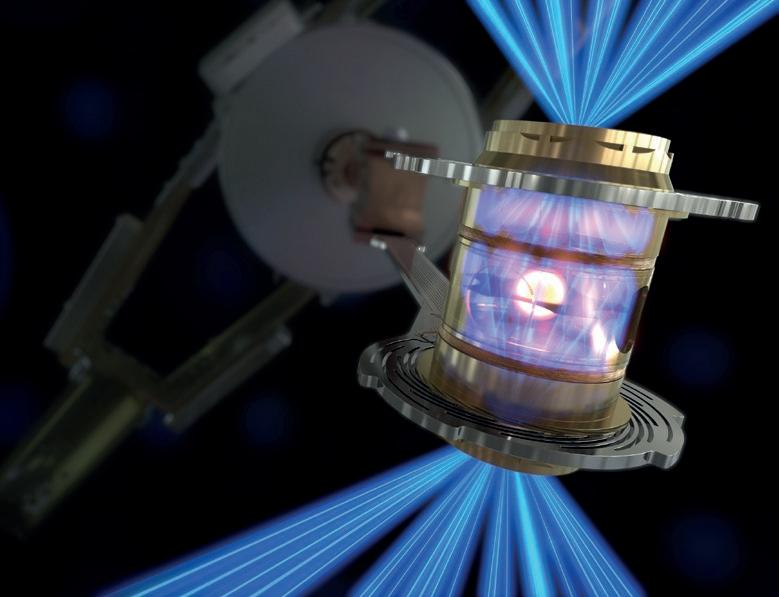
4 minute read
APPLICATION TECHNOLOGY
radiative equilibrium with the radiant energy within the cavity. This idealised cavity can be approximated in practice by making a small perforation in the wall of a hollow container of any opaque material.
Up to last December, experiments in both kinds of fusion had always required more energy to start them than was obtained from the reaction. In an experiment on 5th December at the NIF, 3.15 megajoules (MJ) of fusion energy was produced while the lasers made an input of 2.05MJ of energy into the target chamber. For the first time, a controlled fusion reaction had yielded more energy than was required to start it.
Advertisement
To do so, the beams are switched on for just a few billionths of a second and the target implodes inwards on itself at over 1 million miles per hour to heat and compress the fusion fuel to replicate the conditions at the centre of the sun. This is where the fusion reaction starts and releases both neutrons and alpha particles. The neutrons escape, but the alpha particles deposit their energy in the dense fuel, heating it even more. This causes a domino effect between fusion reactions, and more heating, and more fusion reactions, and so on. For a few tens of picoseconds (1 trillionth of a second), the fuel’s own inertia holds it in place while the fuel burns, giving rise to the name inertial confinement fusion.
The NIF laser fusion research is an international collaboration that includes the UK’s Central Laser Facility. Dr Robbie Scott, senior plasma physicist, Science and Technology Facilities Council at the Central Laser Facility and chair of the UK Inertial Fusion Consortium, is a member of the research team working on this project. He said: “…what a huge breakthrough it is for laser fusion research. More importantly, however, is the fact that it paves the way for rapid development of laser inertial fusion energy – power generation by laser fusion.”
UK Science Minister George Freeman said of the breakthrough: “This is a fantastic result that proves the exceptional potential of fusion power, and the National Ignition Facility team should be congratulated on their outstanding achievement. I’m proud that the Department of Business, Energy and Industrial Strategy funded Central Laser Facility was able to play a part in supporting the endeavour. Though there is still some way to go to deliver fusion power generation at scale, results like this illustrate that there is a viable route to commercial fusion energy ahead, and the UK is in pole position to build on this work towards a clean energy future.”
Better than fission
All current nuclear power stations derive their energy from the breaking apart of heavy elements, usually uranium. The fuel is expensive and dangerous to handle and when spent, it remains dangerous as a contaminant. Dealing with spent fuel remains a problem; it has to be stored for a hugely long time before its radioactivity falls to a safe level.
With fusion, there is no such problem. The fuel is isotopes of hydrogen, which is the most common element in the universe. However, to make it fuse, incredible temperatures and pressures are required. In a fission reactor, control rods slow the reaction to the required level, but there is always a fear that the rod system may be damaged and the core will go out of control and become a ‘melt down’. That’s one reason there is such concern about the fission reactors in Ukraine because of the war with Russia. Only a few people will also not know the name ‘Chernobyl’ where a fission reactor exploded in 1986. Fusion reactors have none of these problems. Even though they replicate the reactions at the core of stars (including our own sun), they stop at the slightest opportunity. The big challenge is to keep the reactions going.
