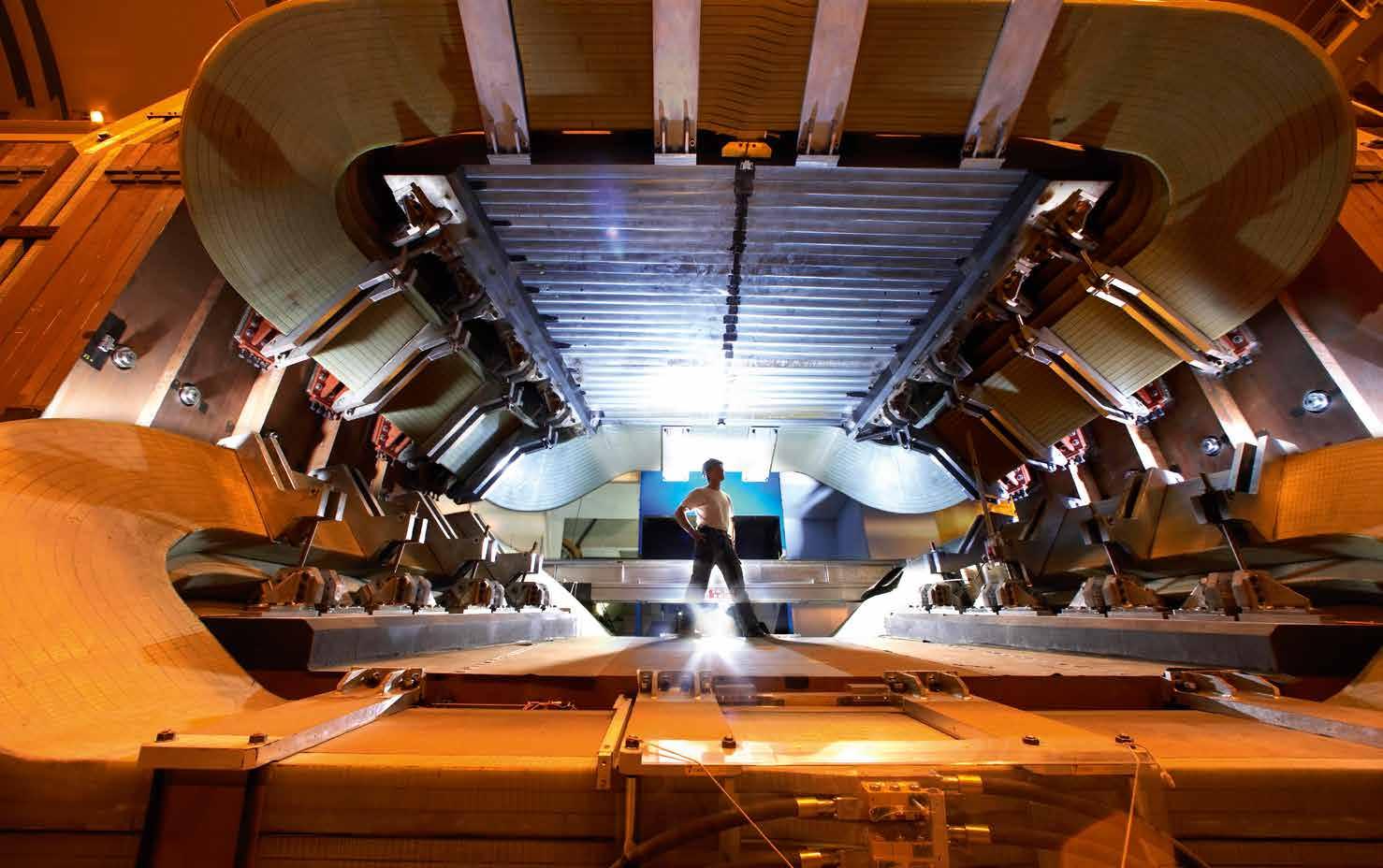
8 minute read
RECEPT
© 2008, Courtesy of CERN
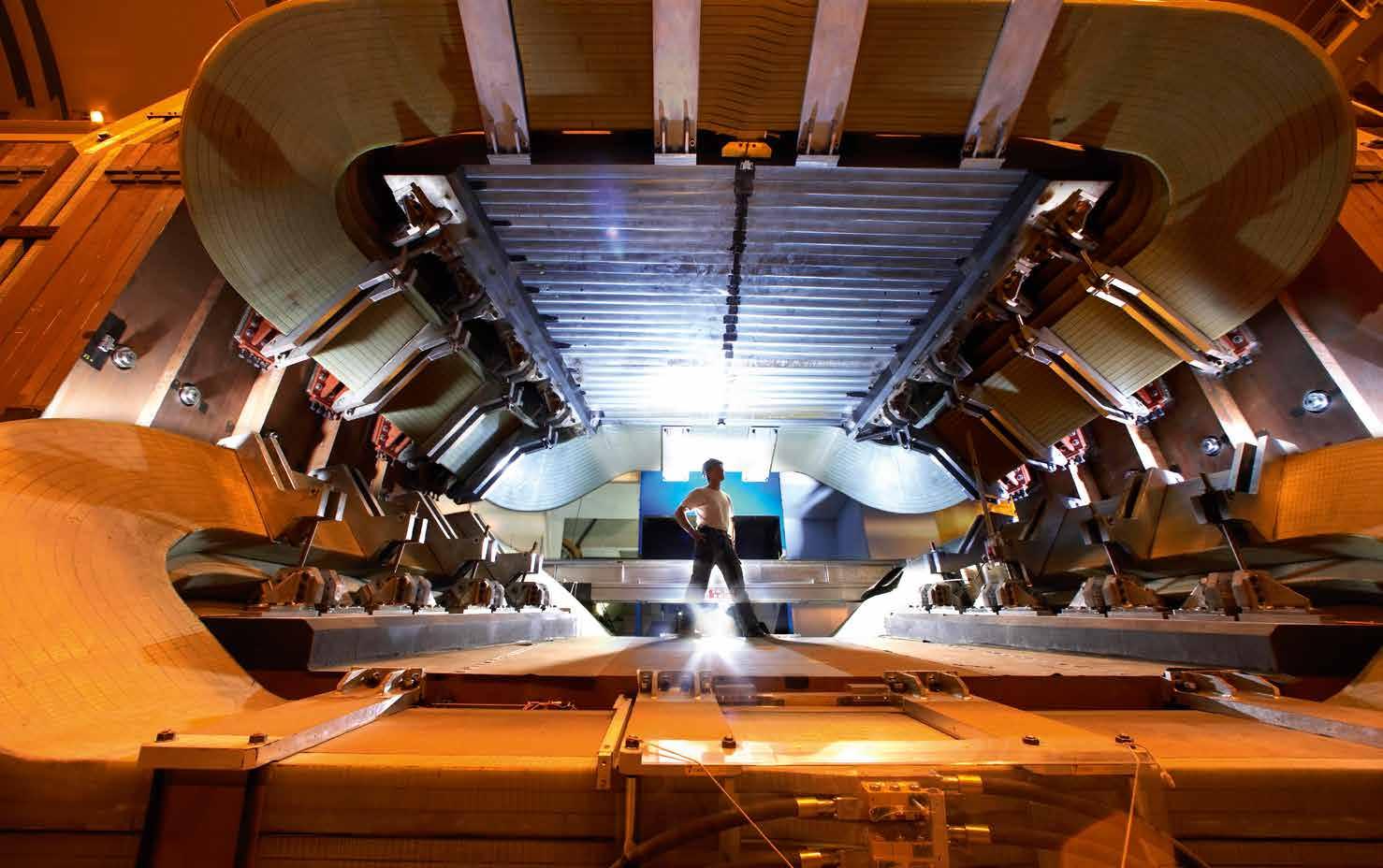
A window into new physics
The standard model of particle physics is extremely successful in describing nature on microscopic scales, yet it is not completely compatible with theories of the macroscopic universe. Researchers in the Recept project are probing the possibility of new physics beyond the standard model by testing a prediction called lepton universality, as Dr Vladimir Gligorov explains.
