
4 minute read
THE HEART OF AN EXPLORER
In 2018, a team of NASA astronomers shared a photo of Icarus, an individual star 9 billion light-years from Earth.
It was the most distant star ever seen, and it was the Hubble telescope that enabled its discovery.
Advertisement
To a structural biologist, the body is its own universe. Within each cell, each uncharacterized protein, peptide, enzyme or lipid represents an undiscovered new world no less awesome than the stars and planets.
Like astronauts or explorers of old, structural biologists seek out unexplored terrain. If the great Greek cartographer Ptolemy had lived today, he might have chosen structural biology for his life’s quest. Microscopes, rather than telescopes or ships, open these new worlds to modern-day explorers of inner space.
A new world
Viewing a protein’s structure for the first time—seeing its arrangement of atoms, how it folds into origami-like shapes and how those shapes dictate the protein’s role in the living organism—that’s the thrill of discovery that motivates Florida-based biologist Tina Izard, PhD.
“The first time I saw a three-dimensional structure, it just took my breath away,” Izard says.
Using a specialized technique called cryo-electron microscopy, Izard recently enjoyed yet another first, seeing the structure of a protein critical to a healthy heart and implicated in heart aging and stiffness. Known as metavinculin, the protein tethers the cardiac cell’s membrane to the matrix within.
Heart Wall
3 MILLIMETERS 3 MILLIMETERS
SMALL: The wall of the right ventricle is about 3 mm thick.

Heart Cells
10-25 MICROMETERS
SMALLER: A cardiomyocyte has a cylindrical shape and measures about 100 micrometers long by about 10 to 25 micrometers in diameter. A micrometer is 1/1000th of a millimeter. The green dots at the tips of the heart cells represent the location of metavinculin proteins.
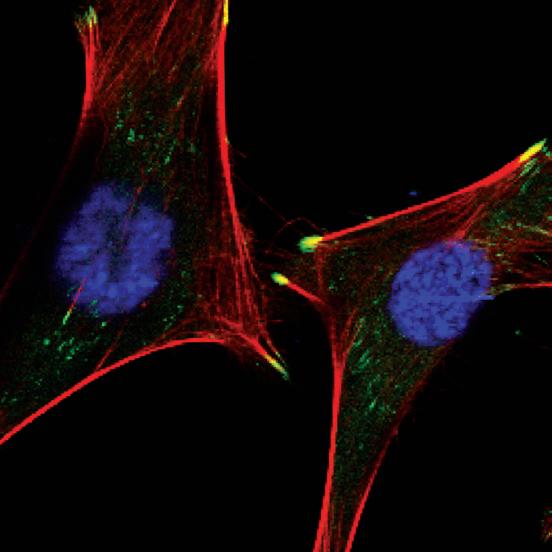
Heart Proteins Metavinculin
100 ANGSTROMS
SMALLEST: The metavinculin protein structure is seen in a cryo-electron microscope image for the first time, folded as it appears in the actual heart cell. It is about 100 angstroms long. An angstrom is 1/10,000th of a micrometer. This protein connects to a group of other proteins, which includes actin, talin and integrin. They all work together to give the heart cell its structure.
