Retrofitting M elbourne’s high - rise social housing buildings: improv ing liveability and energy performance in a circular economy
Isabella Fyfe
The University of Melbourne, Melbourne, Australia i.fyfe@student.unimelb.edu.au
Supervisors:
Dr. Dominik Holzer
Dr. Behzad Rismanchi
Melbourne School of Design
Melbourne School of Engineering
The University of Melbourne 2023
Abstract:
Adopting circular economy principles such as retrofit is key for a low carbon transition in the built environment sector. Residential buildings are an important target for operational and embodied carbon reductions in Australia and transforming existing social housing buildings through retrofit presents a unique opportunity to address social and technical sustainability goals. However, social housing retrofits typically focus solely on technical improvements, including energy performance and embodied carbon reductions, meaning that social impacts like liveability are overlooked This thesis aims to propose a sociotechnical systems (STS) approach to the retrofit of Melbourne’s high-rise social housing buildings, to improve liveability and energy performance in the context of a circular economy. An STS decision support tool was developed, and its theoretical application demonstrated on the retrofit of a case study building through a ‘façade subtract and add’ design intervention. This approach was found to improve the liveability and energy performance of the building; however, further studies are required to assess the operational carbon savings against the embodied carbon required to deliver the intervention. This study shows that the retrofit of Melbourne’s high-rise social housing buildings is possible, and that it can be done in a way that improves liveability and energy performance with minimal disruption to residents.
1 Introduction
Mitigating climate change will require the decarbonisation of the built environment sector, which accounts for 39% of greenhouse gas (GHG) emissions globally (World Green Building Council, 2019). Adopting circular economy principles such as the retrofit is key for a low carbon transition (Intergovernmental Panel on Climate Change, 2022) Residential buildings account for almost one quarter of total energy use in Australia (Commonwealth of Australia, 2023) and are therefore an important target for both operational and embodied carbon reductions Within the residential building sector, transforming existing social housing buildings through retrofit presents a unique opportunity to address social and technical sustainability goals. Improving the liveability and energy performance of social housing, for example, could help to achieve the United Nations Sustainable Development Goals (UN SDGs) of making “cities and human settlements inclusive, safe, resilient and sustainable” and ensuring “access to affordable, reliable, sustainable and modern energy for all” (United Nations, 2016). However, social housing retrofits typically focus solely on technical improvements such as energy performance and embodied carbon reductions, meaning that social impacts such as liveability are overlooked (Baker et al., 2023) Hence, there is a need to balance technical and social retrofit aspirations to improve existing social housing buildings and the lives of those who live in them.
On 20 September 2023, the Victorian Government announced a significant urban renewal project called Victoria’s Housing Statement. Melbourne’s 44 high-rise social housing buildings will be demolished and replaced with a mixture of private and social housing between 2023 and 2051, resulting in the forcible relocation of approximately 10,000 residents (Victorian Government, 2023) Currently, there is no publicly available information that supports the government’s assertion that the buildings are derelict beyond the point of retrofit, and no potential retrofit alternatives have been proposed (Porter et al., 2023). This thesis aims to propose a socio-technical systems (STS) approach to the retrofit of Melbourne’s high-rise social housing buildings, to improve liveability and energy performance in the context of a
circular economy. The objectives of this study are to develop an STS decision support tool and demonstrate its theoretical application on the retrofit of a case study building through a design intervention. It is hoped that the findings in this paper will demonstrate to policymakers that the retrofit of Melbourne’s high-rise social housing buildings is possible, and that it can be done in a way that improves liveability and energy performance with minimal disruption to residents.
2 Literature review
This literature review includes an overview of the context surrounding high-rise social housing building retrofits, both in a global and Melbourne-specific context. Additionally, several example buildings are assessed against criteria in a background study conducted to identify different retrofit approaches. Finally, a gap analysis summarises key findings
2.1 High-rise social housing building retrofits
2.1.1
Context
Globally, social housing buildings are characterised by poor indoor environmental quality (IEQ) conditions and poor building thermal performance, putting low-income populations at higher health risk (Haddad et al., 2022) and negatively affecting liveability for residents. Retrofit is proposed as a cost-effective solution to concerns around energy performance, thermal performance, and quality issues (Baker et al., 2023) in the social housing sector. Furthermore, retrofit has the advantage of saving embodied carbon emissions associated with the demolition of existing buildings and the construction of new buildings (Giesekam et al., 2014). In a circular economy context, decisions to retrofit existing buildings are frequently based on technical aspects of building performance, including energy performance compared to new building standards, and assessment of environmental impacts, such as embodied carbon, of buildings over their lifetime (Crawford et al., 2014; Lalande et al., 2015). However, consideration of improving only technical aspects of building performance through retrofit such as energy or carbon can lead to undesirable effects (Vakalis et al., 2021); in a social housing context, adopting this approach means that social impacts are overlooked (Baker et al., 2023). However, it has been found that the refurbishment of social housing can simultaneously deliver significant energy improvements and improved living standards for residents (Crawford et al., 2014)
2.1.2
Opportunities
Onyszkiewicz and Sadowski (2022) conducted a review of several prefabricated Large Panel System (LPS) housing estate retrofits located predominately in Europe. They identified several best practice retrofit strategies that should be simultaneously considered during the retrofit process. These included social strategies, such as social participation, and detailed social and urban planning research, and technical strategies, including building evaluation, heat loss minimisation by transmission, façade and ventilation improvements, and energy performance simulations. The study highlights the technical and social benefits associated with an ‘add-on balcony system’ retrofit in eliminating existing thermal bridges, improving apartment functionality, enabling social participation by providing residents the opportunity to personalise space, and improving aesthetics of the building facades. Additionally, the study recommends the consideration of circular economy principles and prefabrication to reduce embodied carbon emissions associated with the use of new building materials. The re-use of dismantled façade elements in the retrofit of existing buildings is suggested as one way to achieve this.
Adopting a socio-technical systems (STS) approach to the retrofit of social housing buildings in the context of a circular economy can assist in balancing technical-dominated concepts of energy demand and embodied carbon reduction, alongside the people-focused experience of housing and liveability (Willand et al., 2019) STS are “material, conceptual or symbolic constructs that are characterised by the links between humans and materials, machines or technologies” that include several interacting elements that change over time and do not necessarily follow the rules of linearity or proportion (Shrubsole et al., 2018). Approaching social housing retrofits using an STS approach allows for greater comprehension and appreciation of how to manage the various complex social and material relationships at play (Ozarisoy & Altan, 2021). Several studies call for the adoption of this approach in projects that aim at improving building performance and social outcomes (Shrubsole et al., 2018; Willand et al., 2019)
2.2 Melbourne’s high-rise social housing buildings retrofit
2.2.1
Context
Melbourne’s high-rise (eight stories or more) social housing buildings were built between 1960 and 1975, comprising 45 buildings and 7,834 apartments, ranging from 27 m2 bedsitters to 96 m2 3-bedroom apartment units. The buildings were assembled in-situ using a structural system of precast concrete panel floors and walls, and have a projected life well into the 21st Century (Vella, 1990). Today, there are 44 high-rise social housing buildings across 21 estates in Melbourne (see Appendix 1). They account for 10% of the city’s social housing stock (McNeils & Reynolds, 2001) and house some of Australia’s most vulnerable people, including the low-income, elderly, and sick (Baeza et al., 2020). However, the buildings do not meet contemporary living standards due to decades of inadequate maintenance and repairs (Porter et al., 2023) and their poor IEQ conditions are negatively affecting the comfort, health, and wellbeing of residents (Jara-Baeza et al., 2023). Since the 1980s, retrofits of the buildings have been piecemeal, ranging from social initiatives aimed at improving resident wellbeing (McNeils & Reynolds, 2001), to technical glazing and insulation upgrades aimed at improving energy performance (Floyd, 2007). To date, no all-encompassing retrofit strategies to improve Melbourne’s high-rise social housing buildings have been proposed
2.2.2
Opportunities
There are some studies that identify potential retrofit opportunities for Melbourne’s high-rise social housing buildings. McNeils and Reynolds (2001) co-authored a review into the buildings that investigated ways to create better futures for residents. They found that upgrades to the apartments and common areas (including corridor spaces) could restore the buildings to contemporary housing standards, and address social issues of health, liveability, and poor amenity. Additionally, they found that technical upgrades to the windows and the external building (i.e. façade) could improve the poor energy performance of the buildings, which is attributed to poor shading of windows in summer and poor heat retention of windows in winter. However, to date, no in-depth energy performance analysis of the buildings has been published. Hence, these assumptions about potential technical retrofit opportunities are not well supported More recently, Jara-Baeza et al. (2023) conducted a holistic assessment of resident’s IEQ perceptions of the buildings. The study found that access to positive stimuli including fresh air, window control, natural light, and pleasant views (in order of importance) has several positive social outcomes, including improved wellbeing of residents. Additionally, the study found that pleasant views access can positively impact building-related health issues, suggesting a link between increased resident wellbeing and health, through the provision of access to positive stimuli.
2.3 Background study
A background study of existing high-rise social housing retrofits, both in Australia and globally, was conducted to determine the most effective socio-technical retrofit approaches.
2.3.1 Criteria
Six criteria (four social and two technical) were selected to evaluate five existing high-rise social housing retrofits. The evaluation criteria were selected based on key social and technical drivers relevant to the project. Example buildings were selected across the disciplines of architecture and engineering.
Social criteria
1. Fresh air access: resident’s ability to access fresh air from within apartment.
2. Window control access: resident’s ability to access window control from within apartment.
3. Natural light access: resident’s ability to access natural light from within apartment.
4. Pleasant views access: resident’s ability to access pleasant views from within apartment.
Technical criteria
5. Embodied carbon: embodied carbon emissions of an apartment retrofit.
6. Energy demand: amount of energy needed to heat and/or cool an apartment.
Objective ratings for each retrofit example were given based on available information in the literature. A normalized five-scale rating system was used (1 – Very Poor, 2 – Poor, 3 – Fair, 4 – Good, 5 – Very Good) to rate each retrofit example against the six criteria, with results of the comparison shown in Figure 1 (see Appendix 2 for justification of ratings). Weighted scores are shown in Table 1 with the criteria weighting and justification given in Table 2. A greater weighting is given to criteria deemed to be more significant, with consideration given to the findings and discussion in Sections 2.1 and 2.2.
Table 1 Background study weighted scores.
Table 2 Background study criteria weighting and justification.
Social 50% Since the primary focus of this study is to balance social and technical retrofit ambitions, a 50 per cent weighting was assigned to this category.
Fresh air access 20%
Window control access 10%
Access to fresh air from within the apartment was voted by Melbourne’s high-rise social housing residents as ‘Extremely important’ in Jara-Baeza et al.’s (2023) study which subjectively evaluated resident wellbeing. Hence, this criterion has the most significant weighting in the social category.
Access to window control from within the apartment was voted by Melbourne’s highrise social housing residents as ‘Very important’ in Jara-Baeza et al.’s (2023) study. Hence, this criterion was assigned a less significant weighting than ‘Fresh air access’ and equal weighting with the other social category criterion.
Natural light access 10% As above.
Pleasant views access
Technical 50% As above. Embodied carbon
As above.
Reducing both embodied and operational carbon emissions is necessary to decarbonise buildings. However, reducing embodied carbon emissions is becoming increasingly important. Hence, this criterion was given a greater weighting than energy demand.
