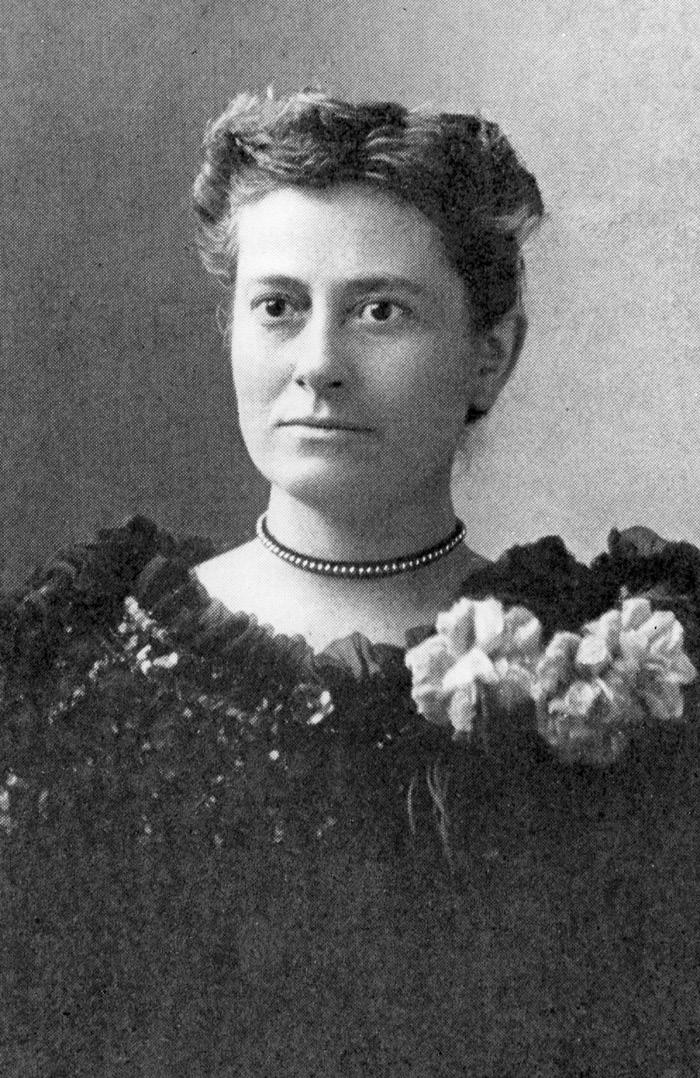
42 minute read
Listening to the Song of the Universe
SCOTTISH MAID
Williamina Fleming was definitely another Harvard computer and her work is finally receiving public acclaim. She discovered the extremely hot, dense ‘white dwarf’ stars and was the first person to see the Horsehead Nebula beyond the eastern star of the constellation Orion – admittedly on a photographic plate and not in glorious technicolour like the images produced from the Hubble Space Telescope. She also classified over 10,000 stars, something astronomer Herbert Hall Turner deemed ‘an achievement bordering on the marvellous’.
Advertisement
Originally from Scotland, Fleming worked as a teacher before emigrating to Boston with her husband in 1878. When Fleming was pregnant with their first child, he abandoned her, forcing Fleming to seek a position at the observatory as a maid. Fortunately, the observatory director at the time, Edward Pickering, recognised her intelligence and talents and she soon switched from dusting the furniture to working as a computer. She would go on to become the observatory’s first Curator of Astronomical Photographs and one of America’s most prominent female astronomers. In 1906 Fleming became the first American woman elected as an honorary member of the RAS.