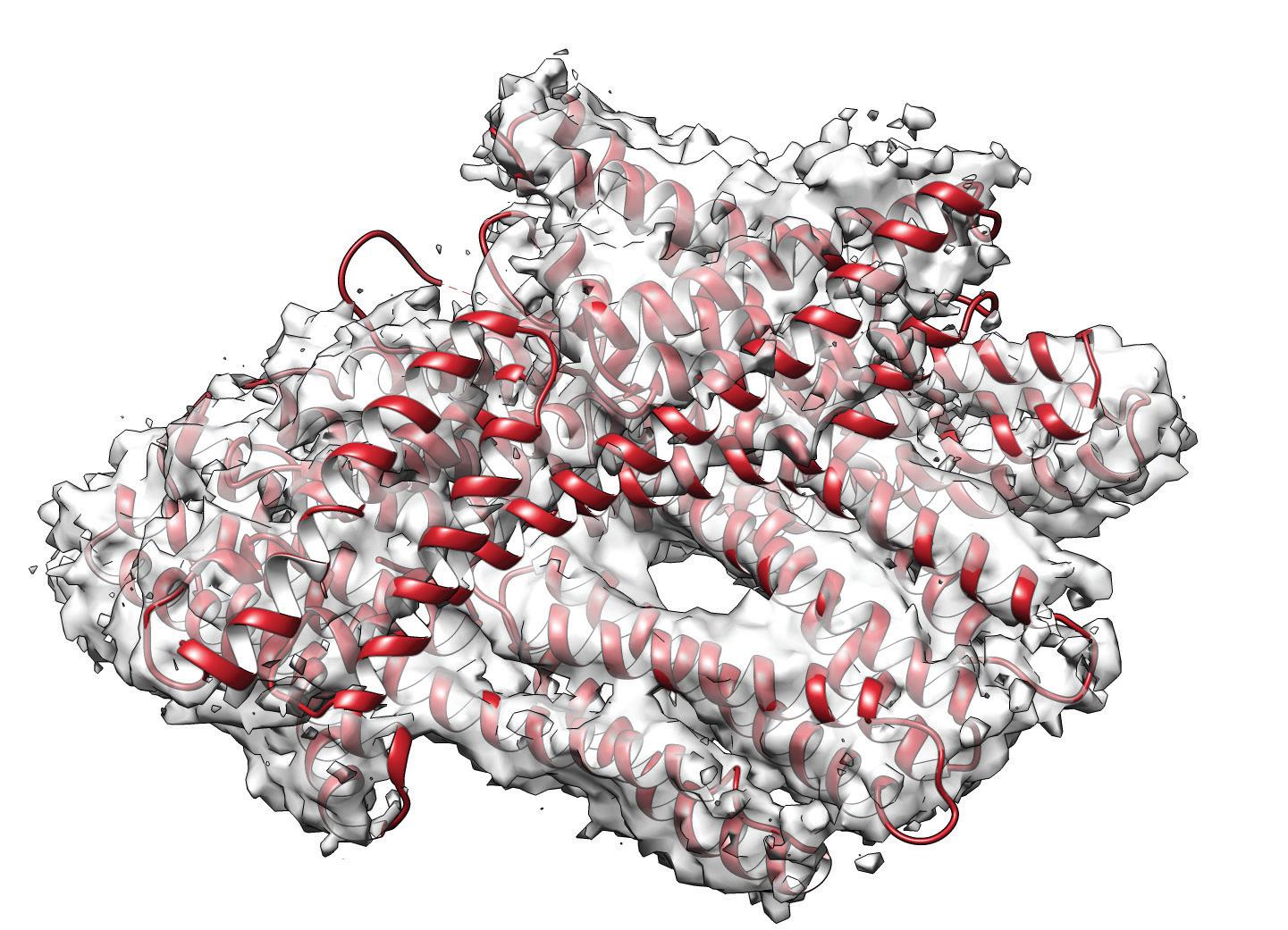
She saw a bundle of four helices, a shape that allows for tightening and loosening, like a cluster of woven finger-trap toys.
“I can’t even describe it. It’s just like, ‘Oh my gosh, it worked! Oh my gosh, we got the structure!” Izard says. “It’s a motif that you see in a lot of proteins, the four-helix bundle.”
The tool Izard used to see metavinculin was a very special microscope, one that involves freezing samples instantly down to hundreds of degrees below zero. It takes thousands of images, like a CT scanner, and then stitches them together to reveal a three-dimensional structure. The cryo-electron microscope model Izard used is being installed at Scripps Research in Florida later this year. On a mission to Japan to learn how to use the new equipment, Izard brought along her metavinculin protein samples in the hopes of seeing its structure.