Energy demand 20% Reducing energy demand (i.e. operational carbon emissions) is becoming less important as buildings become more energy efficient. Hence, this criterion was given a lesser weighting than embodied carbon.
2.3.2 Findings
The following findings arose from the objective assessment and subjective weighting of the five existing high-rise social housing retrofits presented in Section 2.3.1:
• Retrofit aspirations tend to focus primarily on either social or technical improvements. Cité du Grand Parc, for example, exemplified a strong social retrofit focus. Resident’s access to fresh air, window control, natural light, and pleasant views were significantly improved post-retrofit. However, whilst there were improvements to the energy demand of apartments, there were significant embodied carbon emissions associated with the provision of the new winter garden spaces constructed from concrete, glass, steel, polycarbonate sheeting, and aluminium. Opposingly, Ken Soble Tower demonstrated a strong technical retrofit focus with each apartment achieving Passive House certification. Whilst there were significant improvements to fresh air access for residents, other social considerations were not a priority in the retrofit.
• The reduction of operational carbon (i.e. energy demand) is prioritised over embodied carbon reductions across all case studies. Furthermore, whilst each retrofit had some focus on reducing embodied carbon (i.e. retaining certain components of the existing building), there was no exemplar case study that used predominately low embodied carbon new building materials or re-used existing building elements in the retrofit.
• Disruption to residents was noted with some case studies enabling residents to remain in-situ throughout the retrofit process (i.e. Cité du Grand Parc and Cedar Court) and others requiring the full decant and temporary housing of residents (i.e. Park Hill Phase 1, Park Hill Phase 2, and Ken Soble Tower).
• Figure 2 illustrates the different retrofit approaches demonstrated by the examples. Cité du Grand Parc = approach A, Park Hill Phase 1 = approach B, Park Hill Phase 2 = approach C, and Cedar Court and Ken Soble Tower = approach D. Approach A, ‘façade subtract and add’, demonstrates the most potential to improve building performance and social outcomes, supporting the findings in the study by Onyszkiewicz and Sadowski (2022).
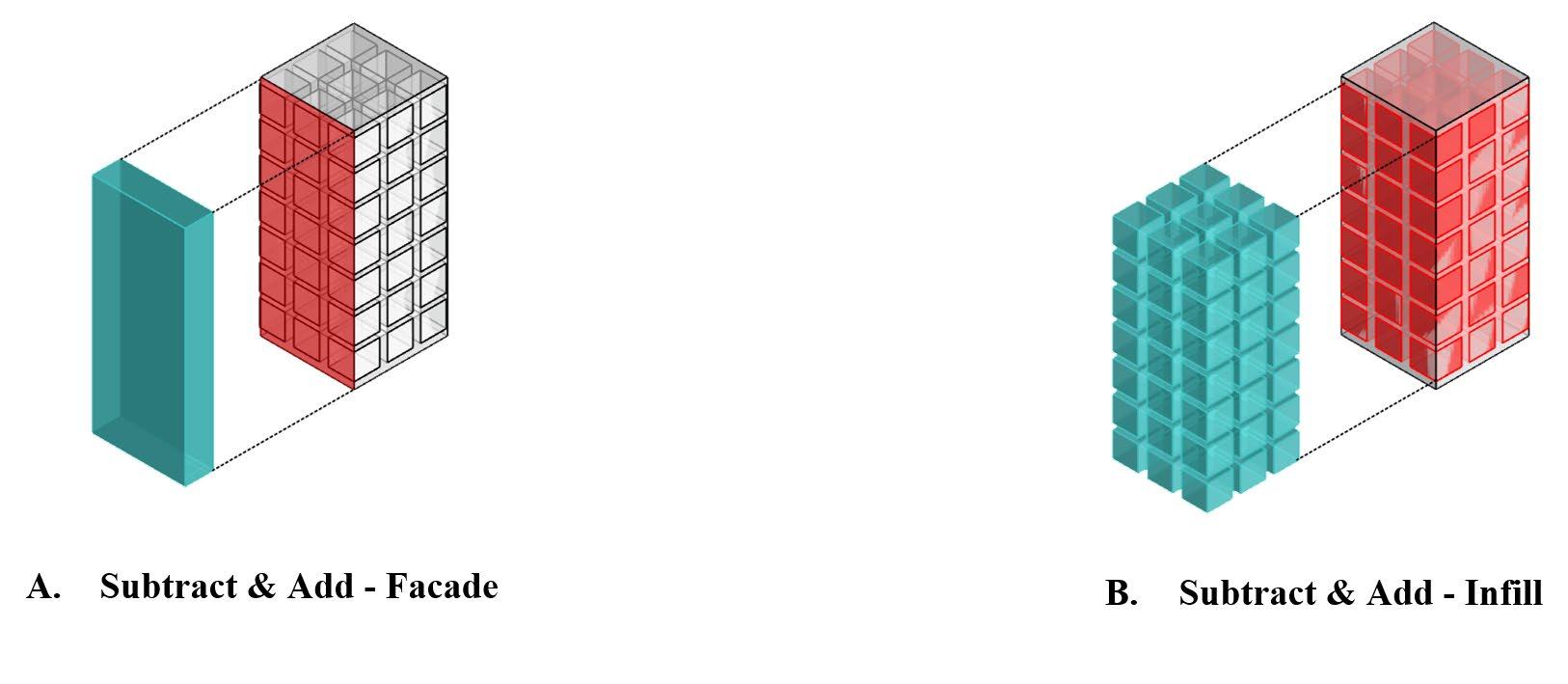
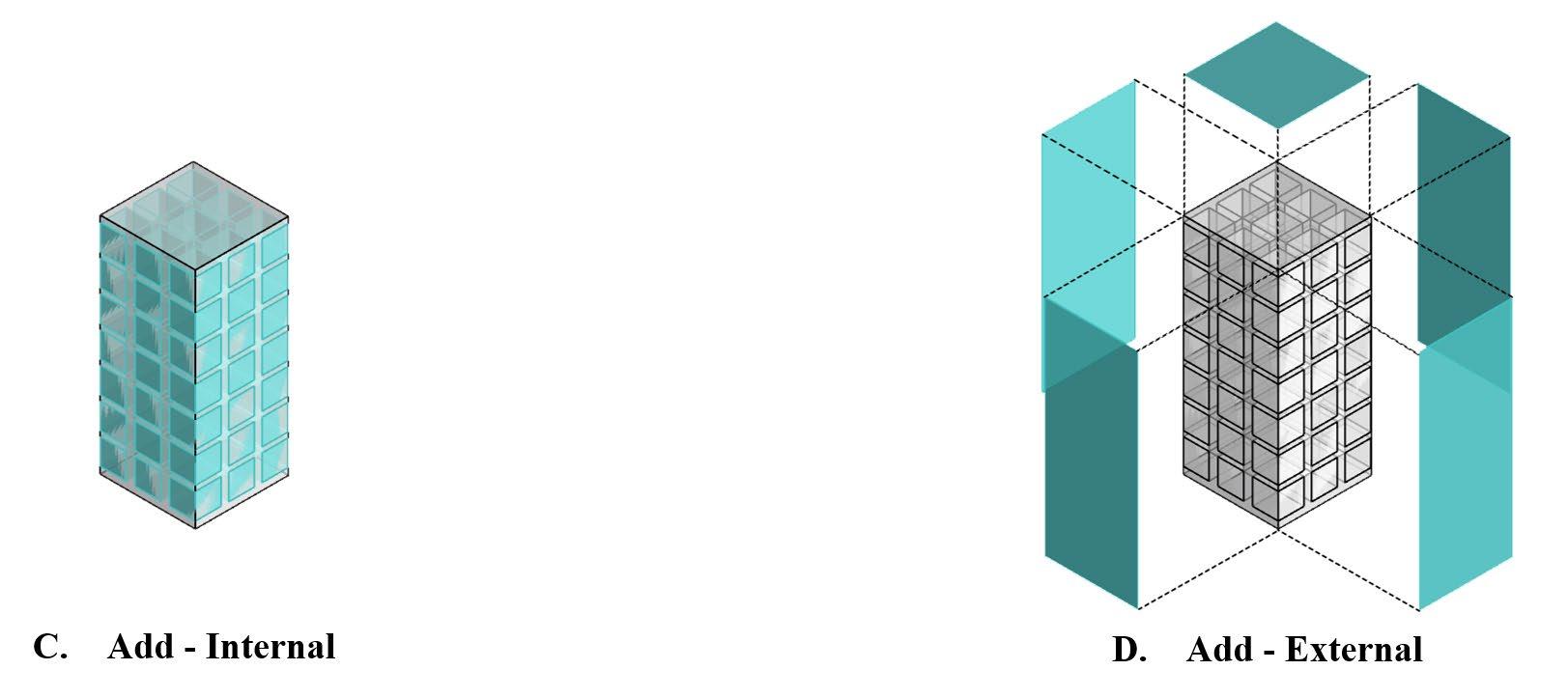
2.4 Gap analysis
The literature review has revealed that social housing retrofits typically focus on technical improvements, such as energy performance, meaning that social impacts including liveability are overlooked Hence, there is a need to balance technical and social retrofit aspirations to improve existing social housing buildings and the lives of those who live in them. To date in Melbourne no all-encompassing retrofit strategies have been proposed to improve the city’s existing high-rise social housing buildings. Furthermore, no in-depth energy performance or embodied carbon analysis of the buildings has been performed or published. The results of these analyses are essential to inform retrofit interventions aimed at improving energy performance by reducing energy demand in the context of a circular economy. From the background study and literature review, a ‘façade subtract and add’ approach was found to have the most potential to improve both building performance and social outcomes. Additionally, the research calls for a socio-technical systems (STS) approach to projects that aim at improving building performance and social outcomes, such as energy performance and liveability. Circular economy principles such as prefabrication, material re-use, and the use of low embodied carbon materials for the retrofit should be considered, all whilst aiming to minimise disruption to residents.
3 Methodology and methods
This thesis aims to propose a socio-technical systems (STS) approach to the retrofit of Melbourne’s high-rise social housing buildings, to improve liveability and energy performance in the context of a circular economy. The outcome of this study is the development of an STS decision support tool and demonstration of its theoretical application on the retrofit of a case study building through a ‘façade subtract and add’ design intervention. The methodological sequence followed to achieve this outcome is shown in Figure 3 The first step is the research phase comprising a literature review and background study (see Section 2). The second step is the critical analysis phase which consists of identifying the research gap (see Section 2) and developing the STS tool (Section 3). The final phase is the technical analysis and design phase (see Section 4) This phase includes the proposed design intervention that responds to the thesis aim. This section (Section 3) illustrates the process followed to develop the STS tool, including defining key terms, formulation of social and technical criteria, introduction of the case study building, and explanation of the methods followed, including key limitations and assumptions.
3.1 Development of STS tool
Before developing the STS tool, it was necessary to define the concepts of ‘liveability’ and ‘energy performance’, and ‘circular economy’ which are central to the thesis aim. Defining these concepts helped to determine a set of social and technical criteria for use in the tool.