Born a few years after Fleming, in 1863, Annie Jump Cannon built on Fleming’s work and became a renowned expert in classifying stars. She also combined two stellar classification methods – a joint one by Fleming and Pickering and the other by Antonia Maury. The Fleming–Pickering method was an alphabetical one dividing stars into twenty-two classes dependent on hydrogen content – A-type stars containing the most hydrogen. Combining the two methods meant these spectral classes, which are still in use today, no longer follow an A, B, C order, but instead run as follows: O, B, A, F, G, K, M. This used
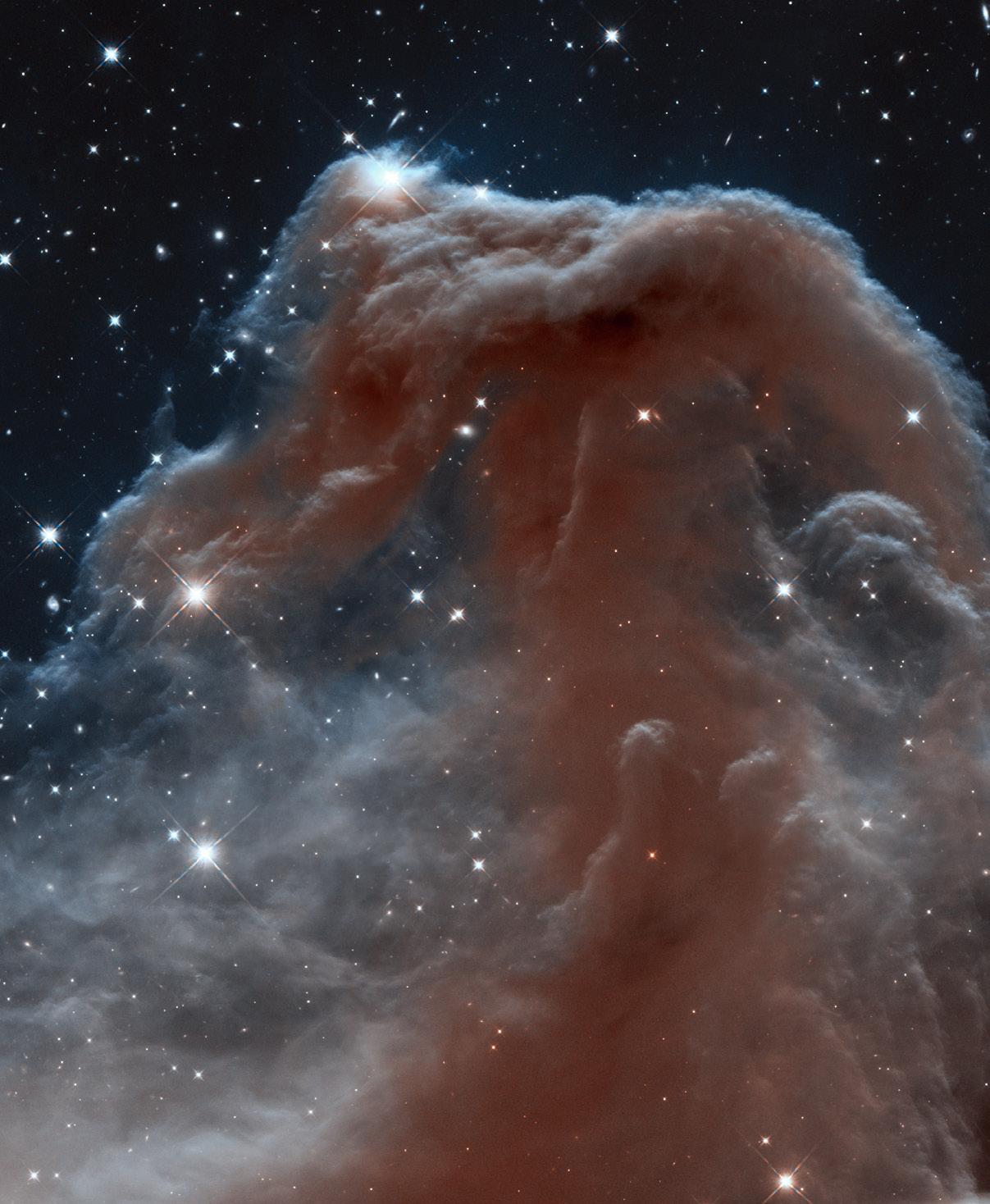
OPPOSITE: Williamina Fleming. Harvard College Observatory, courtesy of AIP Emilio Segrè Visual Archives ABOVE: The Horsehead Nebula as captured by Hubble. NASA/ESA/Hubble Heritage Team
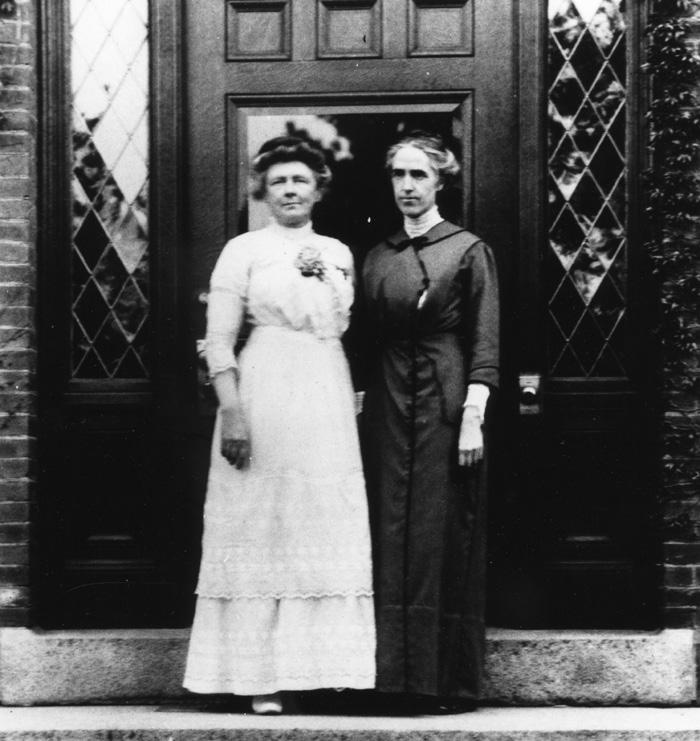
to be recalled by the mnemonic ‘Oh Be A Fine Girl and Kiss Me’, though nowadays #MeToo may have put paid to that! Jump Cannon classified around 350,000 stars – an extraordinary feat – and was the first woman ever to receive an honorary degree from Oxford University.
Then there was Henrietta Swan Leavitt, who identified that the luminosity of a variable star is related to its pulsation period, now known as Leavitt’s Law. She worked out how to measure the ‘intrinsic’ luminosity of stars – how bright a star is as opposed to how bright it appears to be, an important distinction. Otherwise, two stars of equal brightness could appear to be the same distance from Earth when one might be a huge bright star far away and the other a smaller, less bright star but a lot nearer.
Leavitt’s insights enabled astronomers to measure the distances of remote stars and galaxies and proved crucial for Edwin Hubble to prove that the Andromeda galaxy was outside our own. A testimony to the importance of Leavitt’s work is that a member of the Swedish Academy of Sciences nominated her for a Nobel Prize in 1924. Unfortunately, the member hadn’t realised Leavitt had died of cancer a few years beforehand. The prize is not awarded posthumously.
