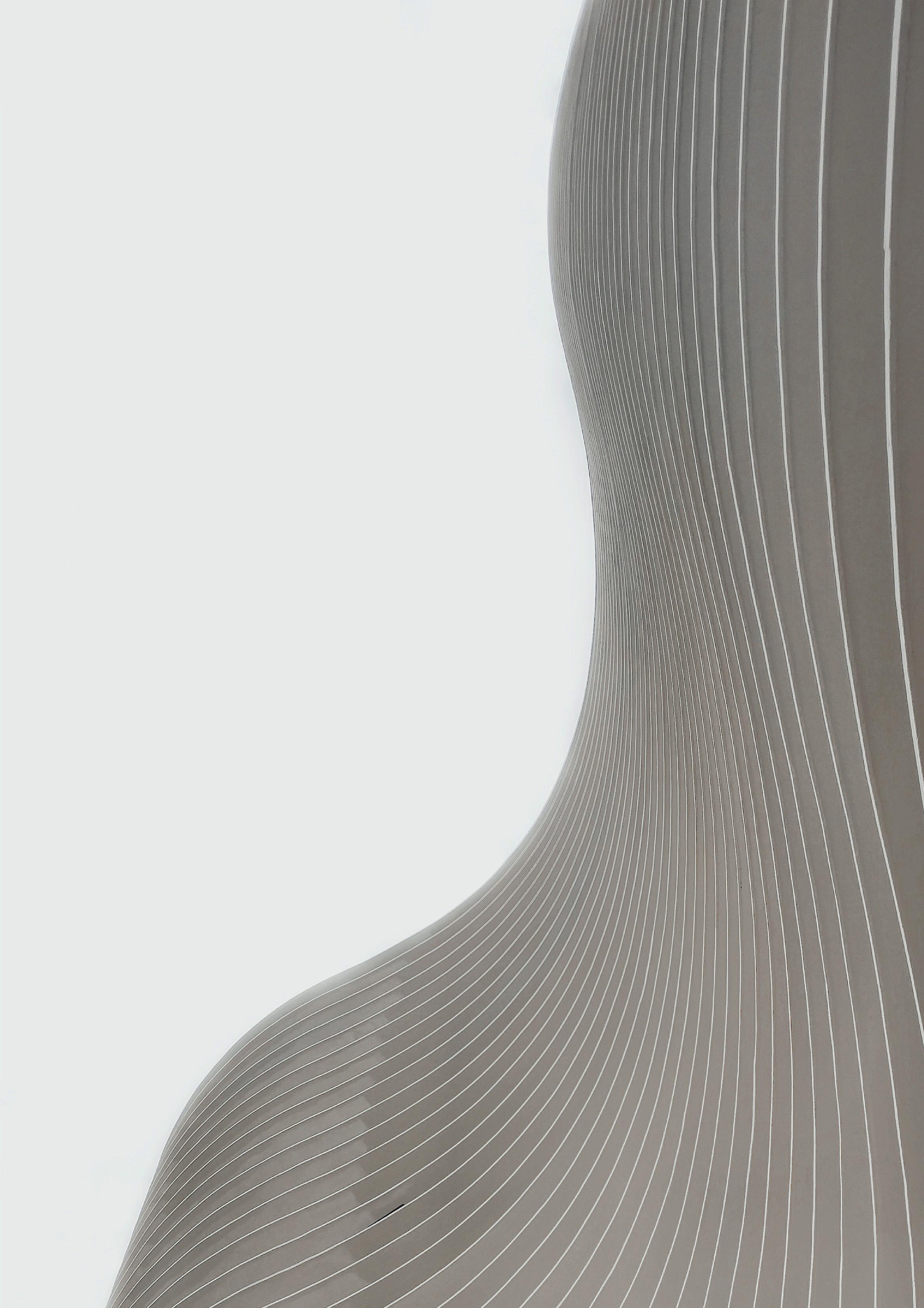
21 minute read
Life Through the ART BUILDING DESIGN
Sustainable
by Kate Xiao
Assessing the environmental impact of buildings
In the contemporary world, buildings are responsible for more than 40% of energy usage and 33% of total greenhouse gas emissions in both developed and developing countries.1 According to the U.K Green Building Council, the construction sector uses more than 400 million tons of materials a year, many of which, such as aluminium, concrete, and steel, have an adverse impact on the environment through their high concentration of embodied carbon content (which refers to the greenhouse gas emissions arising from the manufacturing, transportation, installation, maintenance and disposal of a specific building material), with 9.8 million tons of CO2 produced by 76 million tons of finished concrete in the US.2
Fortunately, this data has not gone unnoticed. In the UK, after the launch of the Green Guide to Specification, Oxford Brookes University and the UK construction industry set out regulations to use certain materials in order to reduce the environmental impact, 230,000 construction projects have improved their environmental standing.2 In the US, the Environmental Protection Agency(EPA) also published a number of rules to reduce negative environmental effects (which includes areas such as soil stabilization, erosion and sediment controls, water contamination and waste management). The EPA also has a Greenhouse Gas Reporting Program (GHGRP), where relevant information is gathered every year from large GHG emission sources and reported to the public every October. This can help organisations and companies keep track of GHG emission states and alter the way of maintenance accordingly.
Before the construction of a building, a life cycle assessment (LCA) is carried out to determine the environmental impact it will bring during its lifetime, from designing to demolishment. A LCA is able to assess energy consumption and environmental impact through a scope of analysis with each type of building and fabrication method and each type of manufacturing or building material. With acknowledgement to different effects building materials and dimension choices will bring, designers can alter their scheme at an early stage and plan to embody the most impact reductions as possible.
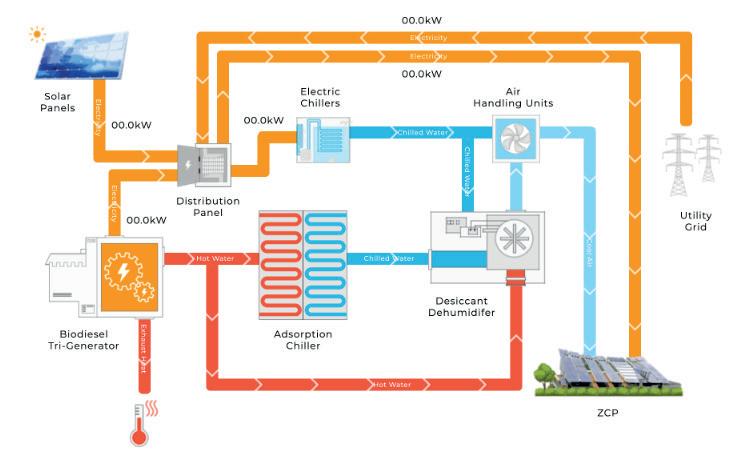
What is a carbon neutral building?
