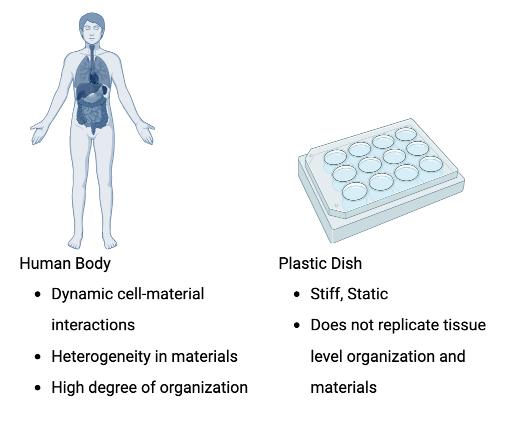
5 minute read
Student Feature: Engineering the Matrix - Soft Materials to Mimic Natural Tissue
Student Feature
Back to Table of Contents
by Adeel Ahmed, Ph.D. Candidate, BMECHE PhD Program, Rochester Institute of Technology
Studying cell biology, drug discovery, and disease progression can appear far removed from the realm of engineering. However, in recent years, innovations in bioengineering have relied heavily on advancements in biomaterials, microfluidics, and engineered tissues.
The stereotypical image of a biomedical lab in movies and TV is that of scientists carrying plastic and glass Petri dishes and reaching to grab a terrified mouse or rabbit for their next experiment. While it is true that plastic and glass dishes are the most widely used substrates to grow cells in most research labs, it does not take much to realize that the stiff, flat plastic dish is not an exact representation of the environment in our body (Figure 1).
Native tissues are highly complex structures comprised of a matrix of protein fibers (micron scale in size), proteoglycans, macromolecules, growth factors, and water (up to 70% of the human body) within which cells are encapsulated (Figure 2). This hydrated macromolecular matrix, referred to as the extracellular matrix (ECM), is the tissue scaffold that allows cells to attach and grow. In addition, the ECM is extremely soft compared to the plastic dishes commonly used – as is evident if you press anywhere on your body compared to the device you are reading this text on. Lastly, the ECM and cells share a dynamic, reciprocal relationship. Cells deposit new ECM material – primarily collagen – during tissue development, and the ECM guides cell functions such as their movement, shape, organization, and gene expression.
Figure 1: Differences between native tissue and cell culture dishes
Figure 2: A cell in its native environment receives guidance from both chemical factors and surrounding materials
Additionally, the ECM is not a random mashup of fibers and
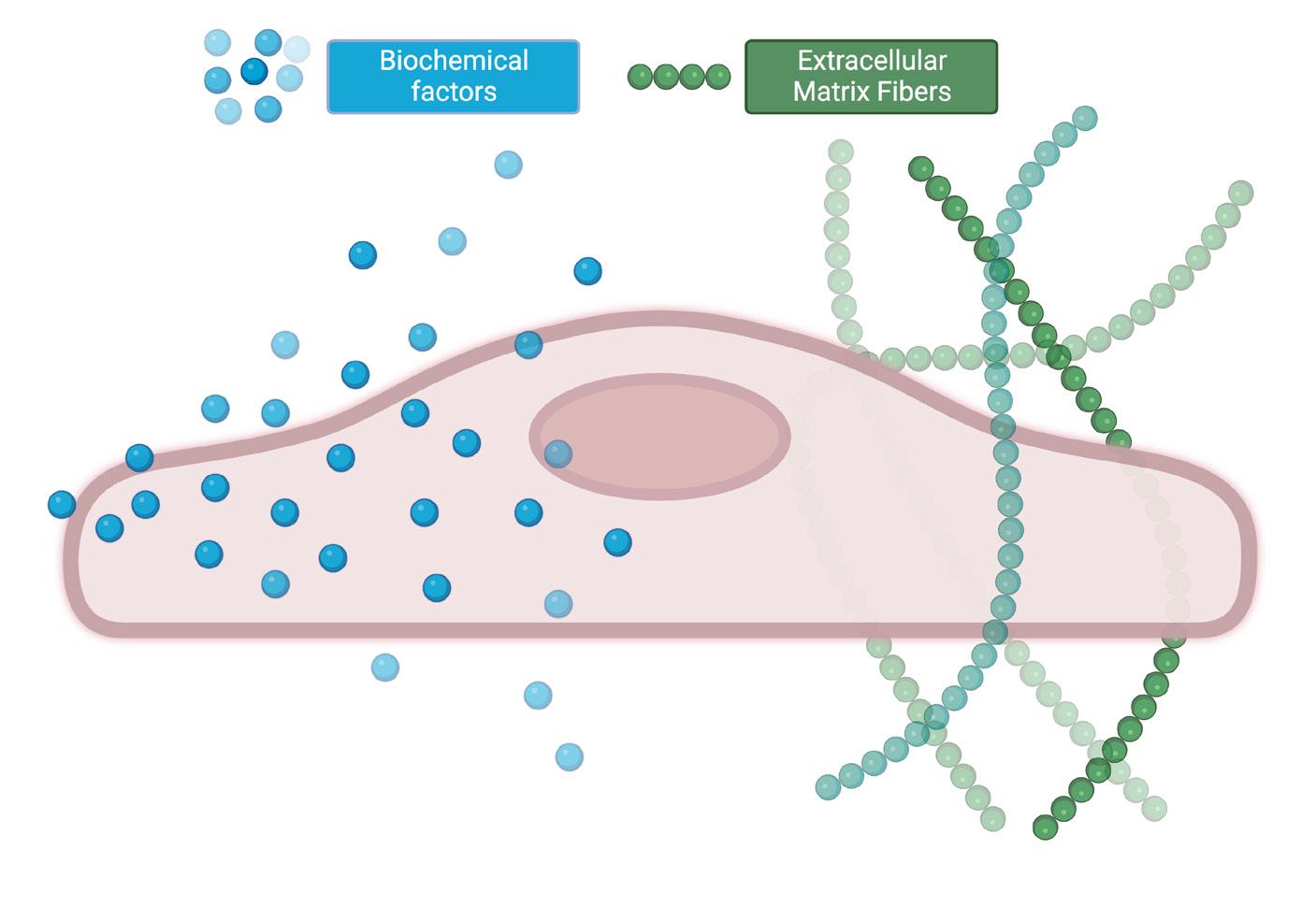
20 | The ROCHESTER ENGINEER SEPTEMBER 2022 student feature