Fuel feed in a practical reactor
As part of the process, NIF is using pellets of deuterium and tritium (DT pellets). Deuterium constitutes a tiny fraction of natural hydrogen, only 0.0153%, and can be extracted inexpensively from seawater. Tritium can be made with lithium, which is also abundant in nature. The amount of deuterium present in one litre of water can in theory produce as much energy as the combustion of 300 litres of oil. This means that there is enough deuterium in the oceans to meet human energy needs for millions of years.
Deuterium can be distilled from all forms of water. It is a widely available, harmless, and virtually inexhaustible resource. In every cubic metre of seawater, for example, there are 33 grams of deuterium. Deuterium is routinely produced for scientific and industrial applications. Tritium is a fast-decaying radioelement of hydrogen, which occurs only in trace quantities in nature. It can be produced during the fusion reaction through contact with lithium – it is produced or ‘bred’ when neutrons escaping the plasma interact with lithium.
One of the biggest challenges is how the fuel in a practical reactor will be delivered to the reaction chamber and how will it be secured while the laser impinge on it? Will it be a continuous flow system or will the fuel be delivered in pellets for a pulsed system? Either way, the fixing system will be a big challenge.
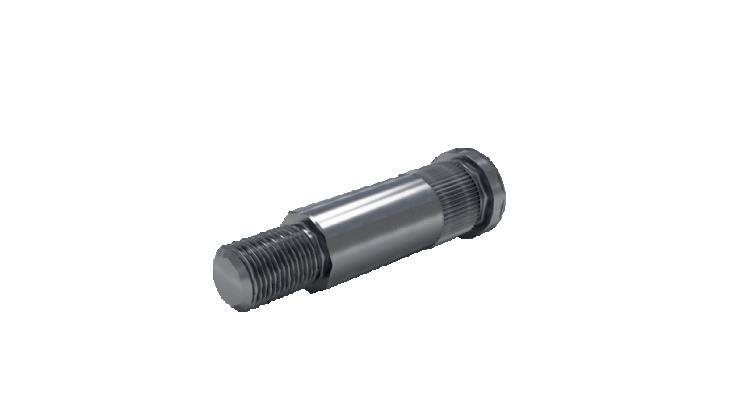
Patti Koning, public information officer in the Office of Strategic Communication at LLNL, explains: “One approach to an Inertial Fusion Energy (IFE) reactor, using Indirect Drive design (lasers shine into a hohlraum that get turned into x-rays to drive the capsule) would use a target assembly that consists of a fuel pellet (with the DT) sitting inside a hohlraum, where one of these fuel target assemblies would get dropped into the chamber 10 times a second. Both the hohlraum and pellet would get expended with each shot.
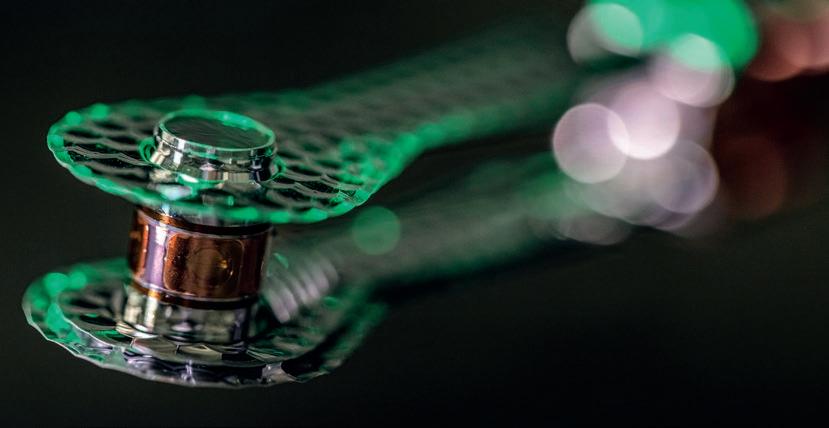
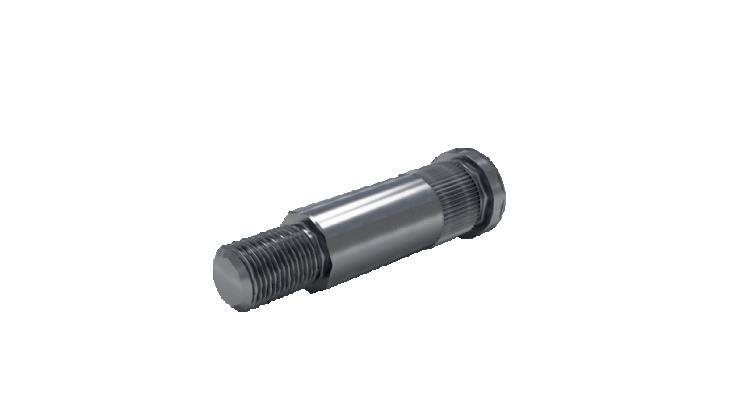