3.1.1 Defining liveability
There is no single definition of liveability and its meaning varies greatly according to its context (Tennakoon & Kulatunga, 2019). However, liveability is increasingly seen as a mechanism to enhance the wellbeing and health of people (Badland et al., 2014), hence, the terms wellbeing and health are linked under liveability. Additionally, the Victorian Competition and Performance Commission’s definition of liveability links place and wellbeing beneath the term: “Liveability reflects the wellbeing of a community and comprises the many characteristics that make a location a place where people want to live now and in the future” (Commission, 2008). Finally, there is a close association between liveability and the social determinants of health, which include the built and natural environments, or place, in which people live (Rydin et al., 2012). Therefore, place and health are also linked under liveability. Holistically linking the concepts of place, wellbeing, and health under the umbrella term of liveability (see Figure 4) allows for better appreciation and understanding of the system as a whole (Badland & Pearce, 2019). Hence, this holistic approach to defining liveability was adopted for this study.
From the literature review, it was found that the poor IEQ conditions of Melbourne’s highrise social housing buildings are negatively affecting the health, and wellbeing of residents. Thus, improving these conditions is critical to improving the liveability of the buildings. Rohde et al. (2019) defines the domains of wellbeing and health through an IEQ lens as:
• Wellbeing is associated with indoor environmental conditions which improve occupants’ happiness through the presence of positive stimuli, providing control and offering variations (associated with psychological dimension).
• Health is attributed to the indoor conditions which contribute to physical resilience, limiting conditions leading to infirmity, disease, and years of life lost (related to physical and psychological dimensions).
However, the health and wellbeing of residents is not limited to IEQ conditions. Places that foster social connection, for example, are found to be beneficial for resident health and wellbeing (Bethell et al., 2021). Therefore, creating spaces that enhance and support social connectedness also directly impacts the liveability of place and is an important consideration in improving liveability.
The wellbeing of Melbourne’s high-rise social housing building residents was subjectively evaluated by Jara-Baeza et al. (2023) through questions on how important it to have access to several positive stimuli. In the absence of detailed sociological and anthropological research into the liveability of the buildings, the results of this study were used to determine a set of social criteria for use in the STS tool. The study found access to fresh air, window control, natural light, and pleasant views (in order of importance) to be the most important positive stimuli that affected the wellbeing of residents. Additionally, the study found that access to view satisfaction can have an impact on building-related health issues, suggesting a link between increased resident wellbeing and health through the provision of access to positive stimuli. Hence, the four positive stimuli (access to fresh air, window control, natural light, and pleasant views) from the study were included as social criteria in the STS tool. A fifth criteria, access to social connection, was also included since it is also a factor that impacts liveability.
3.1.2 Defining circular economy
Circular economy can be defined as maintaining the value of biological and technological resources for as long as possible in closed-loop systems (F. Mendoza et al., 2019). Adopting circular economy principles, such as retrofit, is key to reducing building-related embodied carbon emissions. Consequently, it is becoming increasingly important to consider embodied carbon across the whole building life cycle (Densley Tingley et al., 2018; World Green Building Council, 2019) In the decision-making process for environmentally responsible design, the results from an embodied carbon analysis can inform decisions around material reuse, and the use of low embodied carbon materials when new materials are required. Hence, embodied carbon was included as a technical criterion in the STS tool to inform retrofit interventions in the context of a circular economy.
3.1.3 Defining energy performance
Improving the energy performance of existing buildings through retrofit can significantly reduce energy demand (i.e. energy loads) and consequently environmental, economic, and social impacts (Belussi et al., 2019). In Australia, current regulatory requirements for the energy demand of residential dwellings are specified in the National Construction Code of Australia (NCC). The Nationwide Housing Energy Rating Scheme (NatHERS) is a pathway commonly used to demonstrate compliance with the NCC, with around 90% of new home designs being assessed using the Scheme (Australian Building Codes Board, 2022). NatHERS gives a star rating for a dwelling out of ten with a higher number of stars representing lower thermal operational energy demand. Recent changes to NCC 2022 require new residential dwellings to meet new heating and cooling load limits using a NatHERS compliance pathway. These limits equate to a NatHERS rating of 7 stars and are specified in the NatHERS heating and cooling load limits Standard 2022 (Commonwealth of Australia and the States and Territories of Australia, 2022).
Currently, no in-depth energy performance analysis of Melbourne’s high-rise social housing buildings has been performed or is publicly available. However, Sustainability Victoria (2019) estimates that housing in Victoria built before 1990 averages about 1.5 out of 10 NatHERS stars. Therefore, it can be reasonably assumed that Melbourne’s high-rise social housing buildings have a similar NatHERS rating and therefore, do not meet the current regulatory requirements for energy demand (since the buildings were constructed before 1990) Hence, the results of an energy performance analysis are necessary to inform retrofit interventions for the buildings to improve their energy performance by reducing energy demand. Thus, energy demand was included as the second and final technical criterion in the STS tool.
3.2 STS tool
The STS tool consists of seven criteria (five social and two technical) that were determined in Section 3.1. The tool can be used by architects and engineers in an iterative way to better meet the sensitives of Melbourne’s high-rise social housing buildings and residents’ liveability preference, resulting in more tailored retrofit design interventions. The tool will primarily benefit residents by improving liveability, however, co-benefits include increased public health, reductions in energy poverty, decarbonization and reduced environmental impacts of buildings, and climate change mitigation. A visual overview of the tool, including its social and technical criteria, is given in Figure 5 and its intended application sequence in the retrofit process for Melbourne’s high-rise social housing buildings is illustrated in Figure 6. This sequence can be repeated until the desired retrofit intervention is achieved.
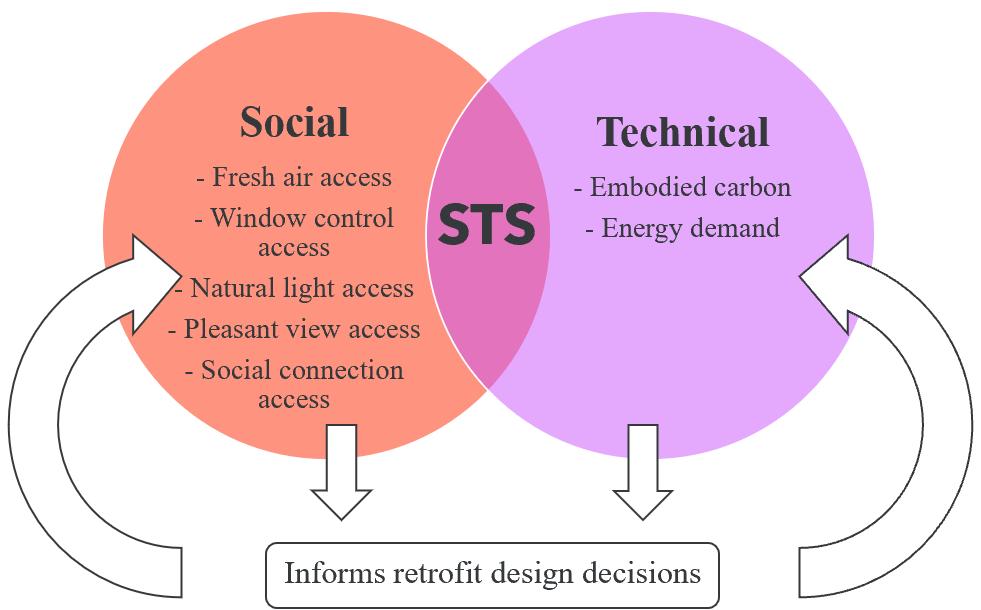
5 Socio-technical systems (STS) tool.
Technical analysis inc. energy demand and embodied carbon analyses
Qualitative assessment of social criteria
Informs retrofit design decisions
6 Socio-technical systems (STS) tool application sequence.
3.3 Case study building
A case study building was selected to demonstrate the theoretical application of the STS tool through a retrofit design intervention. The case study building is one of Melbourne’s existing high-rise social housing buildings, located within NatHERS Climate Zone 60 (Nationwide House Energy Rating Scheme, 2013) at 127 Gordon Street in Footscray, Melbourne. It is the only high-rise social housing building within the estate known as Gaskin Gardens (Vella, 1990) It is 13-storeys comprising 111 apartments of different configurations, including 89 x 1-bedroom apartments, 20 x bedsit apartments, and 2 x 2-bed apartments, across four different floor play typologies (see Appendix 3). The case study was selected based on the availability of documentation drawings and the shape of its plan The building’s T-shaped plan accounts
for the second highest proportion (20%) of buildings surveyed in Jara Baeza et al.’s (2023) study into resident’s IEQ perceptions of Melbourne’s high-rise social housing buildings. This study was instrumental in determining the socio criteria in the STS tool. Images of the case study building are shown in Figure 7
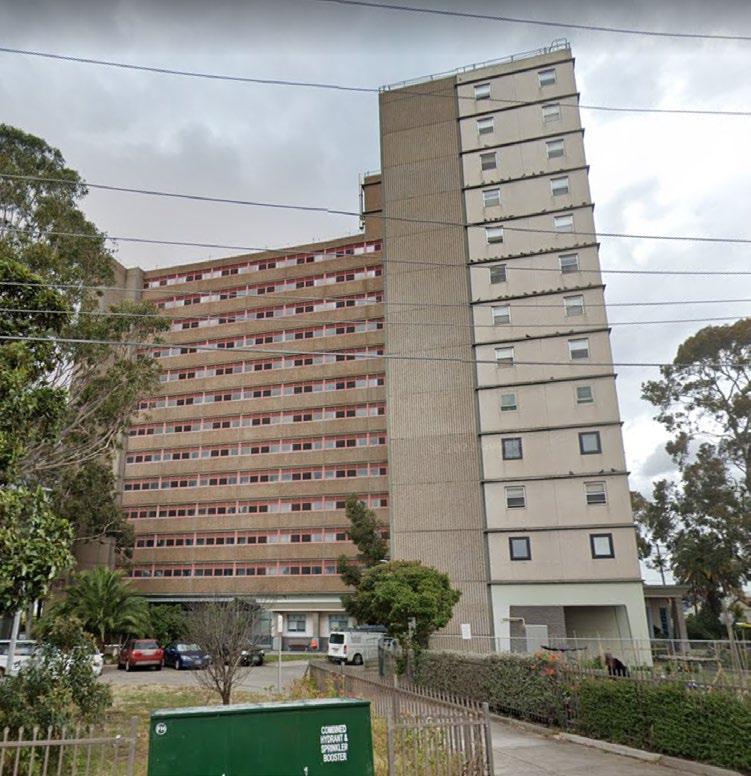
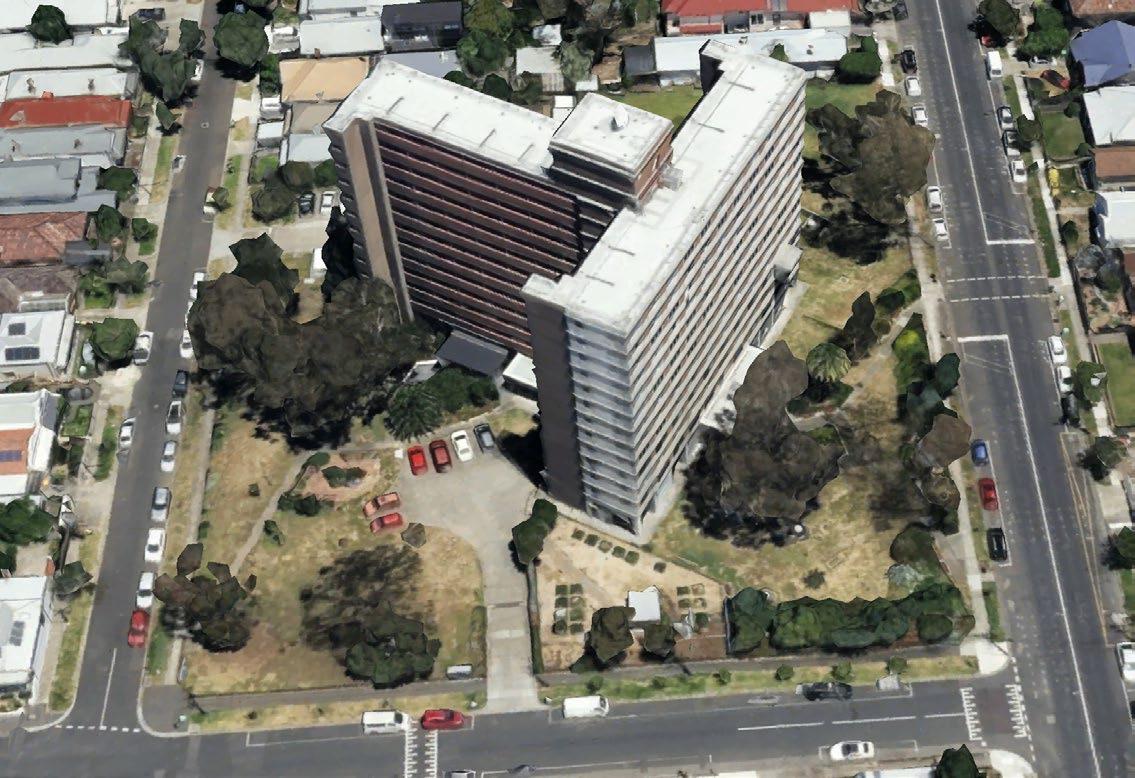
3.4 Methods
This section outlines how each criterion within the STS tool was assessed. The social criteria were qualitatively assessed, and the technical criteria were quantitively assessed. Section 4 demonstrates the application and results of these assessments for the case study building before and after the ‘façade subtract and add’ retrofit design intervention.