materials. The fibers in tissue are highly organized into various microstructures and represent the tissue function. For example, collagen fibers are highly aligned in load-bearing tissues like muscles and tendons. Around tumors, cells reorganize the ECM and use it as a path to invade the surrounding tissue and blood vessels. In turn, the composition and fiber organization also affect the mechanical properties of the ECM.
These mechanical properties (fiber organization, stiffness, viscoelasticity) are well known to direct stem cell growth and gene expression. Thus, the materials that cells interface with play a critical role in guiding biological processes. Once we identify the rifts in the cell-material interface between native tissue and a petri dish, it is no surprise that most drugs that seem to work on cells in a dish fail to work in animal models. Often, drugs that appear to work in animal models still fail in human trials due to the apparent differences between human and animal physiology.
While the incongruity between the environment of a plastic dish and native tissue has been apparent for the past 50 years, materials scientists and engineers have only recently turned their attention toward novel materials that can precisely mimic the ECM.
Our research at the Biological Microsystems Lab at RIT has been focused on using collagen to recreate the fibrous organization observed in the ECM for studying cell-matrix interactions.
Collagen is the most common material in the ECM. Consequently, it has been the most desirable material to recreate the ECM in vitro because it presents low toxicity, does not elicit an immune response, and cells can dynamically engage with collagen fibers to remodel and reorganize them. Collagen is commercially obtained as a solution in an acidic solution. When this solution is neutralized with a base and warmed to 37˚C (body temperature), the collagen molecules spontaneously come together to form a hydrated fibrous network (hydrogel) and mimic the ECM. However, unlike the native ECM, fibers in this self-assembled hydrogel are randomly organized, as seen in Figure 3.
Figure 3: Difference between a bulk collagen hydrogel (top) and engineered aligned hydrogel (bottom) Continued on page 22...
