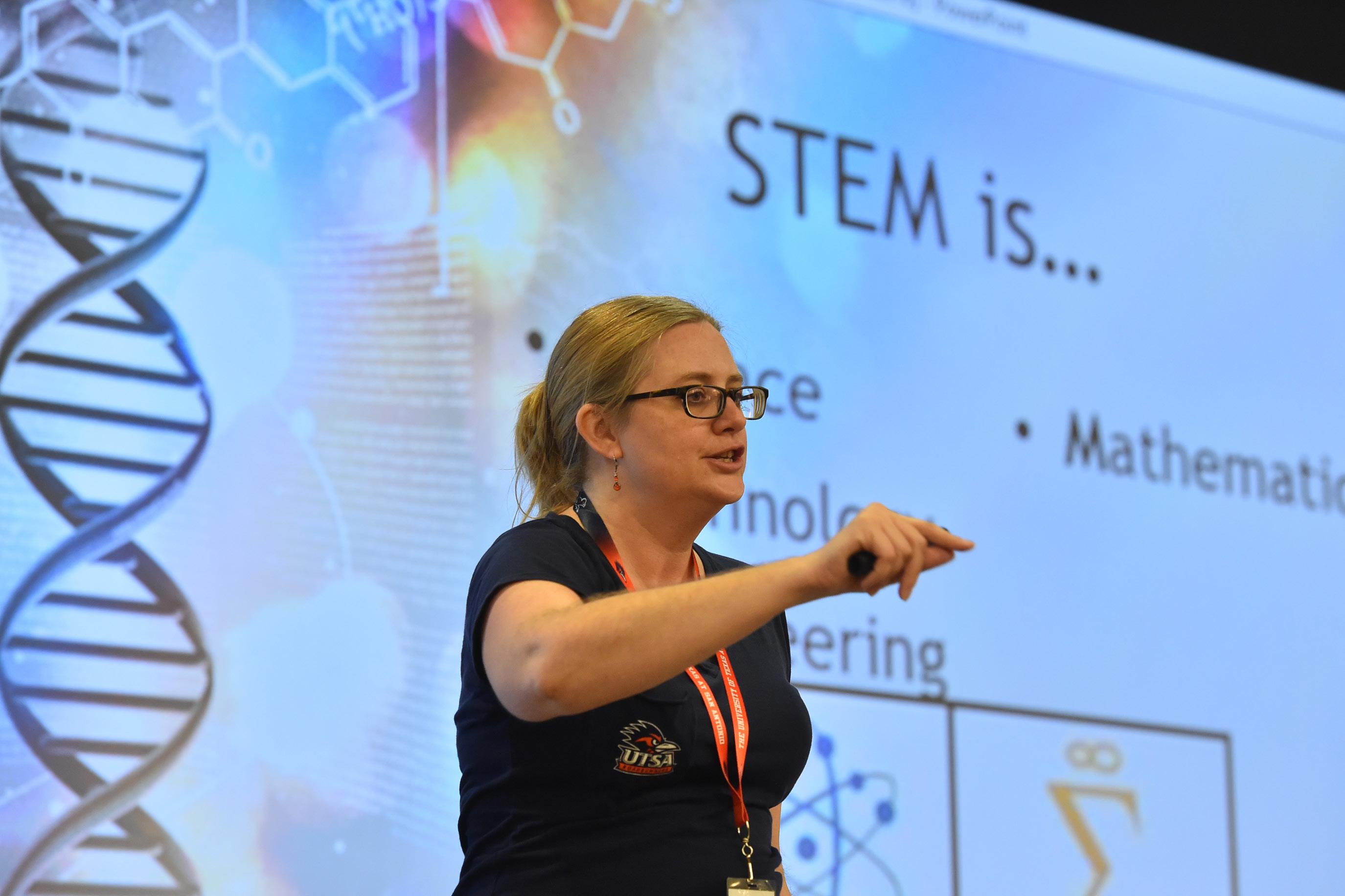
4 minute read
HIGH SCHOOL
Virtually everything just said about middle school applies as well to high school: college and career aspirations ordinarily become more concrete, course selections become more critical, and solid counselling regarding college, career, and courses becomes more necessary.