The definition of a carbon neutral building is a building specifically designed to minimise greenhouse gases at all stages. As opposed to the commuter towns left from the Industrial Revolution and rapid urban development in the 21st century, a new trend in urban planning is led by urban projects that aim to reduce the negative impacts on the environment: The construction of sustainable buildings, carbon neutral buildings, eco-neighbourhoods, and more is highly commended on an international basis.
The principles behind carbon neutral buildings
If other buildings aim to reduce its environmental impact to the minimum, carbon neutral buildings aim to achieve net zero carbon emission. All the climate budget from materials manufacturing, transportation, construction process, and building in operation will be counteracted by investing in renewable energy, using timber as carbon storage, engaging in innovative and novel environmentally-friendly technology (examples are listed in case study below). The point is that over the years, net carbon emission remains zero, achieving carbon neutrality.
Case study: Hong Kong
In Hong Kong, the first carbon neutral building—CIC-Zero Carbon Park—was built in 2012.3
Aside from serving as an exhibition, education and information center, CIC-ZCP acts as a test bed for state-of-the-art eco-building design. A native urban woodland in the middle of the compact, densely populated city, 47% of CIC-ZCP land area is covered in greenery, attracting birds and other animals to create a more biodiverse environment.3 The whole building relies on on-site renewable energy generated from photovoltaic panels and a tri-generation system using biofuel.
Active Systems
Modular Integrated Construction – MiC is an innovative construction method whereby free-standing integrated modules are manufactured in a prefabrication factory and then transported on site for installation in a building.4
The emMiC system in CIC-ZCP makes use of a stormwater box culvert as the heat extraction and rejection media of the Air-conditioning system.5
In an air-conditioner, hot air in the room is sucked in by a grill located on the bottom of the indoor unit, which then flows through some pipes through which the refrigerants also flow in (the most common refrigerant gases include HFCs R-410A, CFCs such as R-22 and hydrocarbons such as R-290). The refrigerant fluids absorb the heat from the hot air and become hot gases themselves. After passing through the compressor, the hot gas reaches the condenser in the air-conditioner and condenses to cooled liquid. The stormwater, which is relatively low in temperature (about 20 degrees Celsius), serves as an excellent condensing medium for the air conditioner.
Compared with a typical AC system/electrical heating system, emMiC can reduce energy consumption by 50% and 70% for cooling and heating respectively5 . Using stormwater as a condensing medium also saves fresh water sources, avoids Legionnaire’s disease and mitigates the urban heat island effect*.
*(Urban) Heat island effect: the effect that cause an area of higher temperature relative to surrounding areas, occurs when high concentration of buildings, pavements and other man-made infrastructures absorb and maintain heat radiation.
Air Improvement Photovoltaic (AIPV) Glass Canopy
CIC-ZCP adopts the Cadmium Telluride nano thin-film photovoltaic technology to generate renewable energy from solar power.
Cadmium Telluride (CdTe) solar cells contain thin-film layers of CdTe material as a semiconductor to convert absorbed solar energy into electricity. This type of solar cell is separated into 5 layers: a copper-doped carbon paste cathode (back content), p-type cadmium telluride and n-type cadmium sulfide (CdS) layers in the middle, a tin oxide or cadmium-based stannous oxide transparent layer acting as the anode (front content), and finally glass substrate on the outside.7
8Among all polycrystalline compound semiconductors, CdTe has become a proven TFSC material for its potentiality in several of its advantages and easier methods of thin film depositions:
- An ideal solar cell has a direct band gap of 1.4 eV to absorb the maximum number of photons from the sun’s radiation. CdTe has a near optimum band gap of 1.44 eV, which, compared to the most widely used material – silicon – in the PV industry (indirect band gap of 1.1 eV), is a much more suitable fit in electricity production.8
- CdTe has a high absorption coefficient due to its short absorption length. It absorbs over 90% of accessible photons (hv > 1.44 eV) in a 1 μm thickness.
- The economic cost of producing CdTe cells (associated with polycrystalline and glass) is much lower than the production involving bulk silicon.
- The polycrystalline layers of a CdTe solar cell can be deposited via many different techniques (such as closed-spaced sublimation, physical vapor deposition, RF magnetron sputtering and more).
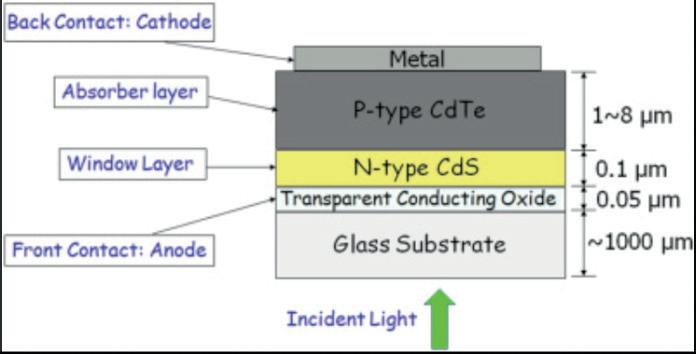
Passive Designs
Cross Ventilated Layout
Cross ventilation is a natural method of cooling the inside of a building —a method that doesn’t require any maintenance cost, carbon emission, or energy consumption, yet still is effective.
The system relies on wind to force external cool air into the building through an inlet (such as a window or wall louver). When this happens, the two sides of the building will be hit with different amounts of pressure. The pressure change will hence force air to the area with lower pressure, which is where the outlet (such as a higher window opening) is located.9 The air circulation allows the inside of the building to experience cool breezes (This is why you will experience breezes when having both the window and room door open).
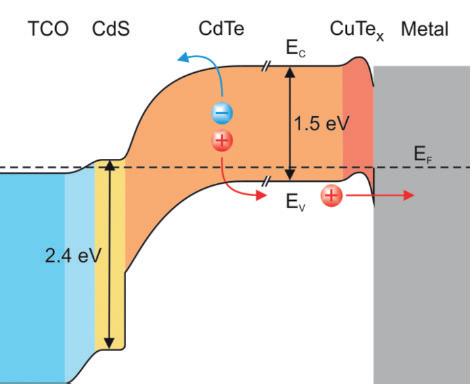
CIC-ZCP locates its main façade to face the southeast to increase efficiency, as the prevailing summer breeze comes from that direction. Compared to a conventional building, the building’s cross ventilated layout is estimated to have reduced the need for air-conditioning by over 34%.3
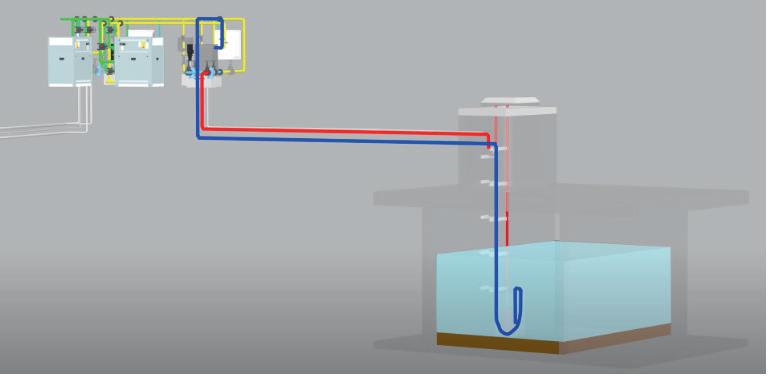
Wind Catcher
A wind catcher is a small chimney fitted on the roof of the building. As the velocity of wind flowing over the roof is greater than the lower windows of the building, air in the shaft is forced down to cool the building. External air from the roof improves the ventilation potential for areas furthest away from the windows and therefore is not touched on by the cross-ventilation system.
