
10 minute read
Chemistry) Behind Fireworks:
Alexia Noirot UVI-5
Fireworks are one of those things that people see and think ‘oh that's so cool!’ but have you ever wondered about how they work?
Advertisement
The source of most fireworks is a small tube called an aerial shell that contains explosive chemicals. All the lights, colours, and sounds of a firework come from these chemicals. The aerial shell is made of gunpowder and small bits of explosive compounds, known as stars, are contained around them. Stars are round pellets ranging in size from a pea to a golf ball and they are responsible for the colour of the firework. There are six main ‘ingredients’ for a successful firework:
● A fuel source
● An oxidising agent
● A reducing agent
● A regulator
● A colouring chemical
● A binding agent
I will talk about each of these separately to describe their purpose in the fireworks.
A superfluid fountain:
The Fuel source:
The most common fuel source for fireworks is gunpowder. It consists of potassium nitrate (75% by weight), charcoal (15% by weight), and sulphur (10% by weight). Charcoal is just a compound of carbon.
Oxidising Agent:
Firstly, the oxidising agents. The three main oxidising agents used in fireworks are: nitrates, chlorates and perchlorates. In the name ‘oxidising agent’ one can assume that their main purpose is to provide oxygen for another chemical or reaction. We know that oxidising agents are the chemical which is reduced (reduction is the gain of electrons), and so they help the other chemical become oxidised (lose electrons by taking electrons from them.
Each oxidising agent has its respective redox equation:
(X denotes the ion the oxidsing agent is bonded too, this is general and not specific to the compound)
Nitrates:
XNO3 —> XNO2 + ½ O2
Chlorates:
2XClO3 —-> 2XC l + 3O2
Perchlorates:
XClO4 —-> XCl + 2O2 nitrate compound used. Avogadro’s Constant (6.02 x 10^ 23) is used to define the number of compounds/molecules in one mole. Chlorates are the most effective oxidising agent in firework manufacturing. The reason being: Chlorates retain the perfect amount of oxygen at the point of explosion, which creates the most impressive combustion. Alternatively, perchlorates retain even more oxygen, but have improved chemical stability – making them less likely to explode. Nitrates use only one-third of their oxygen potential, resulting in an underwhelming firework explosion.
Reducing agents:
Now that we’ve talked about oxidising agents, it only seems right to talk about their partners: the reducing agents.
The most common reducing agents are sulphur and charcoal (compound of carbon) When ignited, these materials react with oxygen (produced from the oxidising agent) to create sulphur dioxide and carbon dioxide, respectively These gases fuel the firework through its path of travel, prior to explosion.
S + O2 —--> SO2
C + O2 —--> CO2
Chlorates are the most effective oxidising agent, they produce 3 moles of oxygen for every two moles of the chlorate compound. Alternatively, nitrates are the worst oxidising agent, producing only half a mole of oxygen for every mole of the
The two equations can also tell us the standard enthalpy change of combustion for carbon and sulphur. Standard enthalpy change of combustion is defined as ‘the enthalpy change when one mole of a compound is completely combusted in oxygen under standard conditions (298 degrees Kelvin and 100KPa of pressure) As we know, combustion is an exothermic reaction so heat energy is given out by the system.
ΔH= 394 kJ mol 1 for carbon
ΔH=-297kJ mol-1 for sulphur faster. One would change this depending on how high up in the sky the firework is designed to explode.
The reaction of carbon is more exothermic than sulphur, therefore more heat energy is released during the combustion of carbon.
The Colouring Chemical: (arguably the best bit of this article and the only physics-y bit)
The part everyone has been waiting for: how do fireworks get their colour? To put it simply, the answer lies in the flame tests we all learnt in GCSE chemistry But to explain how these flame colours arise, a little bit of particle physics is required.