The standard model of particle physics is extremely successful at describing nature on microscopic scales, in the sense that it predicts the properties of a wide range of particles. A contradiction emerges when researchers attempt to reconcile the behaviour of these particles as described by the standard model with theories of the macroscopic universe, such as the Big Bang. “There are numerous difficulties, one of which is the problem of dark matter and dark energy. Essentially, on macroscopic cosmological scales, there is a mis-match between the quantity of visible matter and the mass of matter that you would infer from how the galaxies move around. This is why people hypothesise the existence of dark matter, that could exist outside the standard model,” says Dr Vladimir Gligorov. Based at Cern in Geneva, where the Large Hadron Collider (LHC) is located, Dr Gligorov is the Principal Investigator of the Recept project, a collaborative international initiative aiming to test the predictions of the standard model, which could lead to new insights into fundamental physics questions. “Are there particles or forces beyond the standard model which resolves the early universe?” he asks.
RECEPT project This research involves monitoring the behaviour of particles, using data from the LHCb experiment at Cern, and then looking for evidence of new physics. Despite being based at Cern, LHCb is very much a collaborative initiative, bringing together researchers from 16 countries. The data is generated at the that have been described, and while it can be difficult to produce these particles in the laboratory, Dr Gligorov says it is still possible to investigate them indirectly. “Even if new particles and forces are too heavy to be produced directly in the laboratory, they could interact virtually with the existing particles that you do produce,” he explains.
LHC then distributed across the globe via the worldwide computing grid, essentially a network of data centres in different countries, giving researchers across the world access to important data. “It’s very democractic - everybody involved in the collaboration has equal access to all this data,” says Dr Gligorov. A number of new particles and forces have been postulated to explain the inconsistencies Researchers in the project are using this kind of approach to test a specific prediction of the standard model called lepton universality. “This is very interesting because it’s something that the standard model predicts very precisely,” says Dr Gligorov. “The standard model states that there are six leptons, three of which are charged leptons – the electron, the muon and the tau. There are also three
associated neutral leptons, or neutrinos, one for each type of charged lepton.”