Renewable Energy
Renewable energy is a natural energy that will never deplete and can be used again and again. Many practices were taken to increase the use of renewable energy on site, including but not limited to:
- ZCP has its energy generated mainly from biodiesel. Biodiesel is a type of diesel fuel derived directly from plants and animals, or indirectly from agricultural, industrial, domestic, or commercial waste, and consisting of long chain fatty acid esters. The biodiesel tri-generation system on site is estimated to supply over 129% of ZCP’s energy demand.3
· Different areas of the building are assessed to investigate the irradiance level, and 3 types of pho tovoltaic panels are used based on study. Polycrystalline Silicone PV panels, Building Integrated Photovoltaics, and cylindrical CIGS thin film PV panels are integrated to the inclined main roof, viewing deck, and Air-Tree installation respectively. The PV panels are estimated to produce about 57% of ZCP’s energy demand.3
Contribution to community
Reducing carbon emissions in buildings has a crucial role in achieving the Paris climate goals and achieving net zero emission by 2050. An effective, efficient carbon neutral building takes cost-effective technologies in advantage to reduce emissions. It also serves as an lead example to improve health, equity, and economic prosperity in local communities. The action to invest in carbon neutral buildings around the world remains a priority and symbolises an important step taken in achieving sustainable development.
Bibliography https://www.sciencedirect.com/science/article/abs/pii/S0959652615011695 https://gocontractor.com/blog/how-does-construction-impact-the-environment/
1 Peng, Changhai. “Calculation of a building’s life cycle carbon emissions based on Ecotect and building information modeling.” ScienceDirect, 20 Jan. 2016, www.sciencedirect.com/science/ article/abs/pii/S0959652615011695.
2 Sikra, Sonya. “How Does Construction Impact the Environment?” Gocontractor, 21 June 2017,gocontractor.com/blog/how-does-construction-impact-the-environment/.
3 CIC-ZCP. “Zero Carbon Park. Our Mission, Vision & Value.” CIC-Zero carbon park main webpage, zcp.cic.hk/eng/mission. https://zcp.cic.hk/eng/mission https://www.bd.gov.hk/en/resources/codes-and-references/modular-integrated-construction/index.html#:~:text=Modular%20Integrated%20Construction%20(MiC)%20refers,for%20installation%20in%20a%20building.
4 “Modular Integrated Construction.” Buildings Department, 28 Oct. 2022, www.bd.gov.hk/en/resources/ codes-and-references/modular-integrated-construction/index.html#:~:text=Modular%20Integrated%20Construction%20(MiC)%20refers,for%20installation%20in%20a%20building.
5 Build King Contruction Ltd. “CIC Zero Carbon Park - emMiC (Stormwater Air Conditioning System).” https://www.scienceabc.com/innovation/air-conditioner-ac-work.html
CIC Zero Carbon Park - emMiC (Stormwater Air Conditioning System), e-book ed., p. 1. Pdf.
6 Ashish. “How Does an Air Conditioner (AC) Work?” ScienceABC, 22 Jan. 2022, www.scienceabc.com/ innovation/air-conditioner-ac-work.html.
7 Richariya, Geetam, and Anil Kumar. “Solar cell technologies.” ScienceDirect, 2020, www.sciencedirect.com/topics/engineering/cadmium-telluride-solar-cell.
8 Amin, Nowshad, and Seyed Ahmad Shahahmadi. “Sustainable Energy Technologies & Sustainable Chemical Processes.” ScienceDirect, 2017, www.sciencedirect.com/topics/engineering/ cadmium-telluride-solar-cell.
9 “Cross Ventilation | Wind Effect Ventilation | Moffitt Corp.” Moffitt, www.moffittcorp.com/wind-effect-cross-ventilation/.
60 Scientific Harrovian 2022
Medicinal Applications of SPIDER SILK
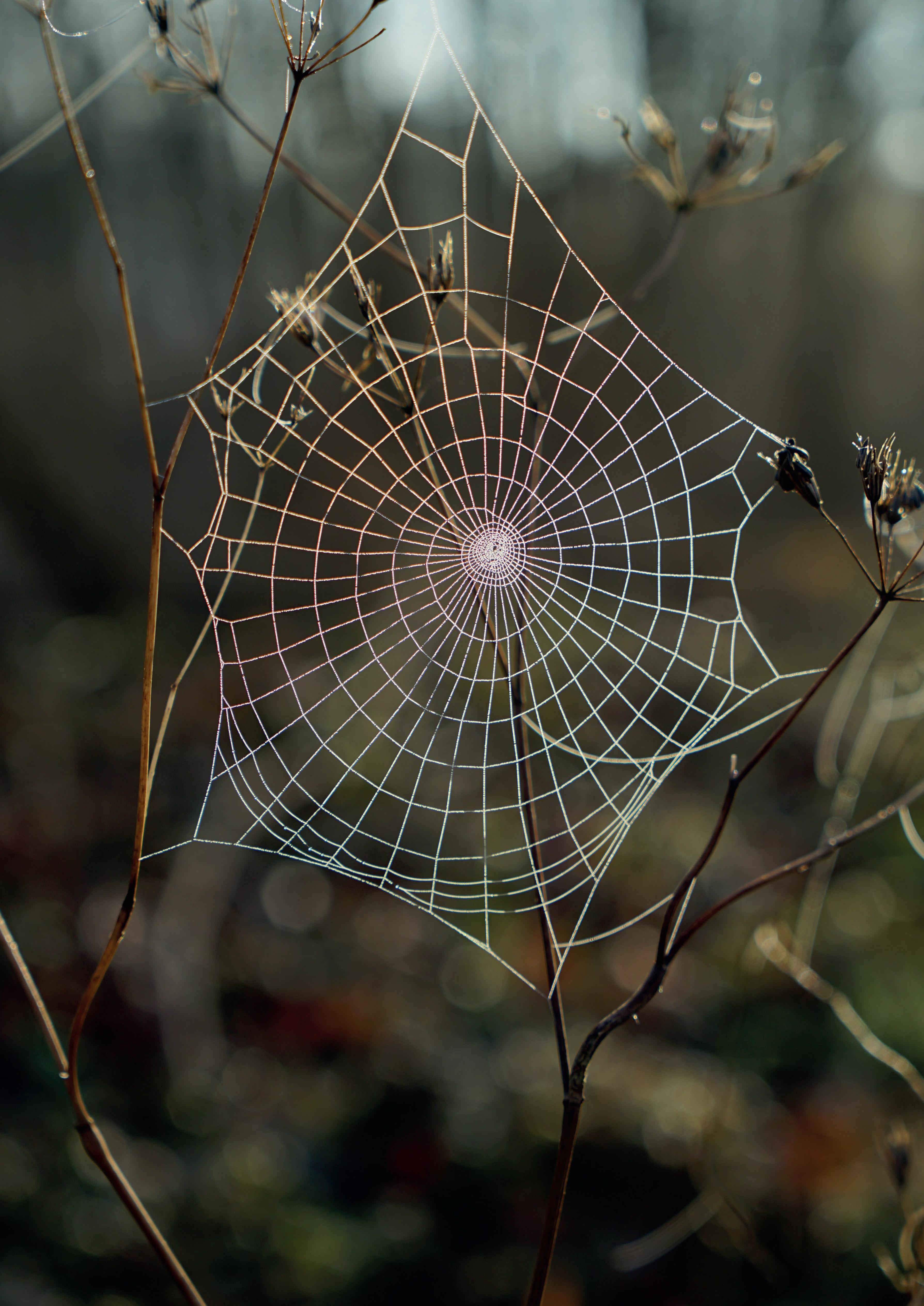
by Jasmine Wong
Silk is a protein fibre spun by spiders to form webs for nests and cocoons, or to catch prey. Their unique properties, such as high toughness and extensibility, make them an excellent new biomaterial.