All these achievements are even more impressive when you realise that many of the women were not allowed to do their own observing as part of their day jobs at the observatory, meaning that their insights and research were primarily done outside work and on their own time. OPPOSITE TOP: Annie Jump Cannon and Henrietta Swan Leavitt outside Harvard College Observatory. AIP Emilio Segrè Visual Archives, Shapley Collection OPPOSITE BELOW: Henrietta Swan Leavitt. Photo by Margaret Harwood, courtesy of AIP Emilio Segrè Visual Archives, Physics Today Collection, Shapley Collection LEFT: Ellen Dorrit Hoffleit in 1973. AIP Niels Bohr Library & Archives
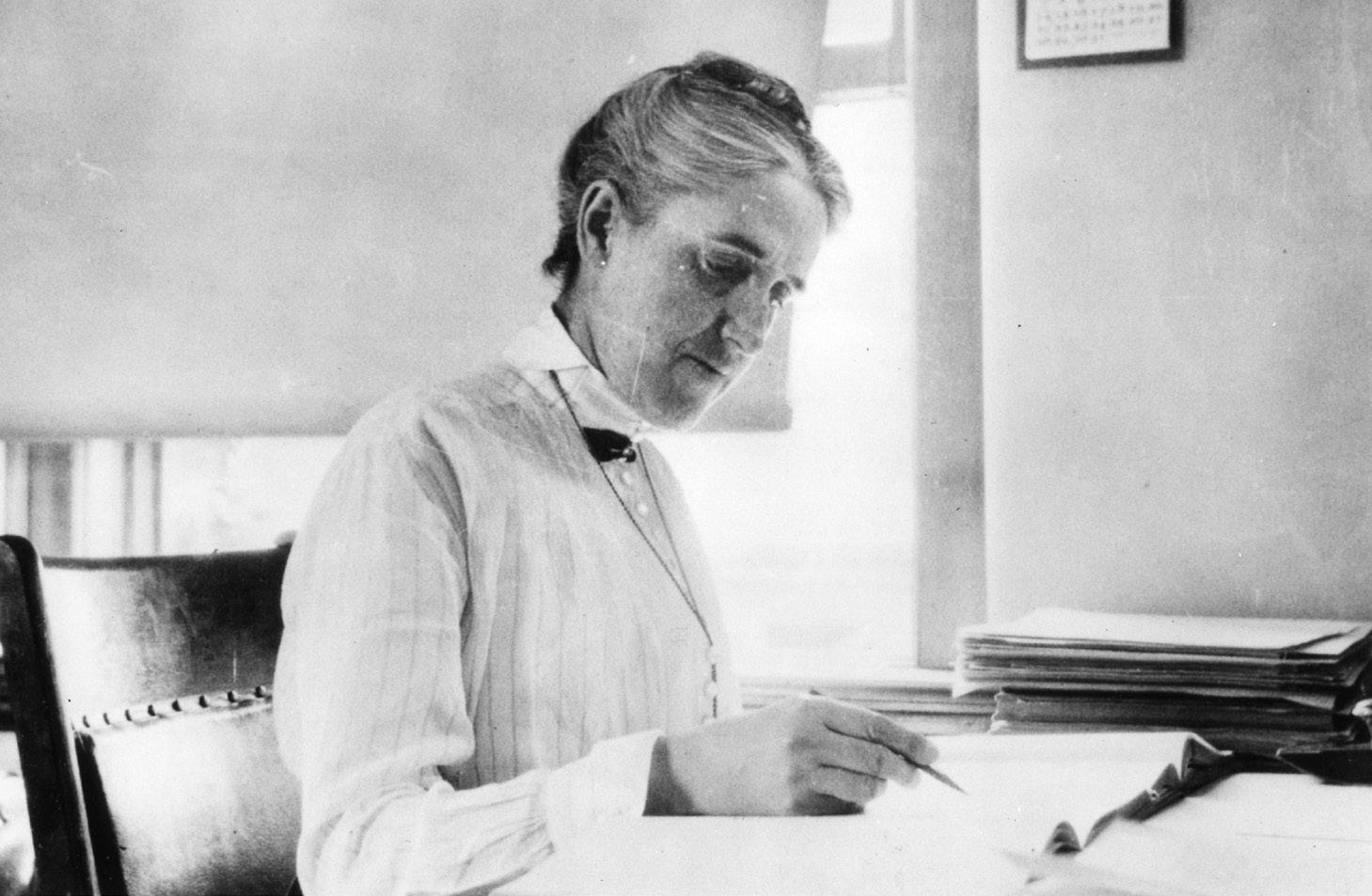
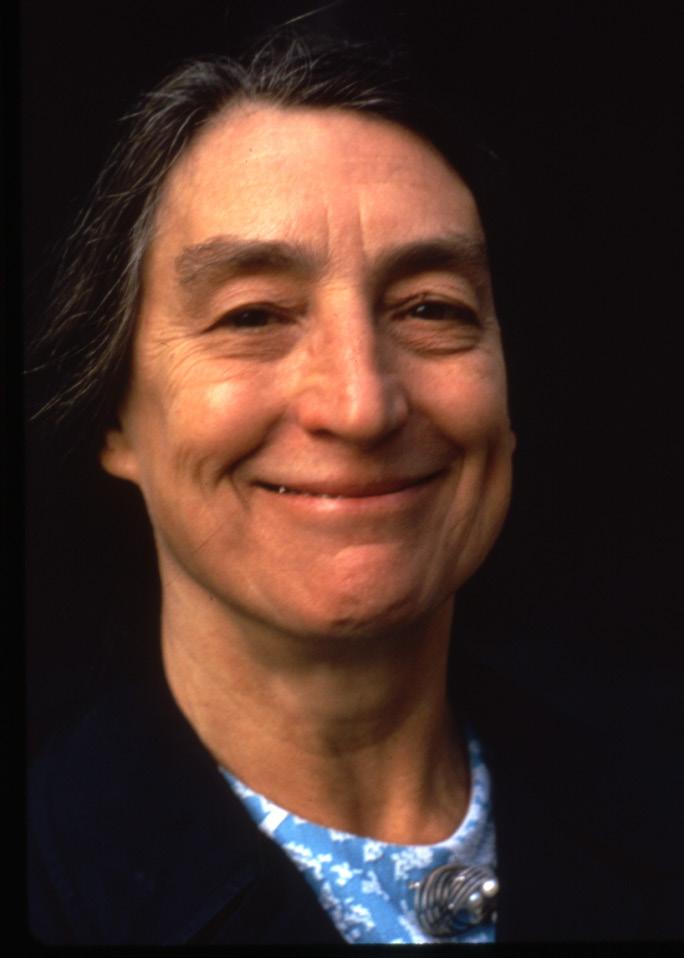
RAZOR-SHARP HOFFLEIT
In 2004, I was lucky enough to meet a woman who had actually worked alongside some of these computers. Dorrit Hoffleit, like Cecilia Payne, was never one herself. She had a degree from a women’s college and joined the observatory, in 1924, as a research assistant to Henrietta Scope, who studied variable stars. Ellen Dorrit Hoffleit was ninety-six when I took a train from Boston to meet her at New Haven, Connecticut. She sparked with vitality, had a razor-sharp mind, was quick to giggle and told me how she first became interested in astronomy. ‘When I was eleven years old, my mother and I were out in the back watching the Perseid meteors and what happened shouldn’t have happened. One Perseid was coming in like this …’ – she raised her right hand – ‘and another was coming in …’ – she raised her left hand. ‘And they collided. I didn’t dare tell anyone about this afterwards because I thought they’d say I was hallucinating!’ One meteor specialist wrote that the chance of a collision occurring like this was once every 30 million years. No wonder Hoffleit decided to later make shooting stars the subject of her life’s research, along with variable stars – those whose brightness fluctuates over time. We spoke in her office at Yale University, where she
worked and wrote astronomical research papers until her death, at the age of 100, in 2007. Hoffleit recalled Jump Cannon as having ‘a charming personality. Everyone liked her.’ If she didn’t want to prolong a heated discussion with the observatory director, Harlow Shapley, ‘Miss Cannon, who was hard of hearing, unplugged her hearing aid and wouldn’t listen to any more that Shapley had to say,’ Hoffleit claimed. Hoffleit remembered her time working at the observatory alongside the computers as ‘fun’, and like the other women she was proud to be there. She praised Maury’s contributions, in particular, as being ‘a great achievement’. However, she was understandably indignant about the structure of academic astronomy during that era. ‘You appoint a woman,’ she said, ‘and then you let her do the work and be the co-author of what she’d already done!’ It seems not much had changed since Annie Maunder’s day.