The standard model predicts that beauty mesons should couple in the same way to electrons, muons and taus, yet some of the evidence gathered from the LHCb experiment has hinted at a different picture. By probing these differences, researchers hope to gain deeper insights into the possibility of new physics beyond the standard model. “If you observe non-standard behaviour then there might be some new particle which is causing this deviation. From the size of this deviation, we can then try to learn something about what kind of new particle is causing it,” explains Dr Gligorov. Researchers in the project are analysing the decay processes of certain particles and looking for evidence of new physics, for example if beauty mesons couple preferentially to an electron, muon or tau. “When we measure lepton universality we rely on collecting huge numbers of abundantly produced particles, and observing their rare leptonic decays which might reveal this universality breaking at high levels of precision.” explains Dr Gligorov.
A large volume of data has been generated at the LHCb experiment, representing a vast source of information for Dr Gligorov and his colleagues to analyse. While some observers had expected that the LHC would reveal the existence of entirely new particles when it was first turned on in 2010, this has in fact not been the case, which Dr Gligorov says illustrates the importance of indirect searches in the investigation of new physics. “If these particles are too heavy to be produced directly in the LHC, then maybe we can indirectly seek evidence of their existence,” he says. This work also holds
Data processing This could help researchers to probe a number of major open questions in physics in even greater detail, including those around testing lepton universality. A very wide range of measurements can be made to probe lepton universality, and Dr Gligorov says there is scope to improve the way these measurements are gathered and stored. “For that, we need to upgrade
relevance in terms of the design of the next generation of particle colliders, which is an important aspect of the project’s research. “Cern has recently proposed building a new collider called the future circular collider,” outlines Dr Gligorov. “The existing LHC has a circumference of 27 kilometres. The future circular collider would be 100 kilometres in circumference and operate at far higher energy levels.” the experiments, to collect a lot more data. In order to collect more data, we have to upgrade our data processing capabilities,” he says. Dr Gligorov and his colleagues are heavily involved in this work. “The aim is to achieve the capability to process 40 terabits of data each second. The LHC collides for approximately 6 x 10 6 seconds per year, so this data must be reduced to around 80 gigabits per second in order to fit
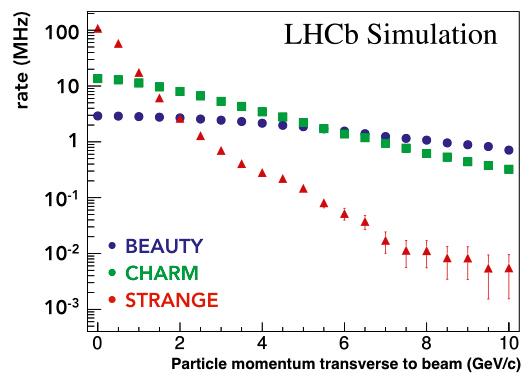
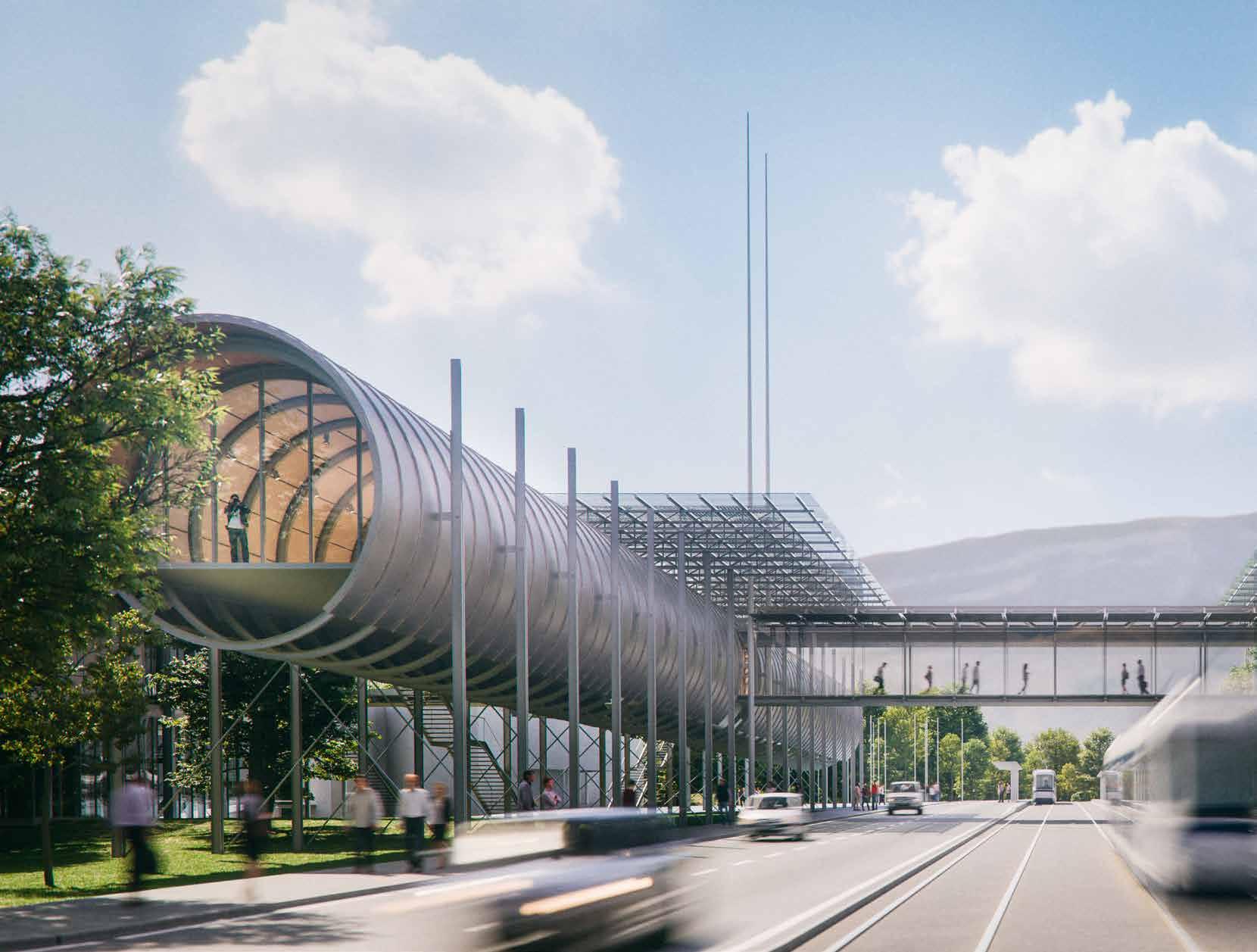
Expected rate of different signal particles produced in a future upgrade of the LHCb detector, plotted against the transverse momentum of the particle.