How is dragline silk naturally produced?
Each orb-weaving female spider has seven different glands, producing seven types of silk with unique properties depending on their purposes. The silk is stored as a liquid in the internal silk glands before it is secreted. It passes through the spigot to the spinnerets on the spider’s abdomen, where it is spun into fibre, forming gossamer [2, 18]. Among these seven different glands, the major ampullate (MA) silk is one of the spider’s most valuable silks, also known as dragline silk.
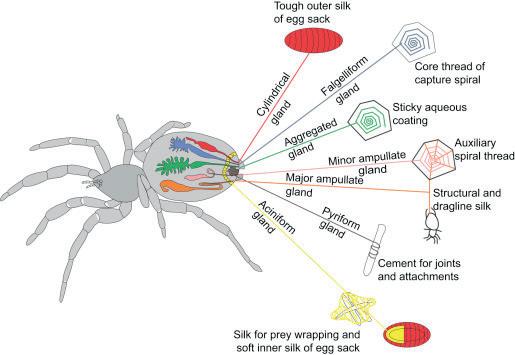
Mechanical Properties of Dragline Silk
The mechanical properties of dragline silk are affected by the amino acids present, insect size, diet, and body temperature [24]; the differences in mechanical properties are dictated by the type of secondary structure of the spider silk, which can be split into four different major motifs [9].
In summary, the primary structure of dragline silk is composed of a sequence of repetitive glycine and alanine blocks [25]. The chain of alanine is mainly found in the crystalline domains of the protein nanofibril composite as shown in Figure 2, whereas glycine is located in the amorphous matrix consisting of beta turns.
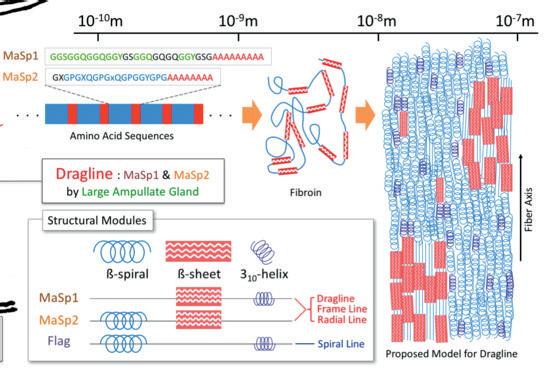
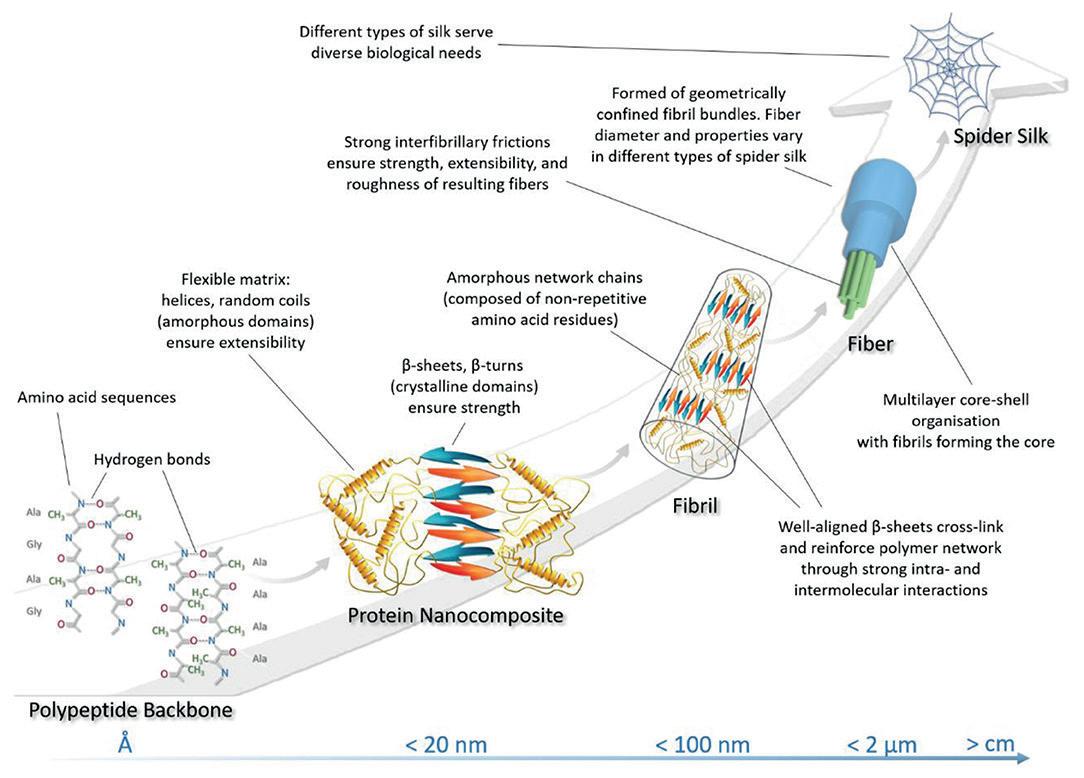
High Young’s modulus and tensile strength
The tensile strength (the greatest stress before breaking) of dragline silk is on par with the tensile strength of high-carbon steel (650 Mpa) [19]. The natural dragline silk produced by spiders can have a strength of up to four or five times that of steel. It has one of the highest breaking energies; hence, it is very tough and can withstand a large impact force without breaking [11]. Dragline silk also can undergo large tensile and compressive strains without fracturing or experiencing plastic deformation.