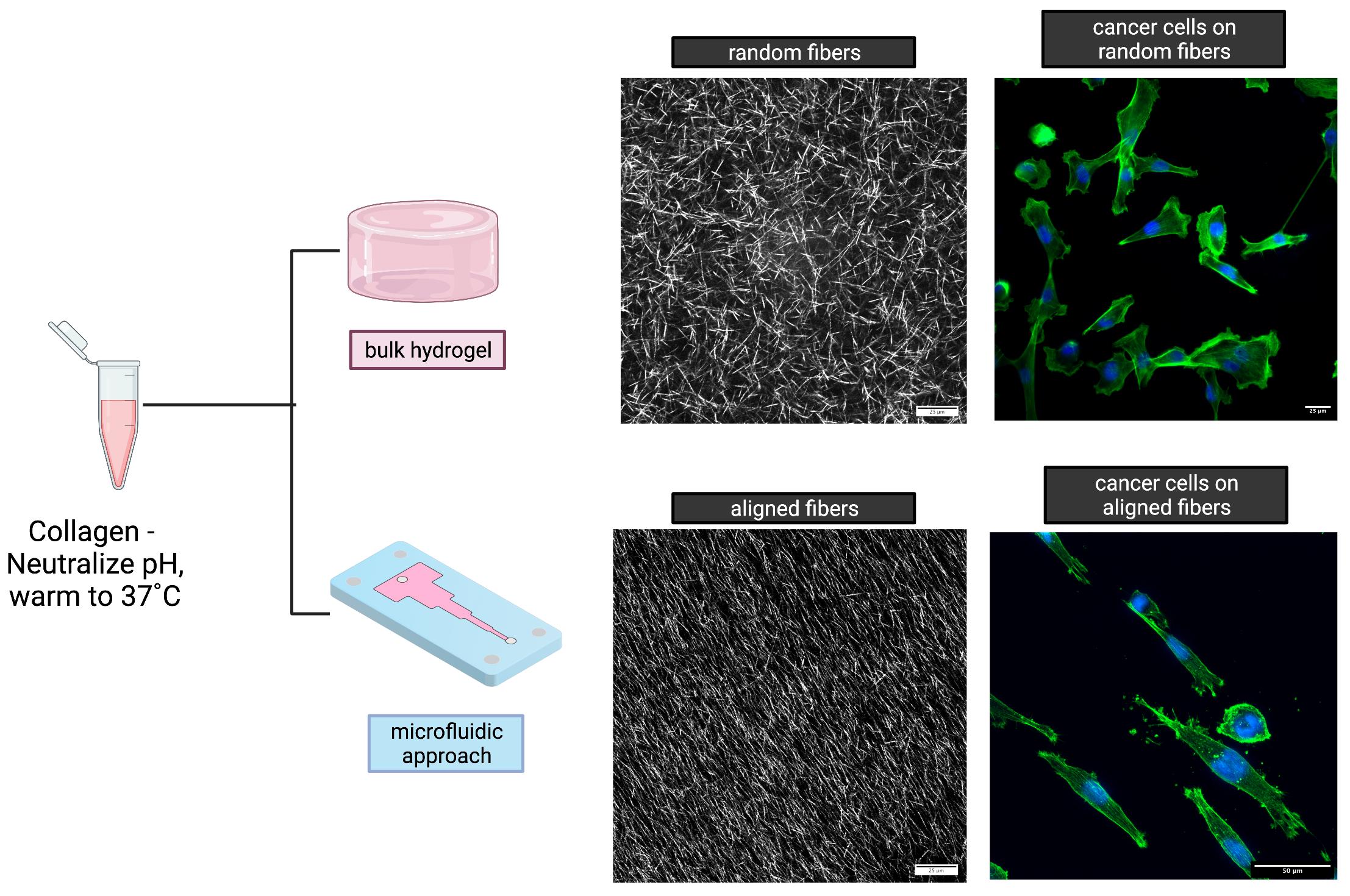
Our work shows that microfluidic systems can be exploited to introduce order into the collagen matrix. We have observed that when a neutralized collagen solution is injected into a microfluidic channel (< 1/100th of an inch) with a constriction in the flow direction, the increased velocity of the collagen solution at the constriction results in an extensional strain component within the viscoelastic collagen solution.
student feature SEPTEMBER 2022 The ROCHESTER ENGINEER | 21
This extensional strain serves to reorient the self-assembling collagen fibers into the flow direction and thus align them.
Using microfluidics and the concept of extensional flows, we have engineered collagen matrices with defined degrees of fiber alignment and directionality (Figure 3). Simply by changing the shape of the microfluidic channel, we can obtain collagen hydrogels with regions of constant fiber alignment, gradients in fiber alignment, and fibers oriented in different directions.
We have further expanded the capacity to engineer the ECM by adding different materials into our collagen to recapture the complexity and heterogeneity of native tissue. Since microfluidic flows are laminar in nature, they prevent the mixing of solutions in them. Thus, distinct materials can be introduced side by side in a microchannel to replicate tissue interfaces or provide unique microenvironments for different cell types. In addition, the mechanical properties and microstructure of the collagen matrix can further be tuned by modifying the self-assembly conditions of the matrix.