within the available storage resources. For that, you essentially have to build a custom data centre, on a relatively small budget,” he continues.
This has been the focus of a lot of attention in the Recept project. “We’ve been working to develop new algorithms, including on some new architectures. Until now LHCb has used CPUs for its real-time processing, but we’ve also developed a way that allows part of this work to be performed on GPU processors – which are potentially more cost-effective,” outlines Dr Gligorov.
This ‘Allen’ framework developed by the Recept team is able to process around 80 gigabits of data per second on a single GPU, and make decisions which parts of that data should be kept. Identifying the important information more rapidly means Dr Gligorov and his colleagues can then devote more of their attention to the most interesting data. “The more you can automate, the more you save your energy for the things that matter,” he stresses. “LHCb’s real-time algorithms allow us to infer not only whether an LHC collision looked interesting but also which parts of this collision are most relevant for further analysis.”
The Recept team is currently waiting to learn if the LHCb collaboration will decide to use Allen for its data processing, but Dr Gligorov stresses that whichever option is chosen the lessons learned from developing a GPU solution will benefit the team and collaboration in the long term.
A successful real-time analysis provides the foundation on which researchers can look to test lepton universality. Researchers are currently in the process of finalising two pieces of analysis on lepton universality, one on rare decay and another on semileptonic decay. “They will help to increase the precision with which we are able to look at lepton universality in different channels,” says Dr Gligorov. Alongside research on lepton universality, Dr Gligorov and his colleagues are also looking forward towards future further upgrades of the LHCb detector. “Looking further ahead, we still plan to gather much more data and to probe lepton universality at higher levels of precision,” he says. “These kinds of experiments run over long timescales, and future upgrades of the detector are already being planned. They will have even bigger data volumes and we are investing a lot of effort in developing our skills in real-time processing on different architectures so we can best tackle those challenges.”
Renzo Piano Building Workshop © 2019 CERN
RECEPT Real-timE preCision tEsts of lePton universaliTy Project Objectives The Standard Model (SM) of particle physics is a remarkably successful description of microscopic nature. Nevertheless, it is in contradiction with successful theories of the macroscopic