Dragline silk requires a large force of 1.1 x 109 Nm-2 to break under tension when stretched out at either end [9]. To put this into perspective, if you were to be stepped on by an average-sized African bush elephant that weighs 6,000 kg and has a foot size of 0.3 m2 , it would only exert an estimated 20,000 Nm-2 on you. A spider silk would require 55,000 elephants stacked on top of each other to break. If the silk is stretched to its yield point, some of the intermolecular interactions would break, causing the amorphous protein chains to extend, uncoil, and straighten out whilst keeping the polypeptide chain intact. As a result, it can be plastically elongated without losing strength under tensile stress – giving dragline silk its high tensile strength property. Furthermore, the combination of being able to withstand high stress yet experiencing little strain due to untangling of amorphous chains means spider silk has a high Young’s modulus value of 10 GPa. Young’s modulus is the stress-to-strain ratio, i.e., how much pressure will give a certain amount of deformation. This means it is very stiff and resistant to bending or stretching, due to hydrogen bonds breaking by a frictional stick-slip motion, in which energy is dissipated through the amorphous matrix.
Elasticity
The elastic properties of dragline silk further make it an excellent material. The extension of spider silk can be up to two to four times its original length, which is much longer than steel [11]. If an insect flies perpendicular to the silk, the web would need to absorb most of the kinetic energy of the insect’s forward velocity to bring it to a stop without causing the prey to be catapulted out of the web. The dragline silk can do this by transferring 65% of the kinetic energy to thermal energy and storing the rest as elastic deformation as it stretches and recoils back to its original shape and length. To do this, the silk must be extremely tough and extensible. Atomic force microscopy reveals that the silk contains a fibrillar structure with bleated fibrils at the fibre’s core. Bleated fibril is a section of fibre that has a completely non-repetitive random arrangement of loops. This core allows for a large force to be applied without breaking by extending itself, as shown in Figure 4 below. Figure 4 also shows that dragline silk has a toughness of 160 MJm-3, meaning the silk can absorb a large amount of energy per unit volume and recoil after a force has been removed. This is because the surface crack propagates only up to a certain point in the crystallite [9] [24].
Dragline silk is fibroin and is made up of MaSp1 and MaSp2 Spidrion proteins which are composed of a repeating sequence of approximately 3,500 amino acids with 42% glycine and 25% alanine [6]. Glycine-rich regions give spider silk its elastic properties where a sequence of 5 amino acids is repeated. Unlike other amino acids, which have carbon instead, spider silk contains hydrogen as a side chain [3]. This is further enhanced by the fact that a β turn (180o) occurs periodically after each complete sequence, forming a β-spiral. Furthermore, the β spiral provides a tight helix which can extend with the amorphous area in the silk structure, providing elastic and tough properties. Its toughness is also brought about by the high molecular mass of the protein, causing many Van der Waal forces between the short-chain amino acids as well as the many hydrogen bonds between carbonyl and amide groups.
These forces are embedded in an amorphous glycine matrix consisting of helical and β-turn spiral structures. Additionally, the solid intermolecular forces of attraction between the layers of crystalline structure, i.e. disulfide bridges, give rise to elastic properties. Hence, when a force is applied, the silk is initially slightly stiff until the weak cross-links between the tangled chains are broken. The chains are then uncoiled, increasing strain for a little extra stress until the molecules become aligned. The band now becomes stiff as the strong covalent bonds between the atoms are stretched. On releasing the stress, the chains recoil back to their original structure.
The spring-like structure of the proline provides the corresponding restoring force to balance the torque acting on it [11]. β-turn is also neatly arranged to form a ‘cable-like structure’ which increases the crystalline stability and strengthens the spider silk. The elastic properties are further enhanced by the GPGXX region in the bleated fibrils where the hydrophobic crystalline structure is. This comes from having strong covalent bonds between every three amino acids in the helical structure, which further increase the tensile strength.
The fibre extensibility of the dragline silk compensates for strength when fibres are loaded on long silk, causing the web fibre to bend downwards, stretching the fibre with F=mg/2sinθ. Dragline silk is different from other low extensibility fibres: rather than the deflection angle becoming smaller, which will cause a larger force to develop, a larger angle allows for a higher εmax of 0.27. This is significant compared to the Kevlar fibre because even with a tenth of the normalised deformation, it will only support a load 40% less than the dragline silk. This is effective for spiders when catching insects flying at a high velocity.
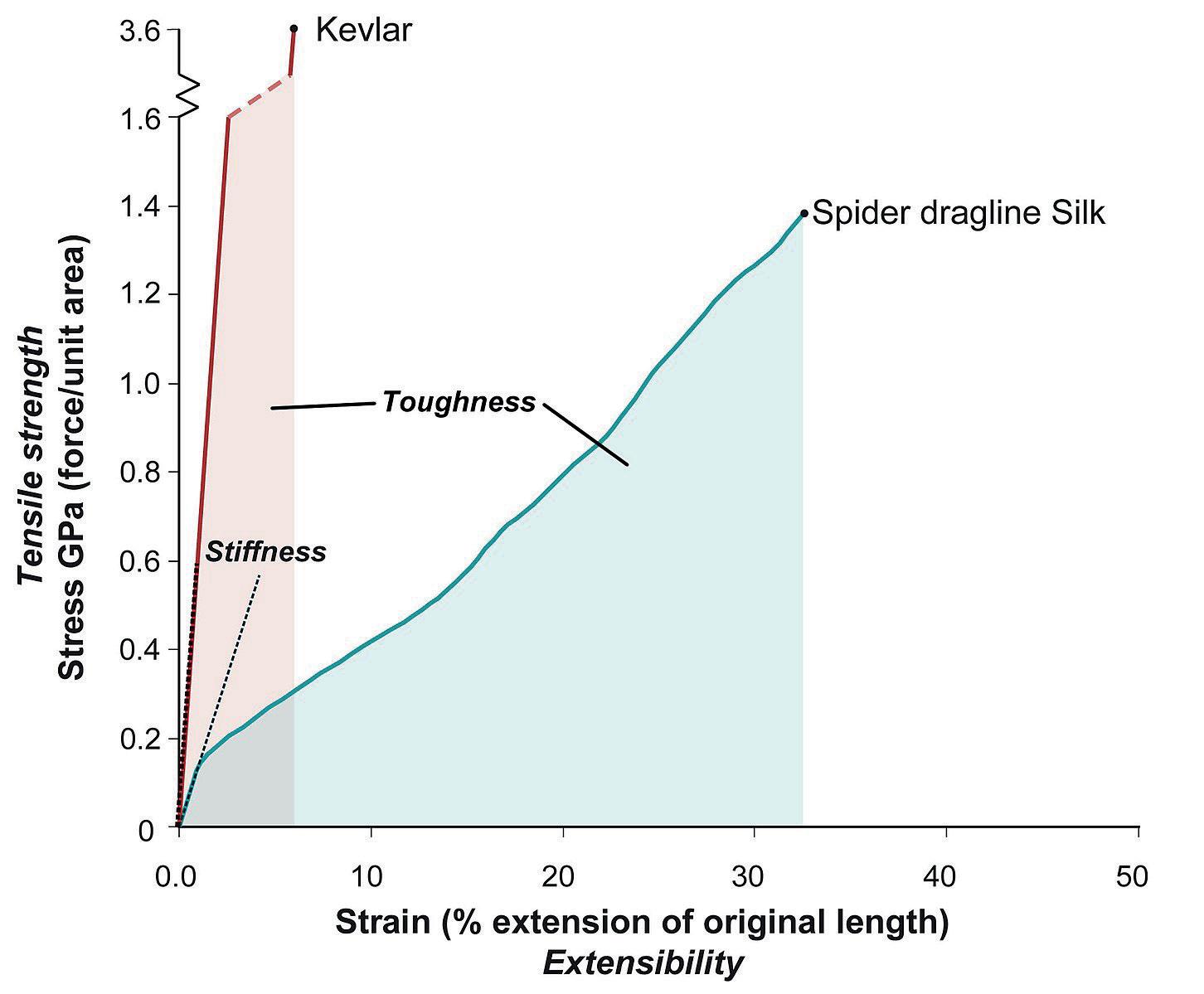
Supercontraction
When submerged in water, spider silk fibres contract, causing a phenomenon known as a super contraction [11]. Changing humidity and moisture can cause the dragline silk to shrink by 40-50% and decrease stiffness by three orders of magnitude, causing it to behave like rubber. Supercontraction, therefore, keeps the web taut. This is believed to be the result of the polar acids forming bonds and interacting with neighbouring atoms.