Regulator:
Regulators are often made from various metal powders and are essential to firework safety As the technical term "regulator" suggests, by adding small amounts of metal to the firework mix, the speed of the explosive reaction can be regulated, the inclusion of metal creates a slower burn and thus, a delayed explosion. Regulators make all the difference in fireworks as one doesn't want the explosion to occur by the ground but rather when the shell is at the top of its trajectory The regulator acts as a ‘stopper’ and prevents the actual explosive part from touching until it has all reacted. The size of the metal chunks can alter the rate of explosion. By using larger chunks, it takes longer for all the regulators to react and the path to essentially be ‘clear’ for the actual firework part to combust. In contrast, using smaller chunks/powdered metal results in the rate of ration of the regulator is faster and so the combustion of the firework is
Electrons are held in quantum shells around the nucleus of an atom. We refer to the first shell as the ‘ground state’ of n=1, the following shells are denoted n=2, n=3 and so on. When electrons are bombarded with energy (from for eg a flame) they absorb the energy and become excited. When excited, electrons jump to a higher energy level. Once at the higher level, they become de-excited as the electron wants to return to the ground state as it is more energetically stable. Upon falling, the electron re-emits the energy that it previously absorbed. This energy leaves the atom as light (or a photon). Light can be modelled as a particle with each particle of light (photon) holding energy (E) equal to Planck’s constant
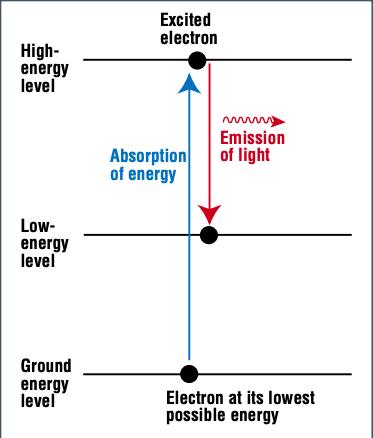
(h) times frequency (f). We can write this in the equation E=hf. From gcse waves, we know that the speed of any wave ( c) is equal to the frequence times the wavelength (Λ): c=fΛ, by rearranging for f and substituting back into the first formula, we get: E=hc/Λ. So each photon of light contains this amount of energy When the electron absorbs this, it rises to a higher state. And as it falls back down, it releases that amount of energy. The colours we see are related to the wavelength of the photon emitted. From the electromagnetic spectrum (EM spectrum) we can decipher what colour the photon will be: red having a wavelength of approx 650 nm, and blue having a wavelength of approx 350 nm. The rest of the colours fall in between. But how come different elements give different colours? The spacing between quantum shells is specific to the element in question. The different spacing of energy levels means that a different amount of energy will be released per element And so, different elements produce different colours when their electrons are excited.
The Binding Agent: the composition together. Dextrin is a water-soluble binder.
How the explosion happens:
Now that we know how all the little bits work inside the firework, it’s time to put them together and find out how the whole thing goes from a shell in the ground to colours in the sky. The explosion of a firework happens in two steps: The aerial shell is shot into the air, and then it explodes in the air, many feet above the ground. To propel the aerial shell into the air, the shell is placed inside a tube, called a mortar, which is often partially buried in sand or dirt A lifting charge of gunpowder is present below the shell with a fuse attached to it. When this fuse, called a fast-acting fuse, is ignited with a flame or a spark, the gunpowder explodes, creating lots of heat and gas that cause a buildup of pressure beneath the shell. Then, when the pressure is great enough, the shell shoots up into the sky
After a few seconds, when the aerial shell is high above the ground, another fuse inside the aerial shell, called a time-delay fuse, ignites, causing the bursting charge to explode. This, in turn, ignites the black powder and the stars, which rapidly produce lots of gas and heat, causing the shell to burst open, propelling the stars in every direction.
Binders are used to hold what is essentially the mixture of the firework together in a paste like mixture. The most commonly used binder is known as dextrin, a type of starch which holds
During the explosion, not only are the gases produced quickly, but they are also hot, and they expand rapidly, according to Charles’ Law (v1/t1 = v2/t2), which states that as the temperature of enclosed gas increases, the volume increases, if the pressure is constant The loud boom that accompanies fireworks is actually a sonic boom produced by the expansion of the gases at a rate faster than the speed of sound! If the stars are arranged randomly in the aerial shell, they will spread evenly in the sky after the shell explodes. But if the stars are packed carefully in predetermined patterns, then the firework has a specific shape.
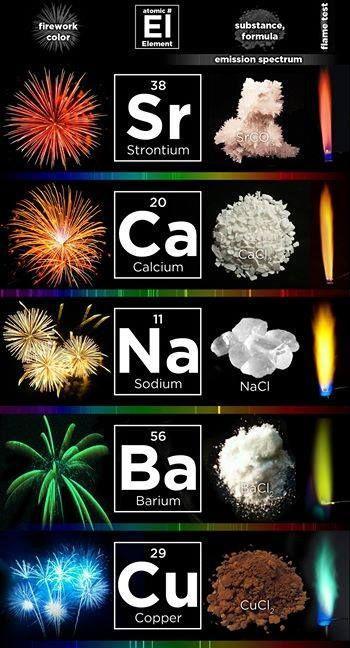
What is the Shape of the Universe?
Lexi Diotallevi LVI-2
The mental picture of the universe many of us have is an infinite expanse with no shape, but is this a true perspective?
Our universe is smooth and homogeneousin other words, everywhere in the universe will have a similar average density of luminous matter and will look the same at any instant in time - because of this, the variety of possible shapes the universe can hold will be limited There are two main theories for the shape of the universe: either that it is flat or spherical.