3.4.1 Social criteria
Objective ratings for the STS tool’s five social criteria were given using a normalized fivescale rating system (1 – Very Poor, 2 – Poor, 3 – Fair, 4 – Good, 5 – Very Good). This process was repeated both before (see Section 4.1.1) and after (see Section 4.3.1) the design intervention for the case study building. This enabled the comparison of the building’s liveability before and after its retrofit. The ratings were assigned based on detailed justifications provided in the Appendices.
3.4.2 Technical criteria
3.4.2.1 Embodied carbon
A life cycle assessment (LCA) can be used to quantify the environmental impact of a building across its whole life cycle in accordance with EN 15978:2011. However, this process is significantly complicated and time consuming. Therefore, an LCA assessment for this study was not performed given data limitations and time constraints. Instead, a different approach using material production and assembly processes data in the form of material embodied environmental flow coefficients from the Environmental Performance in Construction (EPiC) database was adopted. To estimate the embodied carbon of the case study building, material quantities were multiplied with hybridised environmental flow coefficients from the EPiC database (see Equation 1).
Equation 1 Embodied carbon equation using hybridised environmental flow coefficients.
The EPiC database was selected for this study because it comprises hybridised environmental flow coefficients for construction materials in an Australian-specific context. Due to the databases limitations, only the embodied carbon for the product stage (A1 – A3) was estimated for the case study building (see Figure 8). However, the majority of a building’s total embodied carbon is released upfront during the product stage (Carbon Leadership Forum, 2023), hence, diminishing the significance of this limitation.
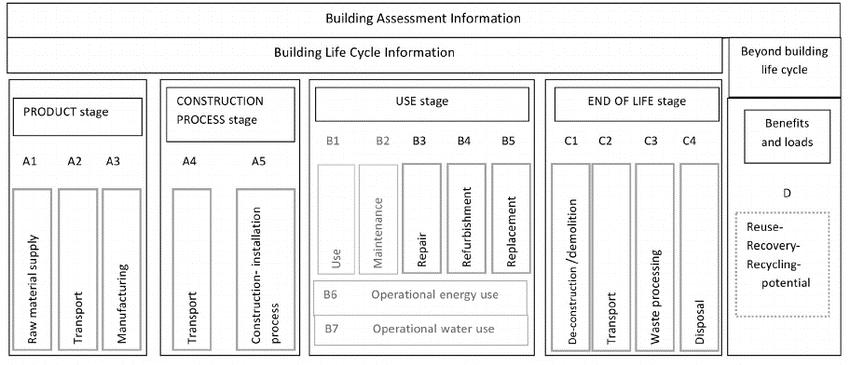
Key limitations associated with this method include difficulties in accurately determining construction material types and quantities due to limited access to the case study building and absence of a detailed construction material inventory. Hence material quantities were assumed based on photographs, documentation drawings, and the information provided in the thermal performance analysis report by Floyd (2007). It should be noted that only residential floors (levels 1 – 12) were considered in the embodied carbon analysis since these floors are the focus of the design intervention (see Figure 9).
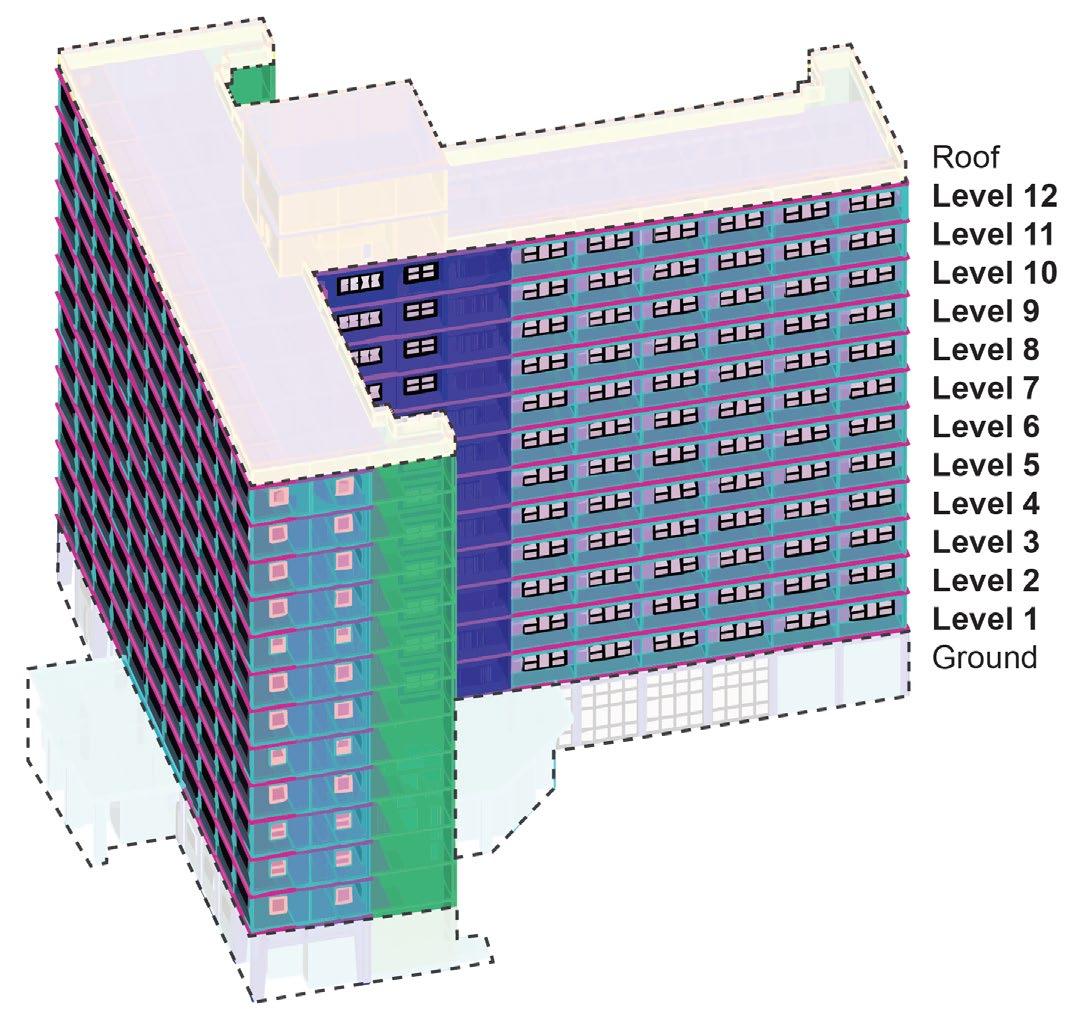
3.4.2.2
Energy demand
NatHERS accredited simulation engine software Home Energy Rating and Optimisation (HERO) was used to quantify the annual energy demand of the apartments within the case study building. HERO calculates the transient heat gains and losses of residential spaces, factoring the thermal performance of the building envelope, thermal storage, orientation, latent and sensible internal gains, cooling effects from cross ventilation and ceiling fans, hourly weather data and typical occupant behaviours (Willand et al., 2019). Air permeability rates and provision, demand or location of HVAC systems are excluded from the software calculations. A typological approach was taken to the energy demand analysis of the case study building. Eleven apartments (see Figure 10) representative of all apartment typologies within the case study building (see Appendix 4) were modelled in HERO. For simplicity, it was assumed that the results from these analyses represented all apartments within the case study building. However, since the ceilings of apartments located on level 12 are located adjacent to the building’s roof space, they are assumed to have a lower energy performance rating compared to apartments on levels 1 – 11. This is because there is an increase conduction heat transfer areas compared to other floors, resulting in more heat loss (Yoon et al., 2019)
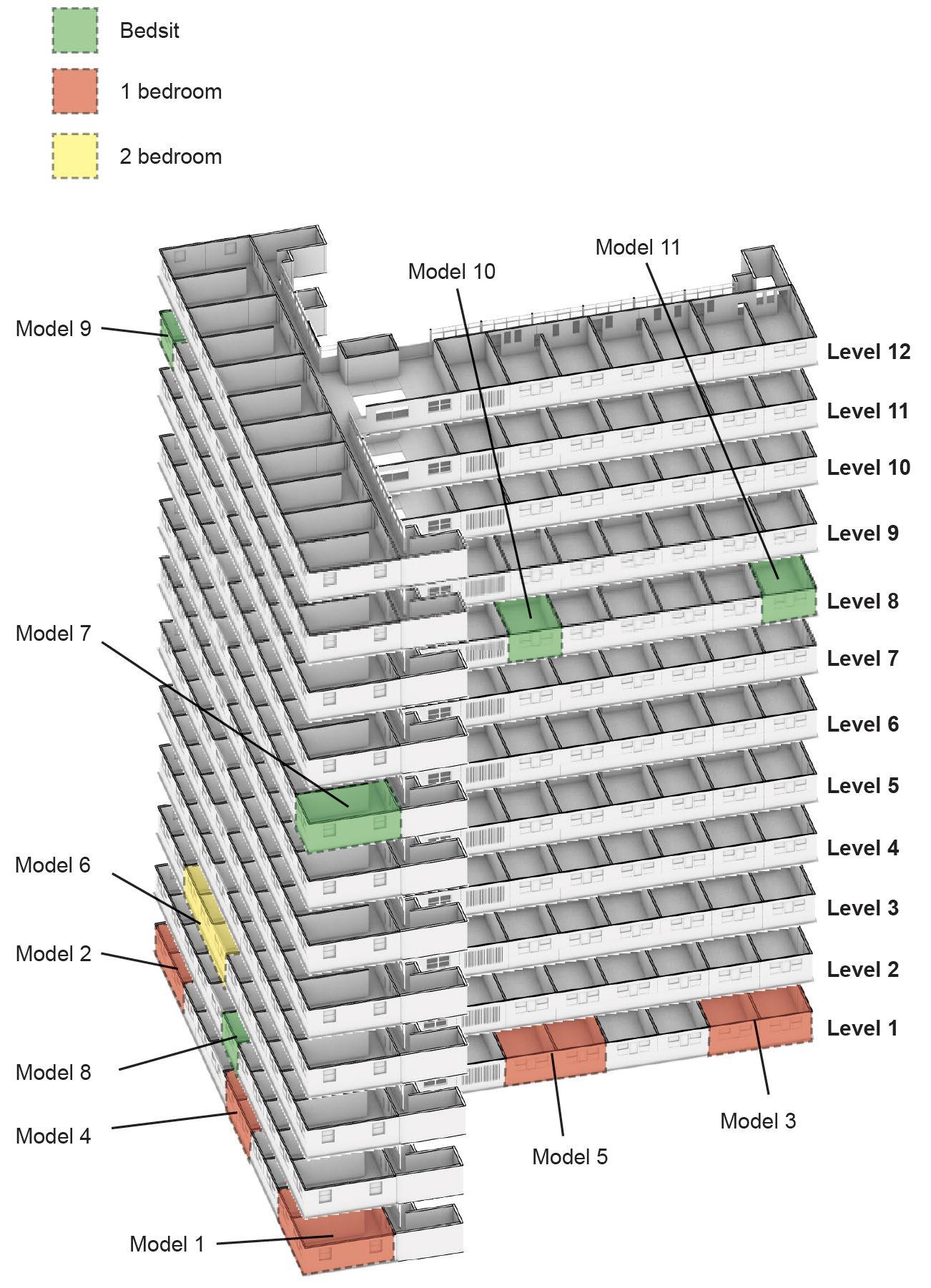
HERO has several assumptions built into the software including assumptions on occupant behaviour, openability of windows and doors etc. Where possible, these assumptions were manipulated to better reflect the case study building in accordance with the NatHERS Technical Note (29 September 2023) document. See Appendix 5 for a full list of the assumptions made for the HERO energy demand analysis. Furthermore, access to the case study building was restricted, therefore, several assumptions regarding apartment typologies, layouts, configurations, materiality etc. were made based on photographs and documentation drawings produced by BKK Architects, Peter Elliot Architecture and Urban Design, and Taylor Cullity Lethlean on 23 August 2011. It is known that several glazing and insulation improvements have been made to individual apartments within the case study building to improve their energy performance (Floyd, 2007). However, these improvements were not applied globally. Therefore, several assumptions about the building’s construction materials were made based on photographs, documentation drawings and the thermal performance analysis performed by Floyd (2007)
4 Results and analysis
This Section presents analysis performed on the case study building in accordance with the social and technical criteria STS tool, both before and after the proposed retrofit design intervention. See Section 5 for detailed discussion of the results.