Biocompatibility
The proteins in dragline silk are biocompatible to humans as they do not contain any toxic substances, nor do they cause immune rejection reactions from the human body. It was suggested in a study that silk produced by the common house spider, T. domestic, had bacteriostatic effects on B. subtilis [32] . The reasons for the reduction were suggested to be due to the copious amounts of glycoproteins in the silk. The hydrophobic nature of both the protein’s crystalline region and the GPGXX sequence can be degraded under specific conditions, and the degraded products can be absorbed by human tissue; hence it is an ideal wound suture and prosthesis-making material.
Glycine acts as an antioxidant, anti-inflammatory, and immunomodulator since it acts as a neurotransmitter in the central nervous system [4]. Being an immunomodulator means that it can change the immune system of the host by activating or suppressing it. Since spider silk is a natural material, it is cheaper and easier to use than manufactured materials. It has antimicrobial properties and is non-immunogenic, non-reactive and biodegradable. The response of mammalian cell lines cultured in vitro to Nephila clavipes further demonstrated that it did not evoke an autoimmune response [32].
Recombinant spider silk production
Harvesting a substantial amount of spider silk from natural sources is a difficult task; hence scientists have been able to produce recombinant spider silk to meet the demands [23]. To form a recombinant protein, recombinant DNA (rDNA) must first be formed. rDNA is a DNA strand that is formed by the combination of two or more DNA sequences from the same species. It can be artificially produced using rDNA technology to put into a host cell. The most common recombinant spider silk proteins are based on the sequence of Araneus diadematus. The most common host organisms used to manufacture the recombinant protein are E.coli, as they proliferate and have high cell density and easy transformation, i.e. high efficiency of introducing DNA molecules into cells and increased ability to express proteins.
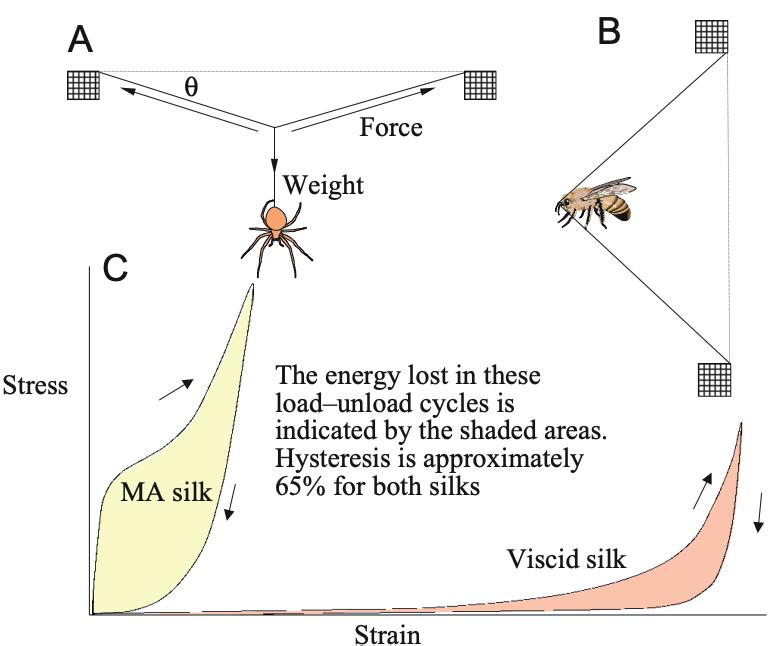
The formation of recombinant protein is as follows:
1. Restriction enzymes cut at a specific sequence of DNA to produce short, single-stranded overhangs[12].
2. Recombinant DNA is formed when the cut DNA fragment (target gene for the production of spider’s silk) is inserted into a vector (a plasmid) using DNA ligase.
3. The resulting vector is inserted into a host cell, ie. E.coli, in a process called transformation. This is done by shocking the bacterial cells with conditions such as high temperature to encourage them to take up foreign DNA.
4. The bacterial cells can now produce the recombinant protein.
5. Once the protein has been produced, the bacterial cells can be split open to release it.
6. The target protein must then be purified or separated from the other contents of the cells by biochemical techniques due to the presence of many other macromolecules around the bacteria besides the target protein.
Medical applications
Tissue engineering
Langer and Vacanti defined tissue engineering as ‘an interdisciplinary field which applies the principles of engineering and life sciences towards the development of biological substitutes that restore, maintain or improve tissue function’ to induce tissue-specific regeneration processes [5]. There are two approaches to tissue engineering. ‘Bottom-up’ is the modular assembly of building units into tissue resembling constructs [15]. ‘Top-down’ is the simple combination of existing components within a given structure.
Example 1: Silk Matrix for tissue-engineered anterior cruciate ligament (ACL)
The high tensile strength and Young’s modulus matches the mechanical properties needed for an ACL [1]. It is also a biocompatible material and avoids bioburdens associated with mammalian-derived materials. It also does not degrade quickly, which means it provides sufficient time for the host tissues to infiltrate and eventually grow back and stabilise the leg.
