RIKEN; Japan’s largest research institution which is in charge of the whole Spring-8, industries such as automotive companies (e.g. Toyota, Nissan) and electronic companies (e.g. Sony, Panasonic, Mitsubishi Electric), universities for professors and students to research, and also companies and groups of researchers from Taiwan as they have banned from using large-scale Synchrotron Radiation Facilities). At the end of the beamlines, there is a special machine suitable for the specific research, where the scientists can place the material or substance to be analysed. Most of the machines have a similar feature, for example, the sample to be tested would sit in the middle of the machine and the part of the machine which detects the X-ray that was been diffracted or spread can rotate around the sample. As the X-ray that travels through the beamline is very narrow, the sample must sit in a specific place and stay there so that it does not miss the X-ray. These machines require skilled experts for each research asked by the researchers. I am sure it is not surprising that there are only a few companies that deal with these machines in synchrotron radiation facilities, for instance, most machines in Spring-8 are from a company in Germany that specifically create and fix machinery for synchrotron radiation. On the other hand, it is astonishing that such a familiar substance, aluminium foil takes an important role in lots of experiments. In experiments that require very precise temperature, aluminium foil maintains the temperature of the beamlines and other machines by wrapping aluminium foil around them tightly so that the temperature stays constant.
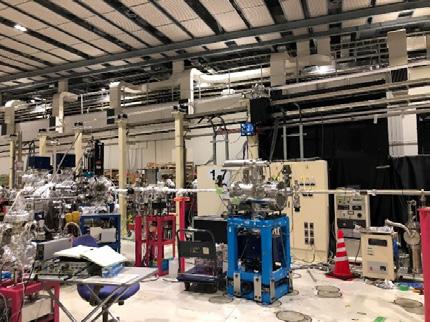
On the picture above, you can see the thin tube going through various machines. This is the beamline where the X-ray passes through. On the left end of the picture, it is not clear, however, the shiny grey object is where the machines are wrapped with aluminium foil.
On the way to Spring-8, I pass through a highway that is famous for being the most financial deficit. I wondered why Spring-8 was situated in Sayo district in Hyogo prefecture which was isolated from large cities. To build the facility, it requires a large space to accelerate the electrons to an enormous speed and to keep it in a circular tube to send X-rays off to individual beamlines. However, I also found out that due to safety reasons, it also requires stable ground. In the case for Spring-8, Sayo district was chosen as it is an upland area with stiff soil and has a low chance of liquifying. From these factors, it is predicted that there is only a 30% risk of experiencing magnitude 5 and a 0% risk of experiencing magnitude above 6. According to the professor who has worked at the Spring-8, Hyogo prefecture offered the land to hand it over to RIKEN for free which was a big factor as even if it is in the countryside, the cost will be enormous with the large area to be dealt and to construct the whole system.
Indeed, the facility itself, including beamlines and lots of different types of machinery around the beamlines to gain scientific proof for research is hugely fascinating. I also found it incredibly amazing that all sorts of different fields of research, from aerospace to bio-research, are using synchrotron radiation facilities.
One of the popular research projects in the field of life science is analysing protein structure in humans. It is said that a human is made from roughly 6x10^13 cells. Most of these cells are still unfamiliar to us, and to find out what each cell does we need to analyse the DNA inside and the structure of the protein. By using this specific X-ray, it allows scientists to gain a clear image of this nano-sized structure of proteins which is impossible in other facilities. These specific structures of proteins permit us to easily find what disease the person has and the ability for researchers to do further research to treat and cure the illness including creating new medicines. Another research that is ongoing, which I think is very relevant and important for the future generation, is on the porous medium. The porous medium is a material containing pores and scientists in the field of particle physics believe that finding out their specific nano-scale structure, can lead to creating new materials to tackle environmental issues, such as carbon dioxide and methane gas. If the research is successful, it is predicted that the new materials can selectively decompose greenhouse gases, remove them, or even store them and convert them into new materials.
I have mentioned two examples out of millions of research that took place or is taking place at the moment, but here are some other achievements from the past. A thin layer at the bottom of the mantle, which was not known what the compounds were, was finally identified (earth science). Similarly, in industrial research, a new catalyst that is more efficient in cleaning exhaust fumes that the cars produce was discovered and the reason for it became clear. The conventional catalyst decreased in their performance as the distance the car travels increased, however the new catalyst, ‘intelligent catalyst’ regenerates itself continuously. This is due to the electrons of the metal in the catalyst. In the previously used catalyst, the electrons of metals repetitively exit and enter the metal causing neighbouring metals to combine whereas intelligent catalysts can maintain their original state. The graph below represents the change of performance in purifying exhaust fumes by the catalysts.
From all the past discoveries and more researchers using synchrotron radiation facilities, I strongly believe that it has a very promising and optimistic future use to improve our knowledge and make the planet a better place for us. Even if you are not interested in physics, research that takes place at the synchrotron radiation facility does not necessarily relate to physics, for example, I have mentioned research in the field of biology and geography/geology. As expressed, the synchrotron radiation facility is a hot topic in STEM and should be discussed further.
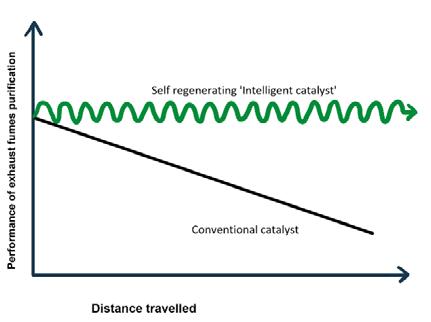