4.1 Before retrofit
Results from the social and technical analysis of the case study building before the retrofit intervention are given below.
4.1.1 Social criteria
The five social criteria from the STS tool were objectively rated using a normalized five-scale rating system (1 – Very Poor, 2 – Poor, 3 – Fair, 4 – Good, 5 – Very Good) with results shown in Table 4 below. Refer to Appendix 6 for detailed justification of the ratings.
Table 3 Social criteria objective ratings before retrofit.
Social criteria Score
Fresh air access 2
Window control access 2
Brief justification
Windows = primary source of fresh air access for the apartments. In corridor spaces, there is always the provision of fresh air through vents located above the windows which are permanently open.
Residents can operate most windows in their apartments (awning and double hung). Some windows are fixed and cannot be operated. Vents and windows in corridor spaces are completely inoperable.
Natural light access 4 Jara-Baeza et al. (2023) found that residents had
Pleasant view access 3
All apartments have unobstructed pleasant views on the apartment-facing façade. Views are obstructed on the corridor side due to the provision of the half-height cold rolled steel wall and vents.
Social connection access 2 Limited access to social connection due to narrow corridor width.
4.1.2 Embodied carbon
The total embodied carbon for levels 1 – 12 of the case study building was found to be 1,658 tCO2e (see Table 4). The results show that concrete (floors, walls, and fire stairs) accounts for 51%, followed by cold rolled steel (corridor façade panels) and aluminium extruded powder coated (window frames and vents), which account for 20% and 12% of the embodied carbon for levels 1 – 12 respectively (see Figure 11). Appendix 7 provides justification of the material types selected for the embodied carbon calculations and shows detailed calculations for a typical floor.
Table 4 Embodied carbon results (levels 1 – 12) by material type before retrofit
3%
Plasterboard - 10mm 0%
Figure 11 Percentage breakdown of embodied carbon (levels 1 – 12) by material type before retrofit
4.1.3 Energy demand
The results from the typological approach energy demand analysis for the case study building (see Table 5) show that the average NatHERS rating for the apartments is 2.1 stars, with an annual average heating load, cooling load, and total load of 419.9 MJ/m2, 11.5 MJ/m2, and 410.0 MJ/m2, respectively (see Table 6). Additionally, the highest NatHERS rating achieved was 3.4 stars for model 8 and the lowest NatHERS rating achieved was 0.8 stars for model 11. The highest annual average heating load, cooling load, and total load were found to be 671.4 MJ/m2, 21.5 MJ/m2, and 690.0 MJ/m2, respectively. The lowest annual average heating load, cooling load, and total load were found to be 243.6 MJ/m2, 4.0 MJ/m2, and 251.3 MJ/m2, respectively. Appendix 8 presents the case study building material assumptions used in the energy modelling software and Appendix 9 provides a detailed overview the energy models for the apartments that achieved the highest (B.2.4) and lowest (C.8.10) NatHERS ratings respectively.
Table 5 Energy demand analysis results.
Table 6 Highest, lowest, and average values from energy demand analysis.
4.2 Retrofit design intervention
The results presented in Section 4.1 were used to inform a retrofit design response for the case study building in accordance with the STS tool application sequence in Figure 6. A retrofit design intervention for the communal corridor spaces on the residential floors was proposed because these spaces offer the greatest potential for improved access to social connection. The intervention takes inspiration from the background study and literature review findings presented in Section 2 which found a ‘façade subtract and add’ approach to retrofit had the most potential to improve both building performance and social outcomes. Hence, this approach was adopted to create a liveable thermal corridor zone adjacent the existing façade of the case study building, drawing architectural inspiration from the Lacaton & Vassal retrofit example (Cité du Grand Parc in Bordeaux, France) examined in the background study. Circular economy principles such as prefabrication, material re-use, and the use of low embodied carbon materials were considered. Additionally, minimising disruption to residents was also a key focus of the intervention. Details of the retrofit intervention, including documentation drawings, are given in Appendix 10
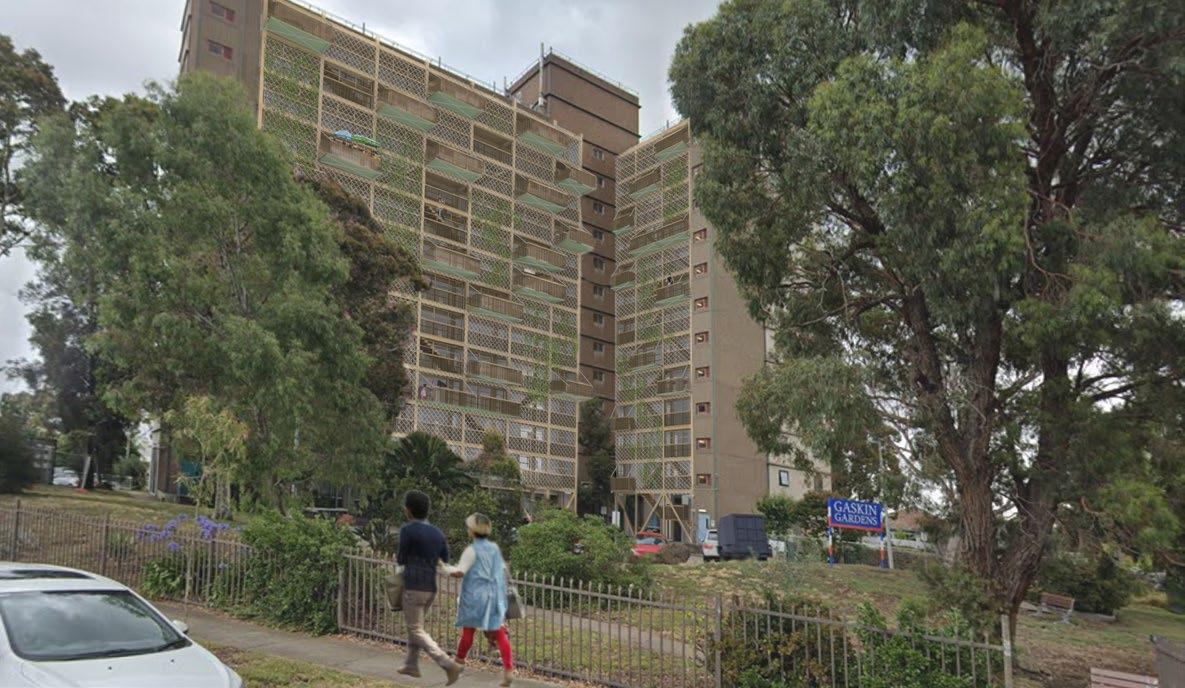
4.3 After retrofit
Results from the social and technical analysis of the case study building after the retrofit intervention are given below.
4.3.1
Social criteria
Again, the five social criteria from the STS tool were objectively rated using a normalized five-scale rating system (1 – Very Poor, 2 – Poor, 3 – Fair, 4 – Good, 5 – Very Good) with results shown in Table 4 below. These results reflect the liveability of the case study building after the retrofit intervention. See Appendix 11 for detailed justification of the ratings. From the results it was found that after the retrofit intervention, the liveability of the case study building significantly increased (see Figure 13).
Table 7 Social criteria objective ratings after retrofit.
Social criteria Score
Fresh air access 4
Window control access 4
Natural light access 5
Pleasant view access 5
Social connection access 5
Brief justification
Increase in area on the corridor façade that can be opened to provide fresh air access, subsequently improving the fresh air access to the apartments through the kitchen and bathroom windows adjacent the corridor.
Post retrofit, residents can fully control windows in the corridor spaces. There is no change to the window control access in the apartments.
Although increased corridor width may reduce some provision of natural light to the kitchen, bathroom, and corridor spaces, this is assumed to be mitigated by provision of floor to ceiling semi-opaque polycarbonate sliding doors.
Again, the provision of semi-opaque floor to ceiling polycarbonate sliding doors in place of the half-height cold rolled steel wall and aluminium powder coated vents significantly increases access to pleasant views.
Additional balcony area transforms corridors from transitory spaces to liveable spaces where people connect with other residents.
Figure 13 Before and after retrofit intervention – social criterion comparison radar diagram.
4.3.2
Embodied carbon
The total embodied carbon for the retrofit intervention was found to be 552 tCO2e (see Table 8). The results show that the glulam structure accounts for 42%, followed by polycarbonate (sliding doors) and stainless-steel wire (façade mesh), which account for 27% and 18% of the
embodied carbon for the retrofit intervention respectively (see Figure 14). The retrofit intervention accounts for 25% of the building’s total embodied carbon (see Table 9)
Importantly, the retrofit enabled the re-use of the existing cold rolled steel elements of the original corridor façade, saving 20% of the building’s existing embodied carbon. Appendix 12 provides justification of the material types selected for the embodied carbon calculations and shows detailed calculations.