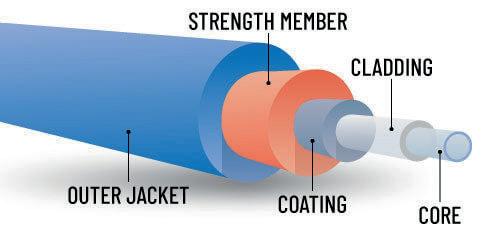
Example 2: Cardiac regeneration
The primary cause of impaired heart function is the loss of cardiomyocytes [10]. One innovative approach is to use spider silk to help grow new cardiac muscle tissue. By using bioengineered spider silk hydrogels as a base, scientists have been able to restore heart tissues. Cardiomyocytes, harvested from human-induced pluripotent stem cells (hiPSCs), are genetically modified using CRISPR/Cas-9 to allow the surface to be negatively charged, so that it can adhere to the positively charged bioengineered spider silk protein. This allows hypertrophy which repairs the sections of the heart. More importantly, the new cardiomyocytes also demonstrate the ability to communicate with other cells.
Prosthesis
An electro-tendon is a part of the robotic hand made up of spider silk which allows electrical signals to be transmitted to the pressure sensor by the transmission of electrical signals through to the pressure sensor, allowing the finger to bend under the control of a motor. It is able to do this as spider silk is a super tough conductor that can act as an electrode. The durable and flexible nature of spider silk allows the electrotendon to withstand up to 40,000 cycles of bending and stretching in a prosthesis hand [22].
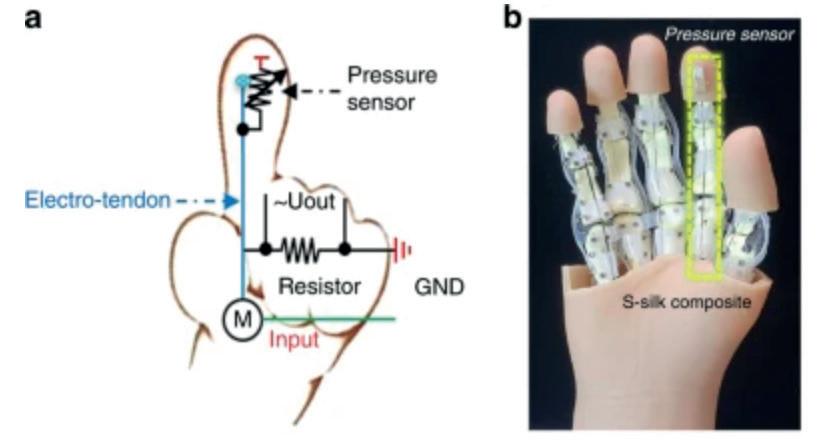
Silk optics
Researchers from Taiwan have made biosensors using spider silk [20]. The dragline silk is harvested from Nephilia pilipes which is native and abundant in Taiwan. Spider silk forms the core of the optic fibres, whilst the biocompatible photocurable resin acts as the cladding. It is further enhanced by adding a nanolayer of gold to enhance the fibre’s sensitivity. In optomechanics, spider silk can be used to form sensors that can detect and measure tiny changes in the refractive index of a biological solution which contains glucose [30]. The beam produced is called a photonic nanojet (PNJ). This is useful as it can be used for biomedical nanoimaging applications, such as measuring blood sugar levels with a higher degree of accuracy without being invasive and expensive [20].
Sutures
Biotechnological spider silk production has enabled scientists to genetically modify the sequence of amino acids within the spider silk, thus altering the chemical and physical properties of spider silk proteins. With artificial spider silk, molecules like antibiotics and fluorescent dyes can be attached to desired soluble silk protein [13]. Antiseptic properties effectively clot blood because of its high vitamin K content. Hence, the rapid regeneration of biocompatible and multifunctional spider silk can have a wide range of applications that is useful for biomedical applications.
The point of sutures is to promote wound healing and avoid infections, but the suture itself is susceptible to causing bacterial infection of biofilms [11]. Thus, an extra layer of antibiotic-based antibacterial coating is added to the sutures to prevent bacterial biofilm formation. However, as microorganisms mutate and become resistant to antibiotics due to selection pressure causing evolution by natural selection, an alternative solution to this problem must be found. The biocompatibility, minimal immune response and controlled biodegradability make dragline silk most suitable for this role. Additionally, because spider silk can be easily degraded, it eliminates any changes or dressing removals that would typically be painful for the patient [7].
Conclusion
In conclusion, the dragline silk produced in the major ampullate gland in spiders provides a naturally high Young’s modulus, tensile strength, elasticity and extensibility. It is not only biocompatible and biodegradable, but it also does not evoke an immune response when used on other mammals, making it an attractive material for biomedical applications such as sutures and optic fibres.
However, the source of dragline silk production should be carefully considered, as exploiting spider glands is unethical since it is unnatural for them to keep making silk. In some cases, it is also inhumane to do so as they are involuntarily sedated with carbon dioxide gas and pinned down by their limbs and abdomen to keep them in place. Tweezers are then used to pull out silk from the spinnerets and attach it to the pool with some glue. The motor then begins to spin to harvest the silk from the spider [26].
Alternative methods should be found to avoid harming the spiders. This can be done by studying the structure of the spiders and using biomimicry to replicate the properties.
[1] Altman, Gregory H., et al. “Silk matrix for tissue engineered anterior cruciate ligaments.” Science Direct, October 2002, https://www.sciencedirect.com/science/ article/abs/pii/S0142961202001564. Accessed 1 November 2022.
[2] Ault, Alicia. “Ask Smithsonian: How Do Spiders Make Their Webs?” Smithsonian Magazine, 3 December 2015, https://www.smithsonianmag.com/smithsonianinstitution/ask-smithsonian-how-do-spiders-make-webs-180957426/. Accessed 28 October 2022.
[3] Betts, M. J., and R. B. Russell. “Glycine: Amino acid properties and consequences of substitutions.” Russell Lab, M R Banes, 2003, http://www.russelllab.org/aas/ Gly.html. Accessed 28 October 2022.
[4] BYJU. “Glycine - Structure, Properties, Uses & Benefits with Images and FAQs.” Byju’s, https://byjus.com/chemistry/glycine-structure/. Accessed 28 October 2022.
[5] Campuzano, Santiago, and Andrew E. Pelling. “Tissue Engineering Approaches in the Design of Healthy and Pathological In Vitro Tissue Models.” Frontiers, 26 June 2017, https://www.frontiersin.org/articles/10.3389/fbioe.2017.00040/full. Accessed 1 November 2022.
[6] CHM BRIS. “Spider Silk.” Spider Silk, http://www.chm.bris.ac.uk/motm/spider/page3.htm. Accessed 28 October 2022.