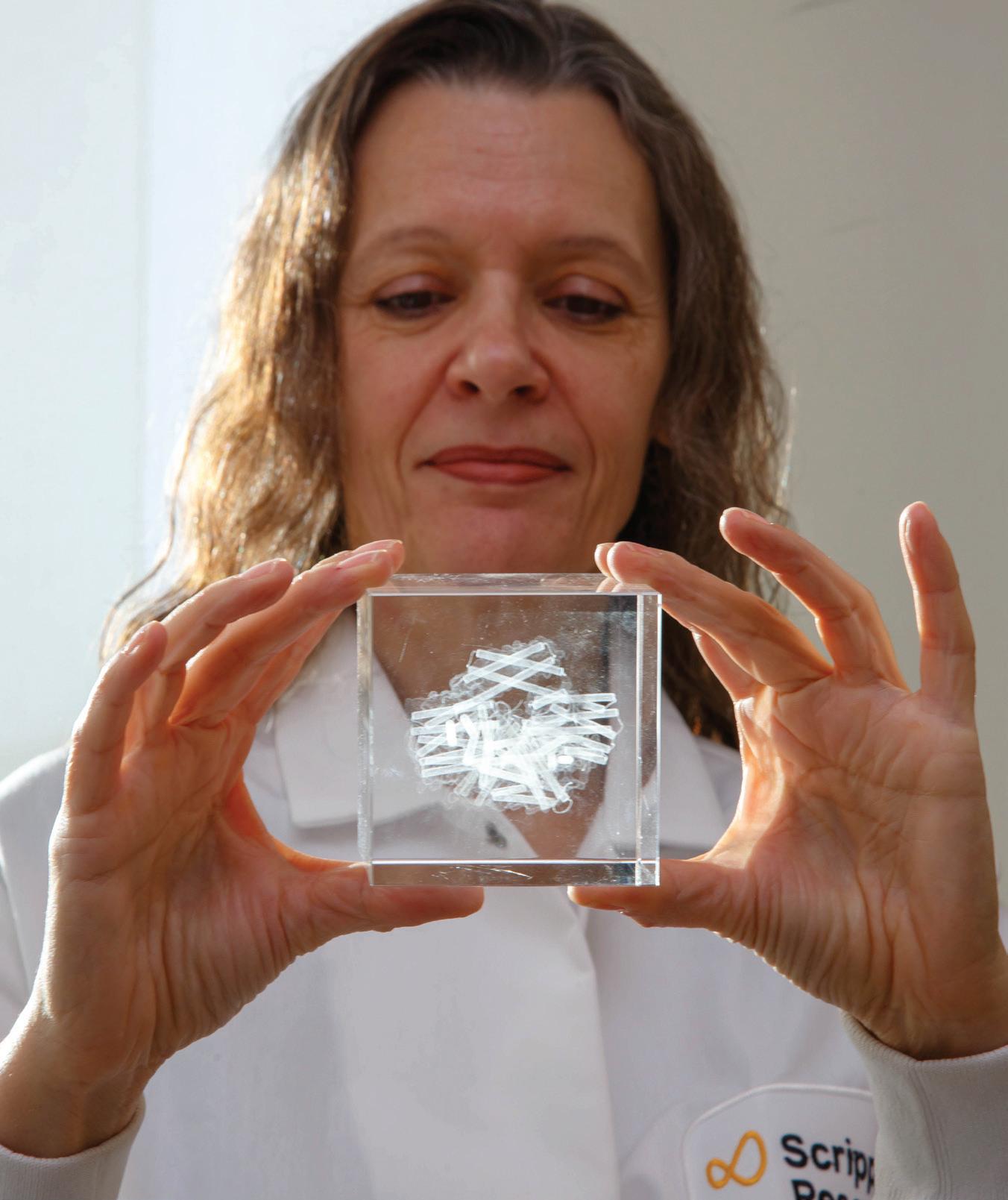
Structural biologist Tina Izard, PhD, holds a crystal cube etched with a "ribbon" model depicting vinculin. Vinculin is a protein that enables cells to adhere to one another.
To see a protein like vinculin, one must go small—much smaller than a traditional light microscope can see.
A human hair is about 29,000 nanometers wide. A red blood cell is about one-fifth that width, around 6,000 nanometers. Light microscopes, at their limits, enable us to see things the size of a bacterium, as small as about 200 nanometers.