Table 8 Embodied carbon results by material type after retrofit.
14
Table 9 Total embodied carbon for case study building including before and after retrofit
4.3.3 Energy demand
The retrofit design intervention was tested against the highest (apartment B.2.4) and lowest (apartment C.8.10) NatHERS rated apartments from the energy demand analysis. After the retrofit, the NatHERS energy rating for apartment B.2.4 (model 8) increased from 3.4 to 5.2 stars whilst apartment C.8.10 (model 11) increased from 0.8 stars to 1.4 stars (see Table 10). Interestingly in both cases the annual heating demand significantly decreased, and the annual cooling demand increased slightly. This resulted in a total annual energy demand reduction for apartment B.2.4 from 250.5 MJ/m2 to 160.3 MJ/m2, or 36%, and from 706.1 MJ/m2 to 514.5 MJ/m2, or 27%, for apartment C.8.10 (see Table 10). Appendix 13 provides a detailed overview the energy models.
Table 10 Energy demand analysis results before retrofit.
5 Discussion
5.1 Advantages
The results from this study shown that the development and use of the socio-technical systems (STS) tool to approach the retrofit of Melbourne’s high-rise social housing buildings has the following advantages:
• The use of the tool can help architects and engineers to balance technical-dominated concepts of energy demand and embodied carbon reduction, alongside the people-focused experience of liveability (Willand et al., 2019)
• The tool brings to light the complex relationship between embodied and operational carbon. It allows designers and decision-makers to weigh potential operational carbon savings from improved energy performance against the embodied carbon required to deliver these savings.
• The tool has the potential to be applied to Melbourne’s other high-rise social housing buildings and the social criteria adjusted to better reflect liveability requirements of residents on a case-by-case basis. Additionally, the tool has the potential to assign weightings to the social and technical criteria. These weightings could be adjusted to suit different retrofit aspirations (i.e. equally balanced social and technical aspirations or retrofit aspirations more weighted either towards social or technical aspirations).
Additionally, this study has demonstrated that the proposed ‘façade subtract and add’ retrofit intervention design approach has the following advantages:
• The approach shows significant potential in improving social outcomes for residents, particularly by improving access to social connection through the provision of liveable spaces that also double as a thermal barrier, insulating the existing building. This
insulating thermal barrier is shown to have an additional benefit of improving the thermal performance of the building, evidenced by reduced energy demand for the apartments.
• The design intervention has the potential to improve access to social participation by providing residents with the possibility of arranging spaces according to their living preferences (Onyszkiewicz & Sadowski, 2022).
• The intervention can be installed with minimal disruption to residents, with the structure being erected alongside the existing building. There are additional social and economic benefits of not having to decant or relocate residents during this process (Crawford et al., 2014)
• The proposed retrofit intervention has the potential to be applied to the non-loadbearing kitchen/living room facades of the case study building which may result in further operational carbon savings from improved energy performance. Additionally, there may be improved liveability benefits associated with increased access to positive stimuli and social connection.
• The intervention can be scaled, adapted, and applied to Melbourne’s other high-rise social housing buildings due to their similar design, construction, building elements, and material characteristics, resulting in far-reaching potential positive social and environmental impacts.
5.2 Limitations
There are several limitations associated with this study, including the following:
• Economic factors were not considered as part of this study.
• A detailed structural analysis of the proposed retrofit design intervention was not performed.
• A retrofit intervention was only proposed for the communal corridor spaces, therefore, for example, excluding additional potential social and technical benefits that could be achieved through the provision of the intervention to the apartment facades.
• A structural analysis of the overall condition of Melbourne’s high-rise social housing buildings is necessary to determine the scope and timeframe of any potential retrofit interventions. Once this information is known, potential operational carbon savings from improved energy performance over the building’s remaining life can be weighed against embodied carbon emissions required to deliver these savings.
• Energy performance calculations after the retrofit intervention were only performed for the highest and lowest NatHERS rated apartments. Additionally, only the enclosed situation (i.e. when all sliding doors are closed) for the proposed retrofit intervention was modeled. It is anticipated that results will differ for the case where the corridor spaces are open (i.e. when all sliding doors are open)
• The use of hybridised environmental flow coefficients resulted in a conservative estimate of the case study building’s embodied carbon. This is because the coefficients can vary greatly, particularly in terms of geographic (i.e. transport distances) and temporal factors (i.e. different manufacturing processes and efficiencies over time) (Reap et al., 2008) Hence, the results are not accurate given that manufacturing processes, transport, and construction processes have become more efficient over time (Crawford & Stephan, 2021). However, the results show the amount of upfront embodied carbon required to replace a building of a similar typology today.
• In the absence of detailed sociological and anthropological research into the liveability of the case study building, the results of the Jara-Baeza et al. (2023) study were used to determine a set of social criteria for use in the STS tool.
• The low value of the annual cooling demand results presented in Table 5 and Table 10 was noted Relative compactness, which is defined as the ratio of volume to surface area, is a building typology factor that directly influences the cooling energy demand in high-rise buildings, with higher ratios associated with lower cooling energy demand and therefore total energy demand (Anaya et al., 2021). For the apartments in the case study building, the amount of wall area exposed to ambient conditions is minimal (predominately the living room/kitchen façade since the corridor façade is shaded and partially enclosed). Hence, the apartments have a high relative compactness ratio which may explain the low annual cooling demand results
5.3 Recommendations
• There is considerable diversity between Melbourne’s high-rise social housing buildings. The buildings vary in size, shape, context, location, orientation, construction date, condition, maintenance history, renovations and alterations, social history etc. Therefore, it is important that sociological and anthropological research for each building. The results can then be used to determine a set of social criteria for use in the STS tool that is unique to each building.
• The provision of the retrofit intervention to the kitchen/living room apartment facades should be modelled and analysed to determine other potential liveability and energy performance improvements in the context of a circular economy
• A detailed design for the structure and connection joints of the retrofit intervention should be performed to assess its feasibility. Additionally, prototypes could be designed and constructed to test joints and monitor the performance of materials, especially with regards to their durability.
• It is important to assess the amount of embodied carbon required to implement the retrofit intervention to see how it weighs against potential operational carbon savings from energy performance improvements. A structural analysis of the buildings is necessary to determine the potential scope of the project and inform this analysis.
• It is evident that the building’s concrete elements contribute most significantly towards the building’s embodied carbon. Therefore, all proposed retrofit interventions for the buildings should retain these elements as much as possible.
• There is potential for the STS tool to assign weighted scores to both the social and technical criteria with greater weighting given to criteria deemed to be more significant. This would result in unique design solutions and retrofit outcomes tailored to each building and their residents
• The façades of the buildings should be insulated where the ‘façade subtract and add’ retrofit intervention cannot be applied to minimise heat loss by transmission (Yoon et al., 2019).
• A life cycle analysis that considers the other stages from EN 15978:2011 could be performed to analyse the impact of the project over its entire lifecycle For example, the sourcing of the materials could greatly influence the sustainability of the project and its impacts on the environment regarding embodied carbon.
• An energy performance assessment for the remaining nine energy models after the retrofit intervention could be performed to determine if the improved energy performance trend is consistent across all apartment typologies. Additionally, modelling for the case where the corridor spaces are open (i.e. when all sliding doors are open) should be performed to provide a more holistic overview of how the retrofit intervention performs in different situations.
• Economic studies should be performed to determine the feasibility of the retrofit design intervention in a real-world scenario.
6 Conclusion
As part of this study, an STS decision support tool was developed, and its theoretical application demonstrated on the retrofit of one of Melbourne’s high-rise social housing buildings through a ‘façade subtract and add’ design intervention (see Figure 15). It was found that the intervention, which comprised a low embodied carbon prefabricated glulam structure erected alongside the existing corridors that re-used the existing cold rolled steel facade elements, could improve the liveability and energy performance of the building, all whilst reducing embodied carbon impacts and minimising disruption to residents. Analysis of the building’s liveability, embodied carbon, and energy demand, performed both before and after the intervention, was used to support this finding. It is anticipated that the intervention could be applied to the apartment facades, further contributing to improved liveability and energy performance outcomes for the building. Additionally, it is thought that intervention can be scaled, adapted, and applied to Melbourne’s other high-rise social housing buildings due to their similar design, construction, building elements, and material characteristics, resulting in far-reaching potential positive social and environmental impacts.
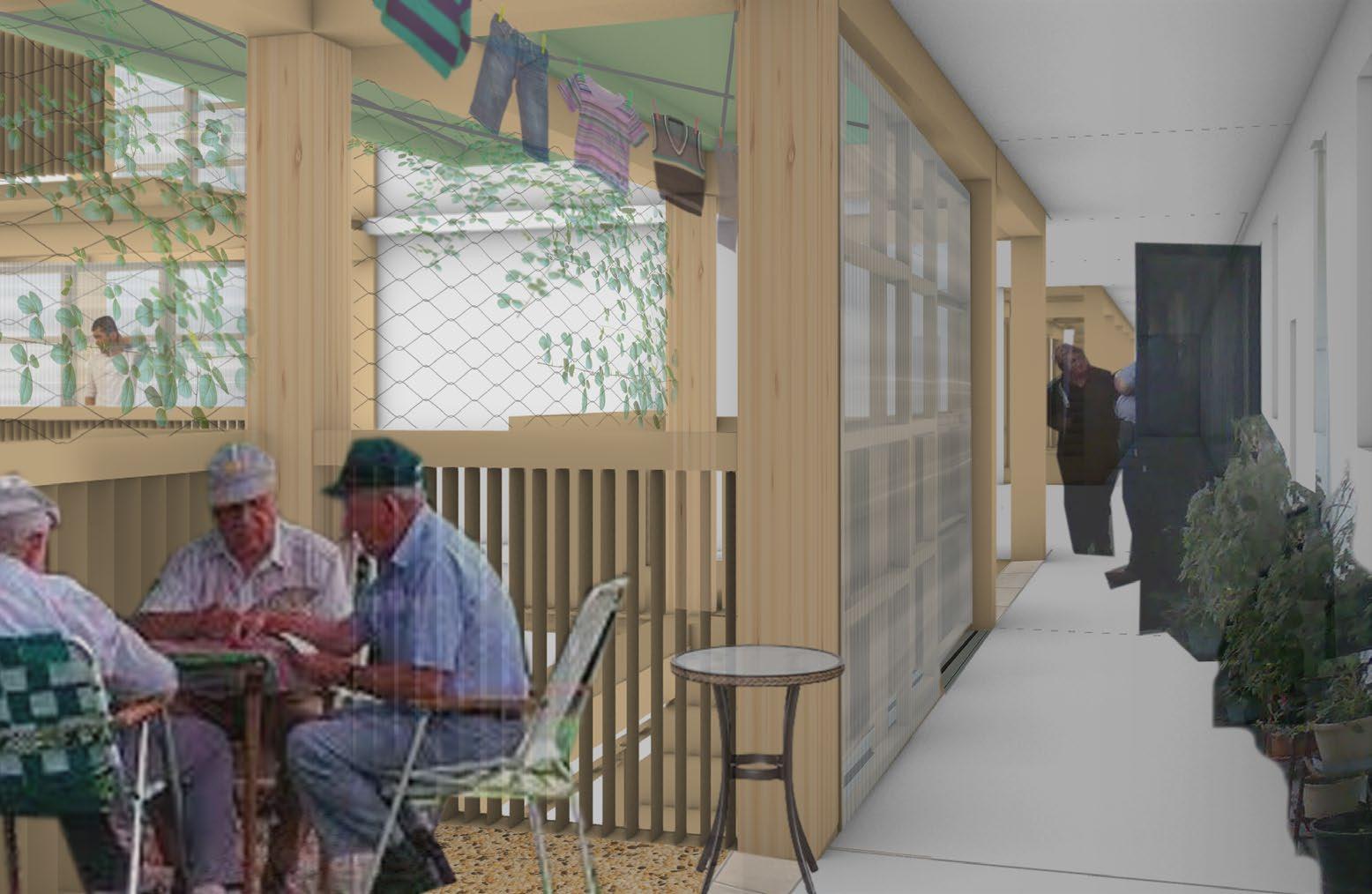
7 References
Anaya, B., Hou, D., Hassan, I., Wang, L., & Rahman, A. (2021). INFLUENCING FACTORS ON COOLING DEMAND OF HIGH-RISE BUILDINGS IN HOT/HUMID CLIMATES: A REVIEW https://doi.org/10.2495/SC210351
Australian Building Codes Board. (2022). Building for 7 Stars: top tips and guidance. Retrieved 2 October from https://ncc.abcb.gov.au/news/2022/building-7-stars-top-tipsand-guidance
Badland, H., & Pearce, J. (2019). Liveable for whom? Prospects of urban liveability to address health inequities. Social Science & Medicine, 232, 94-105. https://doi.org/https://doi.org/10.1016/j.socscimed.2019.05.001
Badland, H., Whitzman, C., Lowe, M., Davern, M., Aye, L., Butterworth, I., Hes, D., & Giles-Corti, B. (2014). Urban liveability: Emerging lessons from Australia for exploring the potential for indicators to measure the social determinants of health. Social Science & Medicine, 111, 64-73. https://doi.org/https://doi.org/10.1016/j.socscimed.2014.04.003
Baeza, F. J., Rajagopalan, P., & Andamon, M. M. (2020). Reviewing indoor environmental quality of high-rise social housing. Proceedings of the International Conference of Architectural Science Association,
Baker, E., Moore, T., Daniel, L., Cairnes, R., Padilla, H., & Lester, L. (2023). Sustainable social housing retrofit? Circular economy and tenant trade-offs.