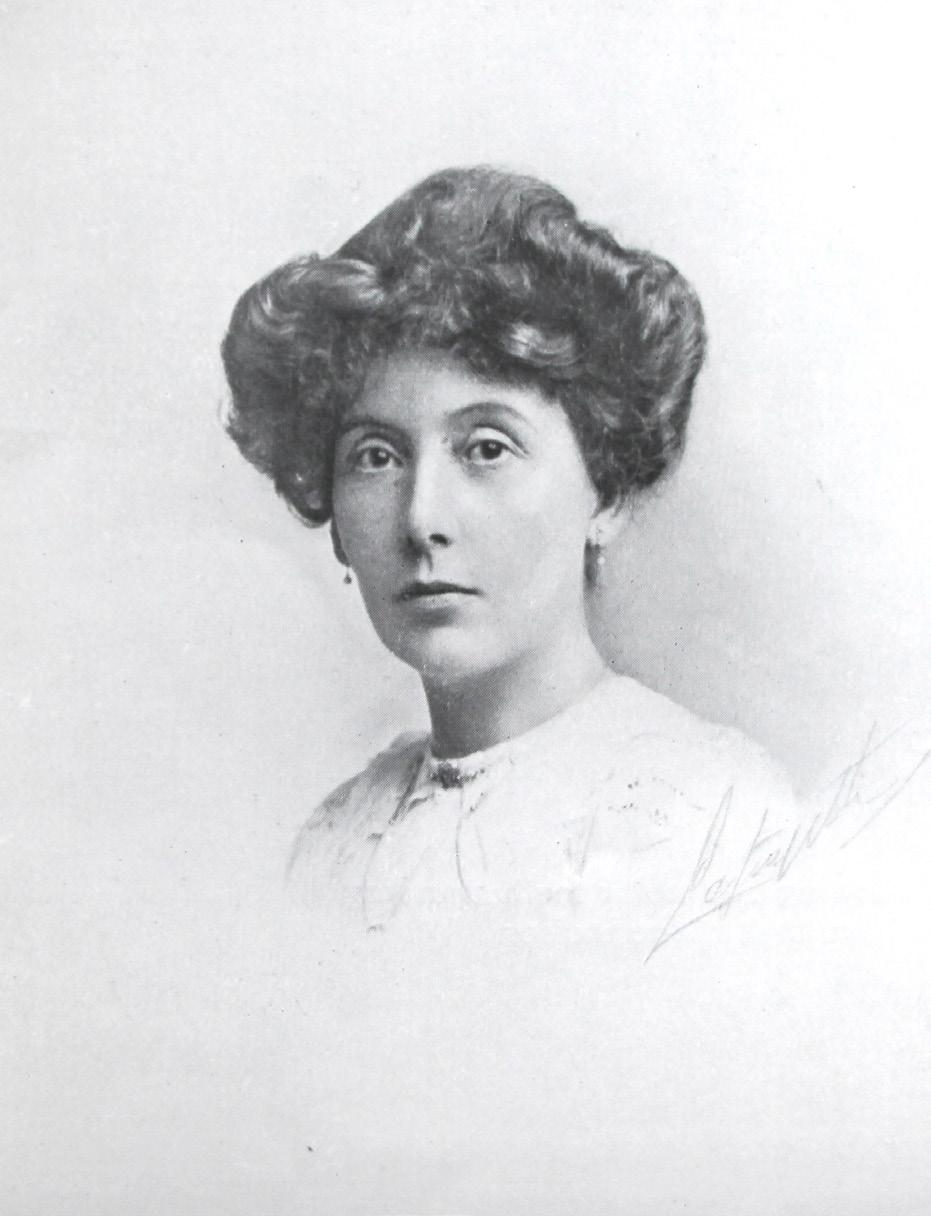
THE FLAME OF FIAMMETTA WILSON
Hoffleit wasn’t the only pioneering woman who found shooting stars utterly absorbing. Born over forty years before her, an amateur British astronomer, Fiammetta Wilson, also loved observing meteors. ‘Fiammetta recorded over 10,000 meteor observations,’ says Bill Barton, Deputy Director of the British Astronomical Association Historical Section. ‘Over 650 were doubly observed and a doubly observed meteor is much more useful for science as you can triangulate its path through the Earth’s atmosphere.’ Wilson was a fascinating character. One of the first female Fellows of the RAS, she was a meteor observer for the British Astronomical Association (BAA), as well as speaking several languages and studying music.