The protein that Izard studies is about 16 times smaller than that. A light microscope won’t do the job. Standard transmission electron microscopes can visualize static things that small, but they destroy biological molecules in the process.
Cryo-electron microscopy has made visualization of these tiny biomolecules possible.
“Time on a cryo-electron microscope is very precious, so I said, ‘Let’s do a real protein,’” Izard recalls. “They collected data in two days, and suddenly it pops out, and I can’t even describe it. It doesn’t happen that often that you put the first one in and there it is. It was quite a historic moment.”
Life in motion
Izard is fascinated by the vinculin proteins because of their many roles in living cells. In the beating heart cell, metavinculin connects to the actin cytoskeleton. Actin is a protein filament that expands and contracts with the moving heart cell. Studies suggest that excess accumulation of metavinculin may occur with age and may be to blame for symptoms such as cardiac stiffening and diastolic dysfunction, which affects how the heart fills with blood.
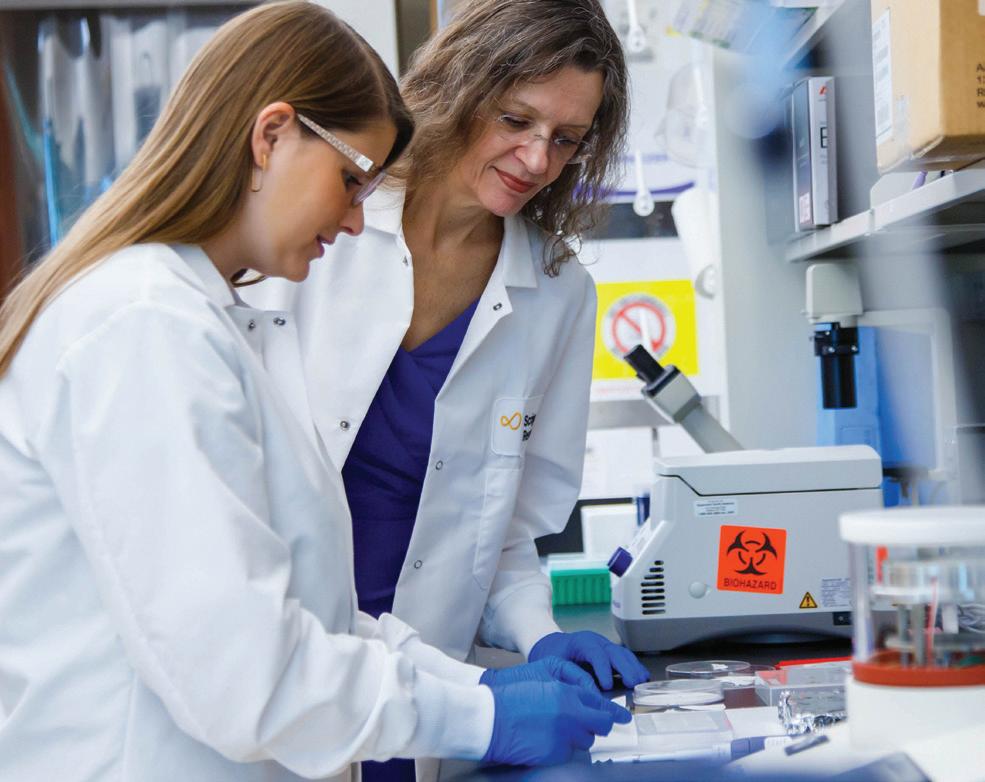
Izard and her research associate, Marino Candido Primi, PhD, demonstrate sample preparation.
Because the vinculin protein also anchors cells together, if it is removed, the cells disperse. That’s why it’s important in the spread of cancer.