Belussi, L., Barozzi, B., Bellazzi, A., Danza, L., Devitofrancesco, A., Fanciulli, C., Ghellere, M., Guazzi, G., Meroni, I., Salamone, F., Scamoni, F., & Scrosati, C. (2019). A review of performance of zero energy buildings and energy efficiency solutions. Journal of Building Engineering, 25, 100772. https://doi.org/https://doi.org/10.1016/j.jobe.2019.100772
Bethell, J., Aelick, K., Babineau, J., Bretzlaff, M., Edwards, C., Gibson, J.-L., Hewitt Colborne, D., Iaboni, A., Lender, D., Schon, D., & McGilton, K. S. (2021). Social Connection in Long-Term Care Homes: A Scoping Review of Published Research on the Mental Health Impacts and Potential Strategies During COVID-19. Journal of the American Medical Directors Association, 22(2), 228-237.e225. https://doi.org/https://doi.org/10.1016/j.jamda.2020.11.025
Blundell-Jones, P. (2011). Reframing Park Hill [Sheffield, England] [journal article]. Architectural review(1376), 83-93.
https://search.ebscohost.com/login.aspx?direct=true&AuthType=sso&db=bvh&AN=6 69491&site=ehost-live&custid=s2775460
Carbon Leadership Forum. (2023). Understanding Embodied Carbon. Retrieved 15 October from https://carbonleadershipforum.org/embodied-carbon101/#:~:text=The%20majority%20of%20a%20building's,after%20the%20building%2 0is%20constructed.
Commission, V. C. a. E. (2008). A State of Liveability: An Inquiry into Enhancing Victoria's Liveability. Commonwealth of Australia. (2023). Residential buildings. Retrieved 11 October from https://www.energy.gov.au/government-priorities/buildings/residential-buildings
Commonwealth of Australia and the States and Territories of Australia. (2022). NatHERS Heating and Cooling Load Limits Standard. In. Canberra: Australian Building Codes Board.
Crawford, K., Johnson, C., Davies, F., Joo, S., & Bell, S. (2014). Demolition or Refurbishment of Social Housing?: A review of the evidence.
Crawford, R., & Stephan, A. (2021). The effect of data age on the assessment of a building's embodied energy.
Densley Tingley, D., Giesekam, J., & Cooper-Searle, S. (2018). Applying Circular Economic Principles to Reduce Embodied Carbon. In F. Pomponi, C. De Wolf, & A. Moncaster (Eds.), Embodied Carbon in Buildings: Measurement, Management, and Mitigation (pp. 265-285). Springer International Publishing. https://doi.org/10.1007/978-3-31972796-7_12
Dunlop, A. (2019, 7 November 2019). Getting warmer: Collective Architecture upgrades Glasgow tower blocks. Retrieved 8 August from https://www.architectsjournal.co.uk/buildings/getting-warmer-collective-architectureupgrades-glasgow-tower-blocks
ERA Architects. (Unknown). Ken Soble Tower. Retrieved 8 August from https://www.eraarch.ca/projects/ken-soble-towertransformation/#:~:text=One%20of%20the%20first%20of,retrofit%20project%20in% 20the%20world
F. Mendoza, J. M., Gallego Schmid, A., & Azapagic, A. (2019). Building a business case for implementation of circular economy in higher education institutions. Journal of Cleaner Production, 220, 553-567. https://doi.org/10.1016/j.jclepro.2019.02.045
Floyd, W. (2007). Building thermal performance report: Office of Housing Tower, Gordon Street, Footscray
Gandh, V., & North, M. L. (2019). Sirius: Retrofitting Brutalism in Sydney. IOP Conference Series: Earth and Environmental Science, 290(1), 012153. https://doi.org/10.1088/1755-1315/290/1/012153
Giesekam, J., Barrett, J., Taylor, P., & Owen, A. (2014). The greenhouse gas emissions and mitigation options for materials used in UK construction. Energy and Buildings, 78, 202-214. https://doi.org/https://doi.org/10.1016/j.enbuild.2014.04.035
Haddad, S., Paolini, R., Synnefa, A., De Torres, L., Prasad, D., & Santamouris, M. (2022). Integrated assessment of the extreme climatic conditions, thermal performance, vulnerability, and well-being in low-income housing in the subtropical climate of Australia [Article]. Energy and Buildings, 272, Article 112349. https://doi.org/10.1016/j.enbuild.2022.112349
Intergovernmental Panel on Climate Change. (2022). Mitigation Pathways Compatible with 1.5°C in the Context of Sustainable Development. In Global Warming of 1.5°C: IPCC Special Report on Impacts of Global Warming of 1.5°C above Pre-industrial Levels in Context of Strengthening Response to Climate Change, Sustainable Development, and Efforts to Eradicate Poverty (pp. 93-174). Cambridge University Press.
https://doi.org/DOI: 10.1017/9781009157940.004
Jara-Baeza, F., Rajagopalan, P., & Andamon, M. M. (2023). A holistic assessment of indoor environmental quality perception in Australian high-rise social housing [Article].
Energy and Buildings, 284, Article 112859. https://doi.org/10.1016/j.enbuild.2023.112859
Lacaton & Vassal. (2021). Rothwell Chair Symposium. Living in the city: exemplary social and affordable housing design, Sydney.
Lalande, C., Emma-Liisa, H., Herda, G., Garrigan, C., Halcomb, J., & Bonetti, M. (2015). Green Building Interventions for Social Housing. UN-Habitat. https://unhabitat.org/green-building-interventions-for-social-housing
Mark, L. (2023). Park Hill, phase 2 : Mikhail Riches' second phase in the restoration of Sheffield's Park Hill grapples with the constructional complexities of Europe's largest listed building [journal article]. Architectural review(1499), 68-76. https://search.ebscohost.com/login.aspx?direct=true&AuthType=sso&db=bvh&AN=8 55868&site=ehost-live&custid=s2775460
McNeils, S., & Reynolds, A. (2001). Creating better futures for residents of high-rise public housing in Melbourne.
Nationwide House Energy Rating Scheme. (2013, March 2013). Climate Zone Map Retrieved 14 October from https://www.nathers.gov.au/themes/custom/govcms8_uikit_starter/climatemap/index.html
Onyszkiewicz, J., & Sadowski, K. (2022). Proposals for the revitalization of prefabricated building facades in terms of the principles of sustainable development and social participation. Journal of Building Engineering, 46, 103713. https://doi.org/https://doi.org/10.1016/j.jobe.2021.103713
Ozarisoy, B., & Altan, H. (2021). A novel methodological framework for the optimisation of post-war social housing developments in the South-eastern Mediterranean climate: Policy design and life-cycle cost impact analysis of retrofitting strategies [Article]. Solar Energy, 225, 517-560. https://doi.org/10.1016/j.solener.2021.07.008
Porter, L., Kelly, D., Kunjan, P., Levin, I., Shaw, K., & Davies, L. (2023). Victoria’s Housing Statement: A critical explainer. https://cur.org.au/cms/wpcontent/uploads/2023/09/vic-housing-statement-explainer.pdf
Reap, J., Roman, F., Duncan, S., & Bras, B. (2008). A survey of unresolved problems in life cycle assessment. The International Journal of Life Cycle Assessment, 13(5), 374-388. https://doi.org/10.1007/s11367-008-0009-9
Rohde, L., Larsen, T., Jensen, R., & Larsen, O. (2019). Framing holistic indoor environment: Definitions of comfort, health and well-being. Indoor and Built Environment, 1420326X1987579. https://doi.org/10.1177/1420326X19875795
Rydin, Y., Bleahu, A., Davies, M., Dávila, J. D., Friel, S., De Grandis, G., Groce, N., Hallal, P. C., Hamilton, I., Howden-Chapman, P., Lai, K. M., Lim, C. J., Martins, J., Osrin, D., Ridley, I., Scott, I., Taylor, M., Wilkinson, P., & Wilson, J. (2012). Shaping cities for health: complexity and the planning of urban environments in the 21st century. Lancet, 379(9831), 2079-2108. https://doi.org/10.1016/s0140-6736(12)60435-8
Shrubsole, C., Hamilton, I. G., Zimmermann, N., Papachristos, G., Broyd, T., Burman, E., Mumovic, D., Zhu, Y., Lin, B., & Davies, M. (2018). Bridging the gap: The need for a systems thinking approach in understanding and addressing energy and environmental performance in buildings. Indoor and Built Environment, 28(1), 100-117. https://doi.org/10.1177/1420326X17753513
Sustainability Victoria. (2019). Comprehensive Energy Efficiency Retrofits to Existing Victorian Houses https://assets.sustainability.vic.gov.au/susvic/Report-EnergyComprehensive-Energy-Efficiency-Retrofits-to-Existing-Victorian-Houses-PDF.pdf
Tennakoon, M., & Kulatunga, U. (2019). Understanding liveability: related concepts and definitions. https://doi.org/10.31705/WCS.2019.57
United Nations. (2016). Sustainable Development Goals. Retrieved 11 October from https://www.un.org/sustainabledevelopment/
Vakalis, D., Diaz Lozano Patino, E., Opher, T., Touchie, M. F., Burrows, K., MacLean, H. L., & Siegel, J. A. (2021). Quantifying thermal comfort and carbon savings from energyretrofits in social housing. Energy and Buildings, 241, 110950. https://doi.org/https://doi.org/10.1016/j.enbuild.2021.110950
Vella, K. (1990). The high rise at a glance : a summary paper profiling Ministry of Housing and Construction high rise accommodation. In V. M. o. H. a. Construction (Ed.).