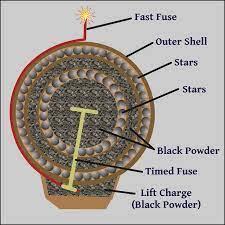
Euclidean space is a 2D or 3D space in which the rules of Euclidean geometry apply, for example angles in a triangle sum to 180° and all right angles are congruent Euclidean space could either be flat (as shown in Fig. 1) or manipulated into other forms, an interesting one being the flat torus shape (Fig. 2). This is a rather special shape as there are some instances where, although the light is travelling in a straight line, it would seemingly be going in a circle around the universe to end up where it started This is because the topology has been distorted when creating the curved structure Theoretically, if you faced one of these directions and there was nothing obstructing the light’s path, the light emitted from behind you would travel around the universe along that straight line until it met your eyes and you would see the back of yourself (Fig 3) You would, however, only be able to notice this phenomenon if the universe was small enough that over the period of time the light has taken to reach your eyes you are able to recognise what it is you are looking at, as by that point the image you are seeing may be from so long ago that the universe looks drastically different now Since spotting copies of ourselves in the universe is imaginably very difficult, scientists have been attempting to find repeating features in the universe by closely analysing the cosmic microwave background radiation (CMBR) left over from the Big Bang.
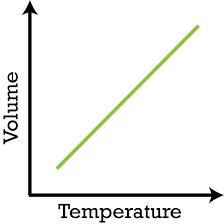
Rather than Euclidean space our universe may instead be spherical We are all familiar with 2-spheres, such as a football or the earth, however, a 3-sphere is an object with 3 dimensions that folds to form the boundary of a sphere in 4D. Life in a 3-sphere is hard to imagine as it would be vastly different from life in flat space. Nevertheless you can try to picture what it would be like by considering yourself to be a 2D being living in a 2-sphere. This sphere would be your entire universe so you wouldn’t be able to access or even see the surrounding 3D space. Just as in a flat universe, the light will travel in straight lines but since you are in a sphere they would actually be circles around the sphere
(Fig 4) Another visual occurrence that would happen to a 2D inhabitant of a
2-sphere would be as follows Imagine yourself at the north pole and someone walking away from you towards the south pole As they approach the equator they will appear to get smaller since your visual circle is expanding and they take up a smaller percentage of it Yet, unusually, as they pass the equator and enter the southern part of the sphere they would appear to get bigger since your visual circle shrinks (Fig. 5). Furthermore, if no one was at the south pole you would see yourself as the light coming off you will travel all the way around the sphere until it returns to you Now, as you may have noticed, we do not see ourselves when we look to the universe nor do we see celestial objects increasing in size as they move away. But, as with the flat torus, just because we don’t see it, that doesn’t mean it can’t exist Perhaps In closing, most evidence indicates a flat universe Despite this, when considering the colossal scale of the universe it could also be easy to conclude that maybe we can only see a very small portion of the universe, which appears to have no curvature, but when knitted together with the multitude of other pieces beyond our view an interesting curved shape is revealed. So perhaps it is more apt to finish by saying that our observable universe is almost undoubtedly flat, but beyond that it is impossible to tell the circumference of the spherical universe is so large that it is greater than the observable universe In a spherical universe the geometry we are so familiar with would be distorted, for example the angles in a triangle would seem to sum to greater than 180° since straight lines would become curved and create a “puffy” triangle For this reason we can test whether the universe is curved or not. By measuring each cold and hot spot in the universe its diameter across and its distance from the earth are known, allowing us to form three sides of a triangle From here the angle the spot subtends from the sky can be measured so that we can see whether the lengths and angles fit a spherical universe or not. The majority of research suggests that the universe is flat, however there are scientists who have contradicted this theory however it is largely thought that their evidence was as a result of statistical flukes
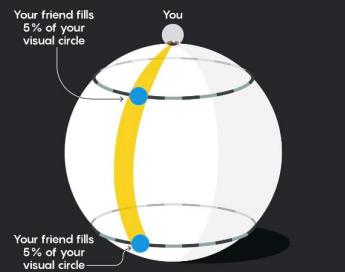
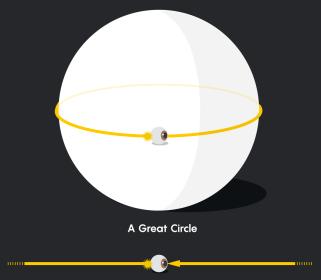
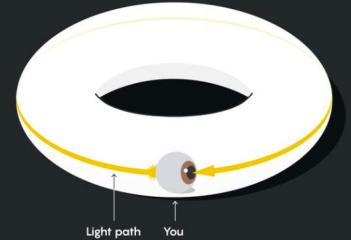
In closing, most evidence indicates a flat universe. Despite this, when considering the colossal scale of the universe it could also be easy to conclude that maybe we can only see a very small portion of the universe, which appears to have no curvature, but when knitted together with the multitude of other pieces beyond our view an interesting curved shape is revealed. So perhaps it is more apt to finish by saying that our observable universe is almost undoubtedly flat, but beyond that it is impossible to tell.