When cancer cells were cultured on our engineered collagen, we observed that they organized directionally and followed the collagen fiber alignment, as they do in native tissues. We observed that cells could reorganize the fibers and migrate into the matrix, a behavior that cannot be observed on plastic dishes or many synthetic ECM materials.
We anticipate that our work will be used to provide novel insights into cell behavior in vitro, accurately model tissues, and be scaled up to include several other biomaterials that are found in the ECM (Figure 4). Engineered ECM constructs are expected to improve the relevance of in vitro testing by providing cells with a biologically relevant microenvironment, cutting down on animal testing, and moving us toward personalized medicine. q
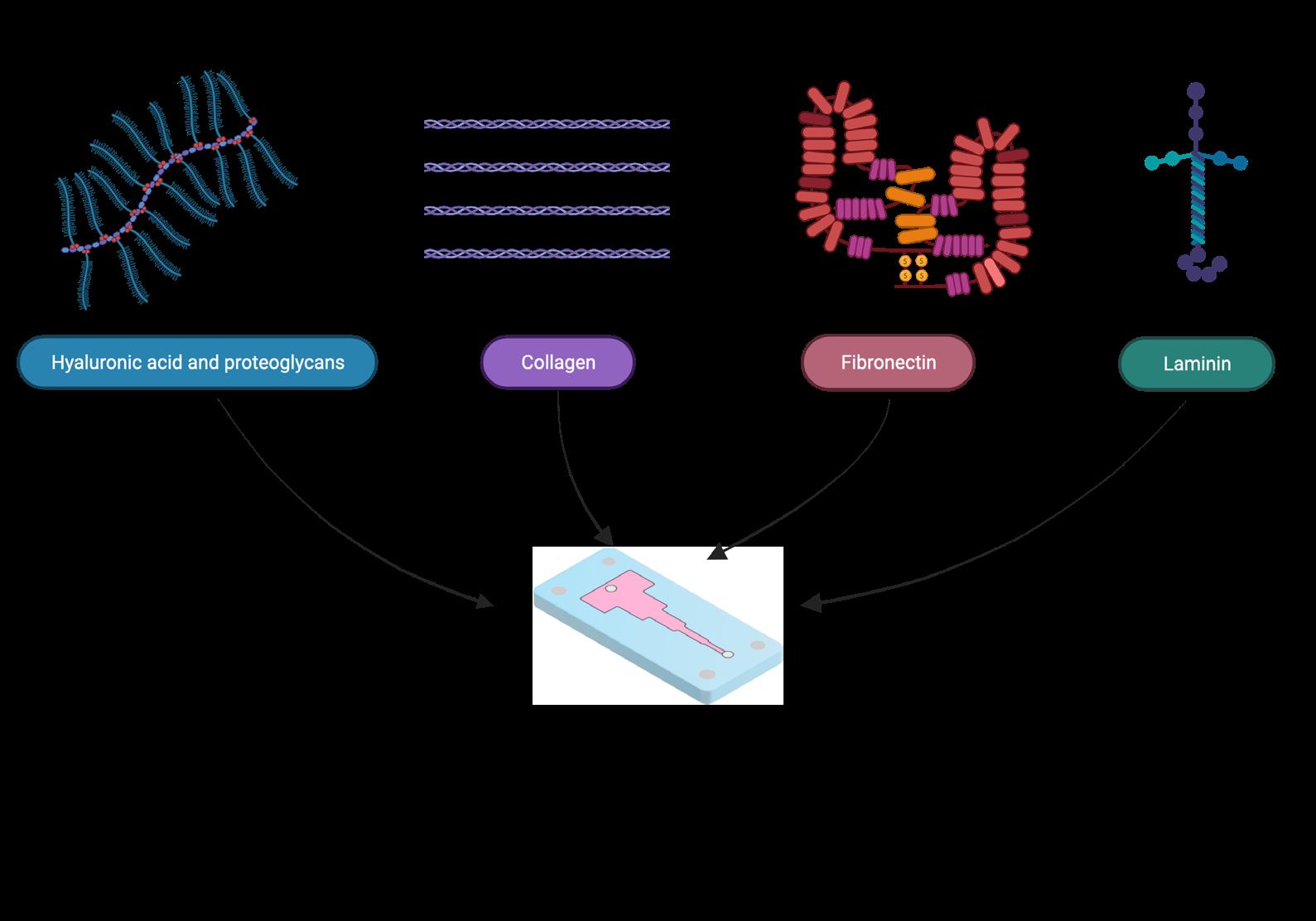
Figure 4: Different ECM materials that can be engineered using microfluidics
22 | The ROCHESTER ENGINEER SEPTEMBER 2022 student feature