Victorian Government. (2023). Victoria's Housing Statement. https://www.vic.gov.au/sites/default/files/202309/DTP0424_Housing_Statement_v6_FA_WEB.pdf
Willand, N., Maller, C., & Ridley, I. (2019). Addressing health and equity in residential low carbon transitions – Insights from a pragmatic retrofit evaluation in Australia [Article]. Energy Research and Social Science, 53, 68-84. https://doi.org/10.1016/j.erss.2019.02.017
World Green Building Council. (2019). Advancing Net Zero. Retrieved 29 August from https://worldgbc.org/advancing-net-zero/embodied-carbon/
Yoon, Y. B., Seo, B., Koh, B. B., & Cho, S. (2019). Performance analysis of a double-skin façade system installed at different floor levels of high-rise apartment building. Journal of Building Engineering, 26, 100900. https://doi.org/https://doi.org/10.1016/j.jobe.2019.100900
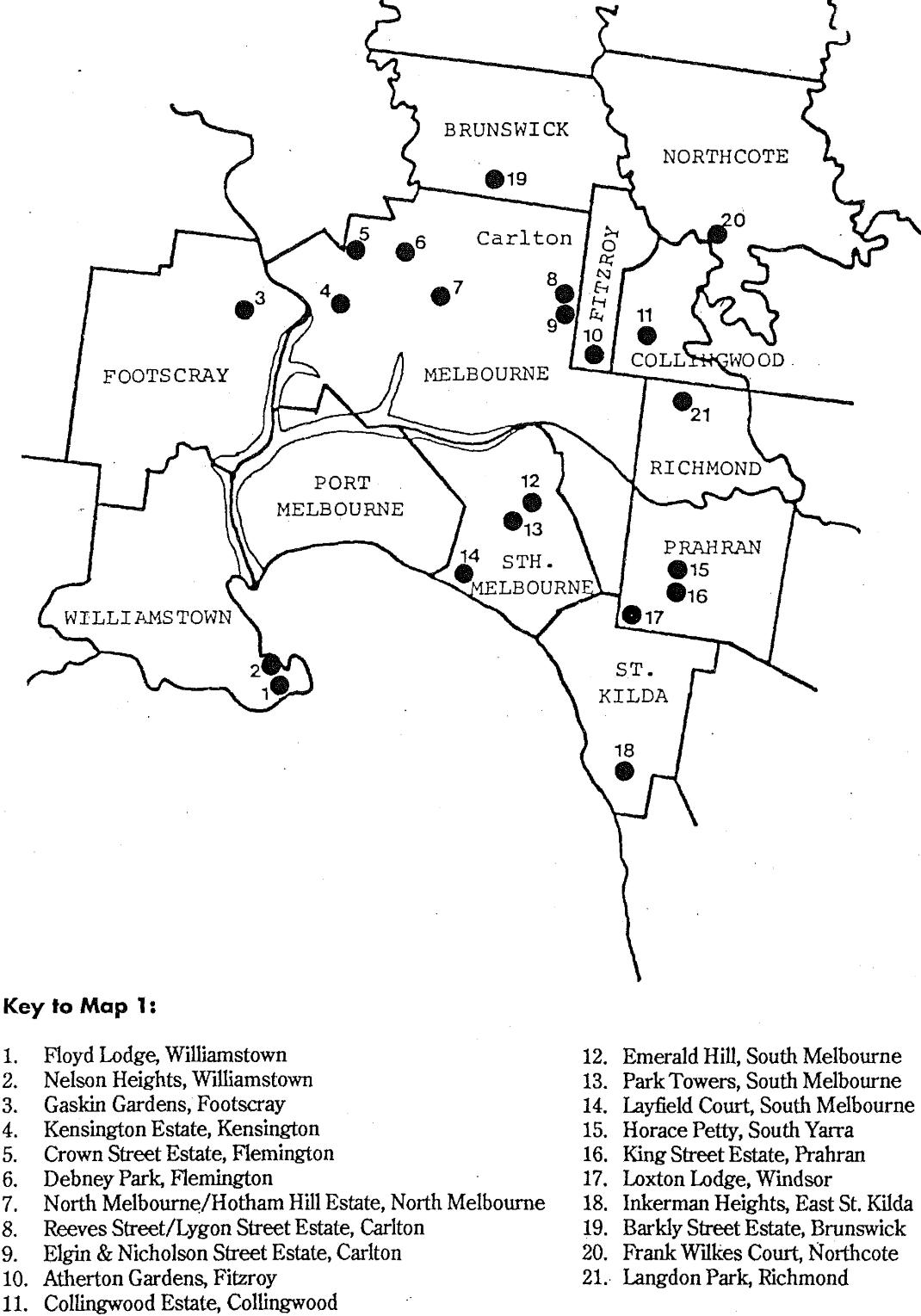
Table 12 Inventory of Melbourne’s 44 high-rise social housing buildings (Vella, 1990).
Image Name Location Architect(s) Social criteria Score Justification
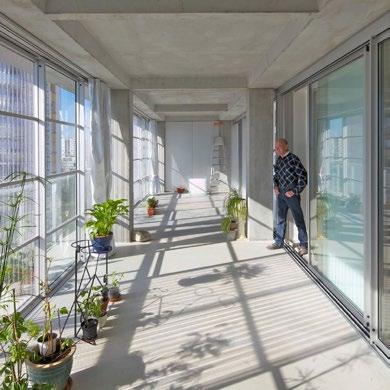
Cité du Grand Parc Bordeaux, France Christophe Hutin architecture, Frédéric Druot, and Lacaton & Vassal
Fresh air access
5 Open-air balconies and provision of operable sliding doors increases resident's access to fresh air.
Window control access
Natural light access
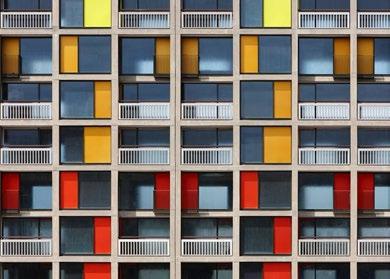
Park Hill, Phase 1 Sheffield, UK Hawkins\ Brown and Studio Egret West
Pleasant views access
Fresh air access
5 Residents have total window (sliding door) control.
5 Replacing existing bay windows, sills and external walls with floor-to-ceiling glazing maximises access to natural light.
5 As above.
3 Windows are ventilated via side panels to keep their design simple, increasing fresh air access without the need to open a window.
Window control access
Natural light access
2 Windows are inoperable. There is some window (sliding door) access which can be controlled by residents.
4 The proportion of glazing was enlarged from one-third to twothirds.
criteria
Embodied carbon
Energy demand
2 Materials used in apartment retrofit include concrete, steel, transparent polycarbonate sheeting and aluminium doubleglazed glass sliding doors. All these materials have high embodied carbon emissions. However, most of the existing apartment structure was retained during the retrofit.
4 Significant improvements to energy performance recorded through addition of winter gardens, new glazing and thermal curtains. Heating consumption cut by 60%. Winter gardens have a double benefit as liveable space and thermal insulator.
Embodied carbon 1 The building was stripped back to its structural frame, resulting in significant embodied carbon emissions to replace the existing infill (precast concrete, brick and glass). New materials used include glass, concrete, steel, and anodised aluminium panelsmaterials which have high embodied carbon emissions.
(Lacaton & Vassal, 2021)
Energy demand 3 New external skins comply with required energy performance standards.
(BlundellJones, 2011; Gandh & North, 2019)
Pleasant views access
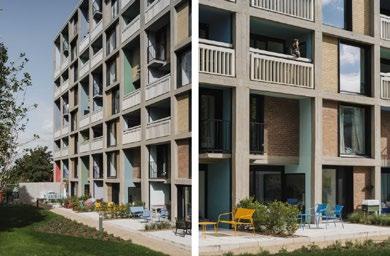
Park Hill, Phase
2 Sheffield, UK Mikhail Riches Fresh air access
Window control access
4 Floor to ceiling glazing provides access to pleasant views for residents. Views are somewhat obstructed by the anodised aluminium panels.
3 Fresh air only accessible through operable sliding doors. Not available through unoperable windows.
3 Window control through provision of sliding doors in living spaces and spaces with access to balconies. All other windows are fixed and nonoperable.
Natural light access
Pleasant views access
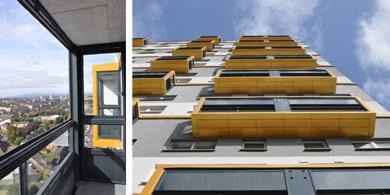
Cedar Court Glasgow, Scotland Collective Architecture Fresh air access
Window control access
Natural light access
3 No significant improvement to natural light access (openings remained same size).
3 No significant improvement to pleasant views access (openings remained same size).
4 Sufficient fresh air access through provision of operable windows.
5 All windows are operable giving residents full access.
3 Sufficient natural light access. Openings remain the same size after retrofit.
Embodied carbon
3 Majority of existing structure retained. Embodied carbon emissions associated with demolition and rebuild of apartment entrance areas, some internal walls, and insulation. New steel framing system, concrete railings, insulation, glazing and entry ways.
(Mark, 2023)
Energy demand
4 Thermal improvements made by adding insulation to inside of apartments - including cavity wall brick panels, balcony flank walls and soffits. Windows moved back into thermal line. Predicted operational energy improvement of 87% supporting transition from gas to electric heating.
Embodied carbon 3 Retention of existing building deemed positive. New windows, external insulation, HVAC systems, and winter gardens (enclosed existing balconies).
Energy demand
4 80% reduction in heating demand.
(Dunlop, 2019)
Pleasant views access 4 Access to pleasant views through addition of winter garden which provide more liveable space and transparency with the outside.
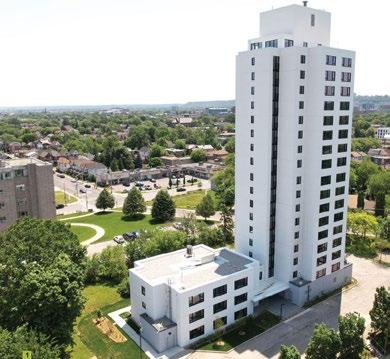
Ken Soble Tower Hamilton, Canada ERA Architects Fresh air access
5 Individual suite ventilations ensures fresh air delivery under Passive House certification.
3 Limited window control due to Passive House requirements. Natural light access 3 Some improved natural light access through the provision of floor to ceiling windows.
Embodied carbon 3 Majority of existing structure retained. Retrofit included addition of insulating building envelope, installation of Passive House certified windows, systems upgrade and internal renovation.
(ERA Architects, Unknown) Window control access
Energy demand 5 Operational carbon emissions reduced by 94%. Apartments meet Passive House energy performance standards.
Pleasant views access 3 Some improved pleasant view access through the provision of floor to ceiling windows.