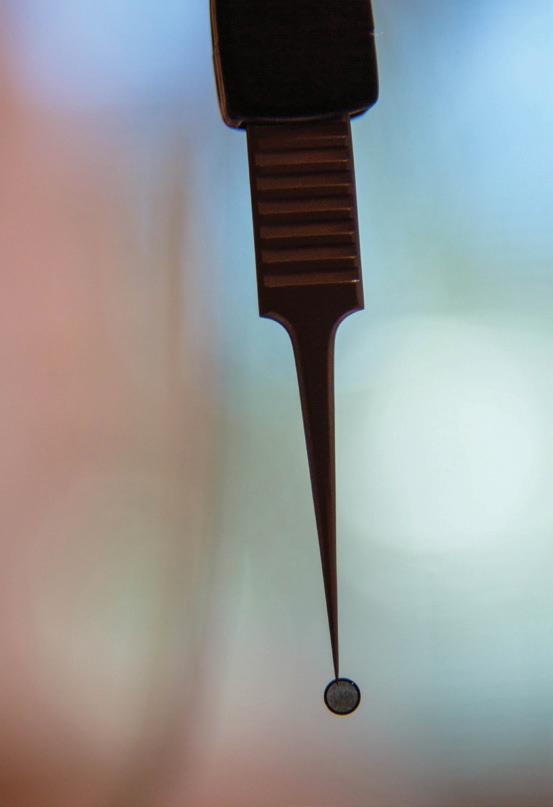
Examining critical biomolecules like vinculin through cryo-electron microscopy involves several steps. First, a sample of the biomolecule must be made. For this, a screenlike grid so tiny that it requires forceps to hold is coated in a film of the sample. Next, it is transferred to a device called a plunge freezer.
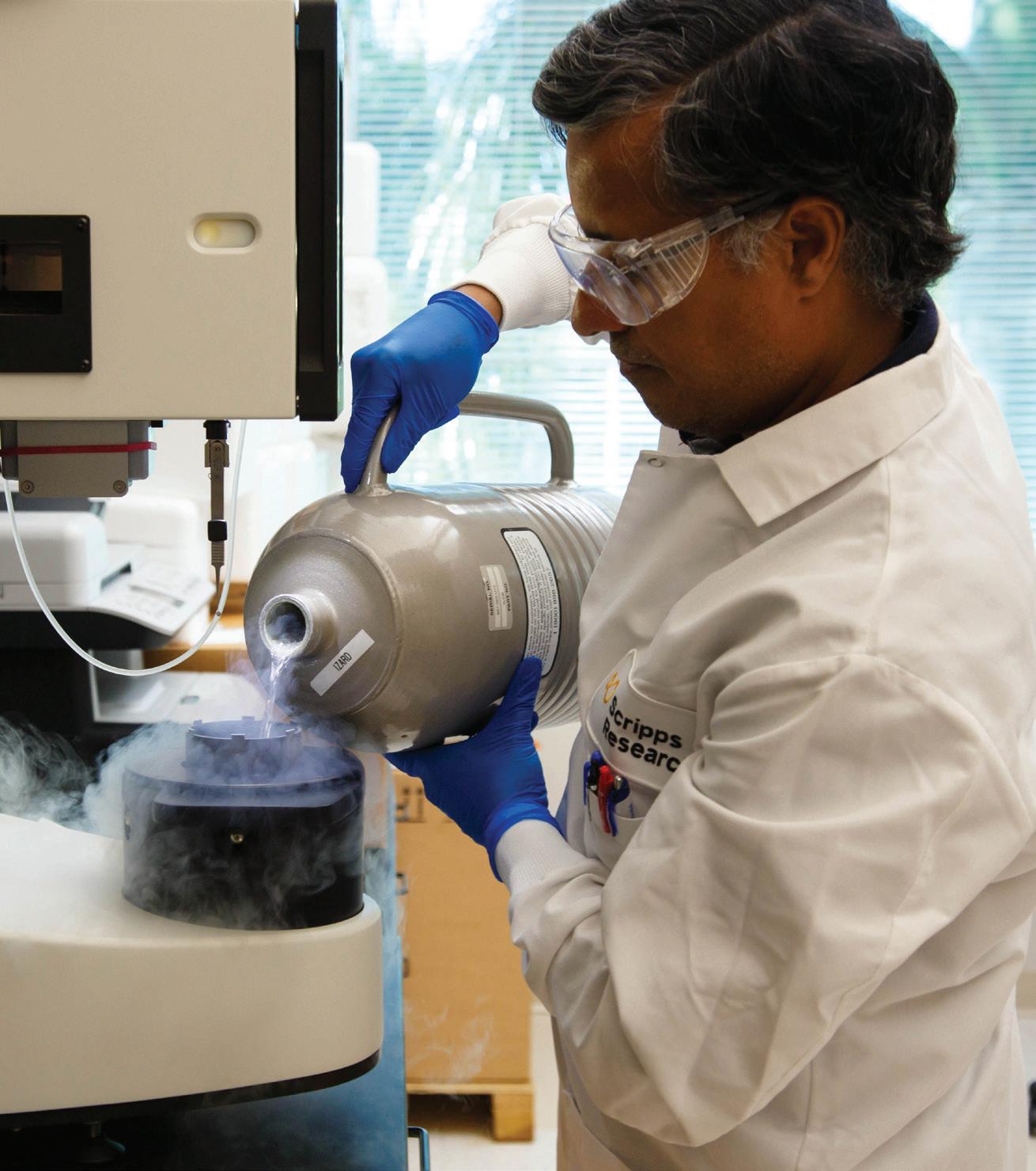
Various cryogen solutions are used to freeze the sample, including liquid nitrogen and liquid ethane. They cool the sample to hundreds of degrees below zero. In a state of suspended animation, the molecule can be photographed thousands of times with the digital cryo-electron microscope. The digital images are then stitched together with software to reveal a three-dimensional picture.
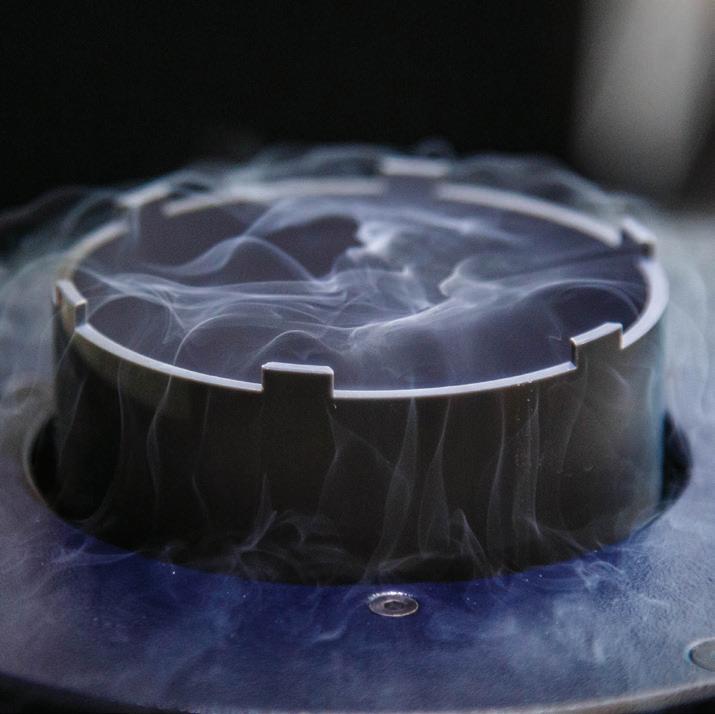
Because form dictates function, important insights about health and disease often result from these images. For molecules at the root of disease processes, this visual information can play a central role in helping scientists develop potential therapies or vaccines. Once prepared, the grid is moved to the plunge freezer. In these images, Izard’s research associate, Rangarajan Erumbi, PhD, prepares the plunge freezer with liquid nitrogen.
Beyond the thrill of seeing something new, understanding the precise structure of a biomolecule associated with a disease presents chemists with an opportunity to design therapies to intervene, Izard explains. Vinculin isn’t only important in heart disease, she adds.
A slightly different form of vinculin plays a critical role in binding cells to one another, like adhesive tape. In this context, vinculin can change shape to help generate cellular movement. This becomes a key issue in enabling cancer cells to spread. Because the vinculin protein also anchors cells together, if it is removed, “the cells just go everywhere,” Izard says.