universe. The RECEPT project uses the LHCb experiment to search for traces of particles or forces from a more fundamental theory beyond the SM which reconciles these. In the process, RECEPT researchers are developing new methods of processing data in real-time which allows these searches to achieve their maximum potential. Project Funding Funded by the European Research Council (ERC) € 1,986,000 total funding. Contact Details Project Coordinator, Dr Vladimir Gligorov Laboratoire de Physique Nucléaire et de Hautes Energies Sorbonne Université, Campus Pierre et Marie Curie Tour 22 - 1er étage 4 Place Jussieu 75252 PARIS CEDEX 05 T: +33 1 44 27 43 34 E: Vladimir.Gligorov@cern.ch W: http://teamlhcb-lpnhe.in2p3.fr/ rubriqueRecept.html W: http://www.cnrs.fr W: https://lpnhe.in2p3.fr
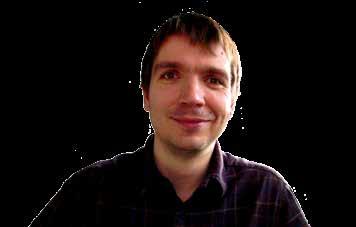
Dr Vladimir Gligorov
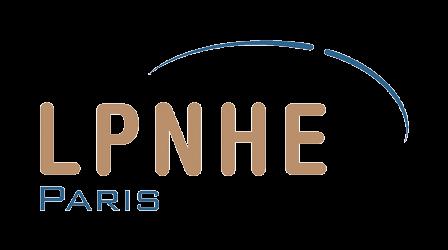
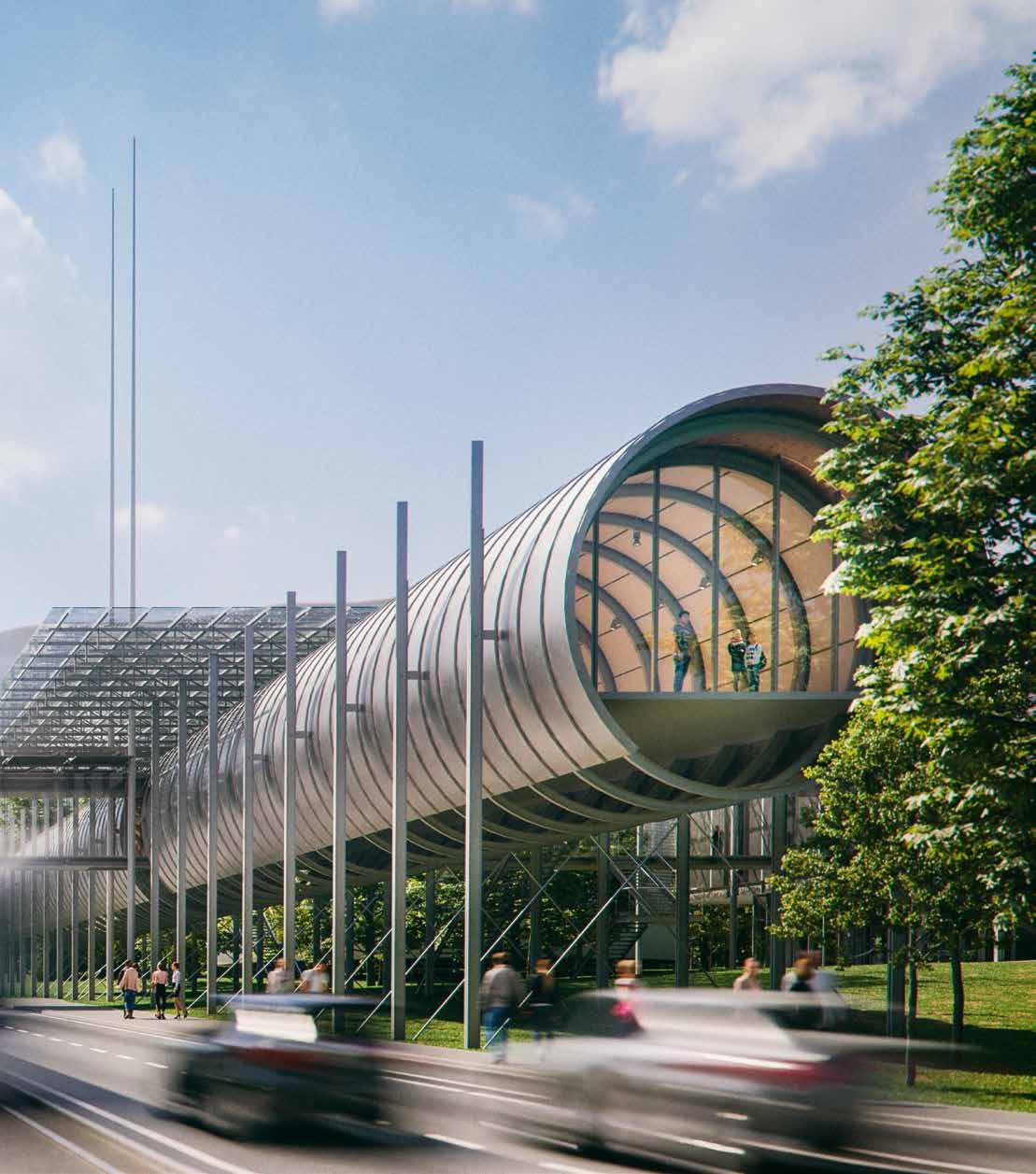
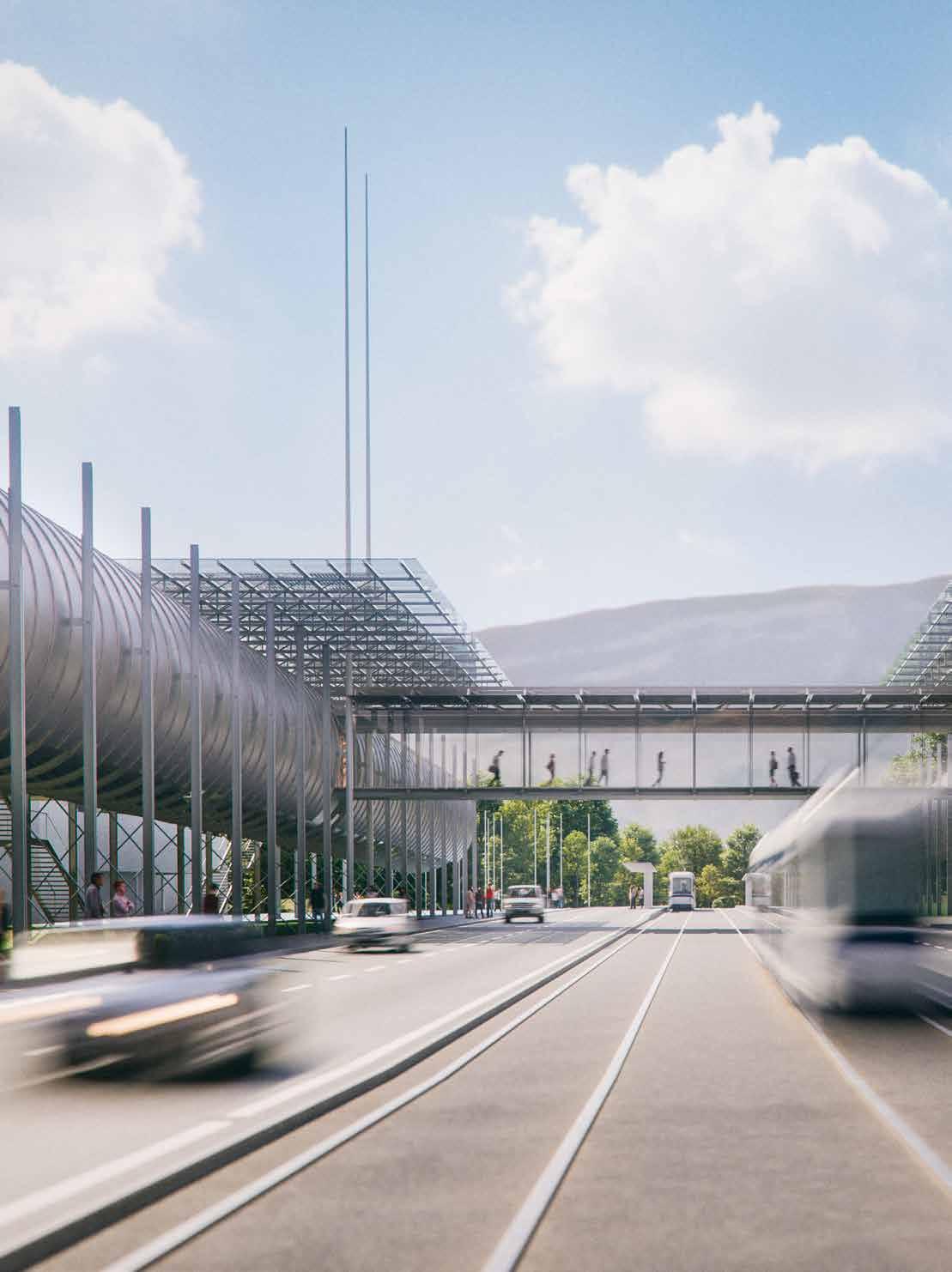
Dr Vladimir Gligorov is a research scientist at CNRS in Paris. He gained his undergraduate and doctoral degrees in physics from the University of Oxford, and completed his post-doc in Glasgow, before spending six and a half years working at CERN as a research scientist. As an experimental physicist, he specializes in the analysis of beauty hadron decay processes and real-time data processing.