A strong math curriculum through and beyond algebra is essential if a student is to be prepared for college level STEM study. According to Cliff Adelman in his classic “Toolbox” studies,
Of all the components of curriculum intensity and quality, none has such an obvious and powerful relationship to ultimate completion of degrees as the highest level of mathematics one studies in high school. This is a very critical equity issue because not all high schools can offer their students the opportunity to learn the higher levels of mathematics that propel people to degrees—no matter what their eventual major field of study... And the precise point at which opportunity to learn makes the greatest difference in long-term degree completion occurs at the first step beyondAlgebra 2, whether trigonometry or pre-calculus. 18
Adelman’s follow-up study both confirmed the key role of high school math beyond algebra 2 as dramatically increasing the odds of baccalaureate completion, and also underscored the diminished opportunity African American and Latino students have for such courses: they are significantly less likely than white and Asian students to be attending a high school that offered either calculus, trigonometry or statistics. 19
If high school mathematics beyond algebra 2 correlates so strongly to baccalaureate completion for all majors, it is even more critical in STEM majors, where failing to enter college at least calculus-ready makes timely degree completion much more difficult. And the continuing disparity in high school mathematics opportunity for students of color and low SES continues to limit the pool of potential STEM majors.
Similarly, a strong lab-based science curriculum is essential for college-readiness and maintained STEM interest. Adelman also includes “more than 2.0 Carnegie Units of core laboratory science (biology, chemistry, and physics)” as well as “1.0 or more Carnegie Units of computer science” in the highest level of a scale describing academic intensity. 20
Again, while this intensity of curriculum is important for baccalaureate degree completion in any major, it is clearly more important for STEM majors. And again minority students are less likely to be enrolled in high schools that offer a full range of laboratory sciences.
As outlined below, 21
funding disparities across school districts tend to mean Latino students have more teachers in their first years of teaching and more who are teaching outside their field. This is especially true in STEM courses.
There have been a number of programmatic efforts to address these disparities. The Texas Prefreshman Engineering Program, 22
for example, has a track record since 1979 of providing high school students a rigorous science and math curriculum over the course of their four summers with documented results in college-going and completion rates. While these are not substitutes for systemic change that will enhance STEM opportunity for all students, they represent promising program models to address the immediate crisis in underrepresentation. Such programs illustrate the importance of serious instruction in math, lab science, and computer science; the value of a college setting (and a college faculty) to accustom students to higher education; and the need to track students throughout the school year. They also offer additional opportunities for informal science experiences and for partnerships with other STEM industries.
Advanced Placement and early college programs are likewise valuable to help students become more college-ready. Racial/ ethnic and economic disparities impact the availability of AP courses, but a growing number of low-income school districts are finding higher education partners for early college programs. Early college also provides exposure to higher education faculty, expectations and settings and strongly supports some of the extra-curricular dimensions of college readiness.
Since not every STEM worker will be a research scientist and the field is also in need of a diversified technical workforce as well, high schools should be mindful of educational opportunities in technology, being careful to avoid rigidly tracking students into tech courses just because of demographic factors.
Finally, high schools can also facilitate work experiences that can include formal and informal science visits and field trips, internships, summer or part-time jobs in STEM fields. These opportunities can not only support the aspirations and formation of future scientists and technicians, but engage industry, nonprofit organizations and higher education institutions in on-going collaborations.
Policy Recommendation: Every high school should be equipped to offer a mathematics curriculum beyond Algebra 2, the core laboratory sciences, and computer science, taught by experienced and qualified teachers. Academic and college counseling should also be available to every student. Advanced Placement should be an option in all schools, and early college programs should be fostered.
Practice Recommendation: Precollegiate summer or after-hours programs in STEM fields can be important supplements to high quality STEM teaching and counseling and provide opportunities for partnerships that can ease the transition to higher education and provide realistic STEM career preparation.
18 Clifford Adelman, “Answers in the Toolbox,” 1999, U.S. Department of Education, p. 16.
19 Clifford Adelman, “The Toolbox Revisited,” 2006, U.S. Department of Education, pp. 31-33, https://www2.ed.gov/rschstat/research/pubs/toolboxrevisit/ toolbox.pdf.
20 Ibid., p. xviii and p. 27.