[7] Cuffari, Benedette. “Role of Spider Silk in Biomedicine.” News Medical, 14 December 2020, https://www.news-medical.net/life-sciences/Role-of-Spider-Silk-inBiomedicine.aspx. Accessed 6 November 2022.
[8] Doistau, Benjamin, et al. “Recent Advances in Development of Functional Spider Silk-Based Hybrid Materials.” Frontiers, 29 May 2020, https://www. frontiersin.org/articles/10.3389/fchem.2020.00554/full. Accessed 12 November 2022.
[9] Ebrahimi, Davoud, et al. “Silk–Its Mysteries, How It Is Made, and How It Is Used.” NCBI, 24 August 2015, https://www.ncbi.nlm.nih.gov/pmc/articles/ PMC4936833/. Accessed 29 October 2022.
[10] Esser, T. U. “Article Highlight: Spider silk could be used to make artificial heart tissues.” Materials Today, 28 June 2021, https://www.materialstoday.com/ biomaterials/news/article-highlight-spider-artificial-heart-tissues/. Accessed 5 November 2022.
[11] Gu, Yunqing, et al. “Mechanical properties and application analysis of spider silk bionic material.” De Gruyter, 24 August 2020, https://www.degruyter.com/ document/doi/10.1515/epoly-2020-0049/html?lang=en#:~:text=The%20main%20chemical%20components%20of,has%20excellent%20elasticity%20and%20 strength.&text=Appearance%20of%20natural%20spider%20silk. Accessed 5 November 2022.
[12] Khan Academy. “Overview: DNA cloning (article).” Khan Academy, 2022, https://www.khanacademy.org/science/ap-biology/gene-expression-and-regulation/ biotechnology/a/overview-dna-cloning. Accessed 1 November 2022.
[13] Kirsh, Danielle. “Artificial spider silk: Why you should care about it.” Medical Design & Outsourcing, 10 January 2017, https://www. medicaldesignandoutsourcing.com/artificial-spider-silk-used-medical-applications/. Accessed 1 November 2022.
[14] Ko, Frank K., and Lynn Y. Wan. Handbook of Properties of Textile and Technical Fibres. 2 ed., The Textile Institute Book Series, 2018.
[15] Langer, Robert. “Tissue Engineering.” Science, 14 May 1993, https://www.science.org/doi/10.1126/science.8493529. Accessed 1 November 2022.
[16] Mahan, Gerald D. “amorphous solid | physics | Britannica.” Encyclopedia Britannica, 31 July 2019, https://www.britannica.com/science/amorphous-solid. Accessed 12 November 2022.
[17] “Mechanical properties and application analysis of spider silk bionic material.” De Gruyter, 2020, https://www.degruyter.com/document/ doi/10.1515/epoly-2020-0049/html?lang=en#:~:text=The%20main%20chemical%20components%20of,has%20excellent%20elasticity%20and%20 strength.&text=Appearance%20of%20natural%20spider%20silk. Accessed 28 October 2022.
[18] Merda, Chad. “Nature curiosity: How do spiders make silk?” Forest Preserve District of Will County, 4 October 2019, https://www.reconnectwithnature.org/ news-events/the-buzz/nature-curiosity-how-do-spiders-make-silk. Accessed 28 October 2022.
[19] Nuclear Power. “High-carbon Steel | nuclear-power.com.” Nuclear Power, 2022, https://www.nuclear-power.com/nuclear-engineering/metals-what-are-metals/ steels-properties-of-steels/high-carbon-steel/. Accessed 28 October 2022.
[20] Optica. “Researchers create biosensor by turning spider silk into optical fiber | News Releases.” Optica, 2 August 2022, https://www.optica.org/en-us/about/ newsroom/news_releases/2022/august/researchers_create_biosensor_by_turning_spider_sil/. Accessed 5 November 2022.
[21] Orkin. “What is Spider Silk? | Spider Silk Types & Facts.” Orkin, 2022, https://www.orkin.com/pests/spiders/spider-silk-facts-and-information. Accessed 28 October 2022.
[22] Pan, Liang, et al. “A supertough electro-tendon based on spider silk composites.” Nature communications, 12 March 2020, https://www.nature.com/articles/ s41467-020-14988-5#:~:text=The%20electro%2Dtendon%20is%20a,without%20any%20change%20in%20conductivity. Accessed 1 November 2022.
[23] Salehi, Sahar, et al. “Spider Silk for Tissue Engineering Applications - PMC.” NCBI, 8 February 2020, https://www.ncbi.nlm.nih.gov/pmc/articles/ PMC7037138/. Accessed 1 November 2022.
[24] Saravanan, D. “Spider Silk - Structure, Properties and Spinning.” Journal Of Textile and Apparel, Technology and Management, vol. 5, no. 1, 2006, p. 1. JTATM. Accessed 29 October 2022.
[25] Shishir, Hasan. “Spider silk.” SlideShare, 13 May 2014, https://www.slideshare.net/sheshir/spider-silk-34606555. Accessed 6 November 2022.
[26] “Spider Silk Harvesting.” jwz.org, 2014, https://www.jwz.org/blog/2015/03/spider-silk-harvesting/. Accessed 6 November 2022.
[27] “Tissue Engineering.” Science, 14 May 1993, https://www.science.org/doi/10.1126/science.8493529.
[28] Vesselnet. “Three Basic Components of a Fiber Optic Cable.” Vesselnet, https://www.vesselnetintegrated.com/single-post/three-basic-components-of-a-fiber-opticcable. Accessed 12 November 2022.
[29] Vincentsarego. “File:Wikipedia Kevlar Silk Comparison.jpg.” Wikimedia Commons, 17 June 2011, https://commons.wikimedia.org/wiki/File:Wikipedia_ Kevlar_Silk_Comparison.jpg. Accessed 12 November 2022.
[30] Weiler, Sarah. “Spider silk spins a stronger lens | Post Scripts | Sep/Oct 2020 | BioPhotonics.” Photonics.com, October 2020, https://www.photonics.com/Articles/ Spider_silk_spins_a_stronger_lens/a66044. Accessed 5 November 2022.
[31] Whittall, Dominic R., et al. “Host systems for the production of recombinant spider silk.” Cell, 10 October 2020, https://www.cell.com/trends/biotechnology/ fulltext/S0167-7799(20)30244-4. Accessed 1 November 2022.
[32] Wright, Simon. “Evidence for antimicrobial activity associated with common house spider silk.” NCBI, 25 June 2012, https://www.ncbi.nlm.nih.gov/pmc/ articles/PMC3443048/. Accessed 1 November 2022.
[33] Zhao, Yue. “File:0.Figure.png.” Wikimedia Commons, 7 June 2016, https://commons.wikimedia.org/wiki/File:0.Figure.png. Accessed 12 November 2022.
[34] CRISPR. “CRISPR/Cas9 | CRISPR.” CRISPR Therapeutics, 2022, https://crisprtx.com/gene-editing/crispr-cas9. Accessed 14 December 2022.