She had arrived into the world in Suffolk as Helen Frances Worthington, in 1864, the eldest of five children. Wilson was her married name from her second husband and the name ‘Fiammetta’ made an appearance after her first marriage had ended. At one point she was also known as a Russian professor from Poland under the name Fiammetta Waldahoff, despite being neither a professor, nor Russian, nor from Poland. But she did teach mandolin at London’s Guildhall School of Music. ‘She was basically abandoned by her first husband within a couple of years of their marriage,’ says Barton. ‘He’d committed adultery so Fiammetta had to get money from somewhere and fell back on what she knew. ‘As a child she’d had a home governess, had been to finishing school in Germany and spent a year in Italy studying music,’ Barton adds. ‘Waldahoff appears to be a complete invention – it just appears in the 1901 census.’ As for the intriguing adoption of Fiammetta as a new name, it is the Italian word for ‘little flame’ and a marvellously apt choice for a woman who loved observing meteors. But there’s another connection too. ‘The name Fiammetta comes from Italian literature,’ says Barton, ‘and it is a lady who is extremely poorly treated by men.’
Wilson’s interest in astronomy began after hearing astrophysicist Professor Alfred Fowler give a lecture at the Imperial College of Science and Technology in London, around 1910. She would make her own observations from the back garden of her marital home in Hertfordshire, using binoculars and later a telescope, and there she discovered her passion for meteors.
During the First World War, Wilson became a temporary co-director of the BAA’s meteor section with Alice Grace Cook and, while Wilson never made a significant discovery, Barton believes her name should be remembered because of her prolific observations.
SHINING STARS
Wilson’s co-director, known by her second name Grace and thirteen years Wilson’s junior, was another amateur astronomer whose passion and enthusiasm for observations and classifications also contributed to the field.
Born in Stowmarket, Suffolk, in 1877, Cook’s family was wealthy enough for her not to work and to devote herself to stargazing. Cook classified 785 objects on British astronomer John Franklin-Adams’ photographs of the night sky, taken between 1902 and 1910. ‘These images captured not only stars but nebulae. At this point in history, many of these objects remained mysterious,’ says Barton. ‘So she sees small objects, diffuse objects, and creates a category for spiral objects, which we now know to be spiral galaxies.’
Photographs of Cook at her home show a surprisingly large observatory in her back garden. This is because the BAA, which was the only astronomical society that allowed women to join at that time, once had a collection of instruments – from small telescopes to observatories – that members could borrow. ‘You applied for an instrument and it was granted to you,’ says Barton. ‘You somehow got it delivered and then you would need to write annual reports to show that you were using the instrument and it was in good working order.’
Cook discovered an unknown meteor stream and was the first person in the UK to see the Nova Aquilae (or V603 Aquilae) in 1918. It was the brightest object of its type throughout the twentieth century and could be seen in the night sky with the naked eye for several months. In 2000, Nova Aquilae was confirmed as a binary star – one of two stars that orbit each other.
Apart from observing meteors, Cook enjoyed studying the aurora borealis, or northern lights. There’s a lovely connection with the ‘lady computers’ too as, in 1920, the Harvard College Observatory gave her a grant of $500 for her astronomy work.
There is no doubt that there are many more names to uncover of women who have played an important role in humanity’s oldest science: observing the stars. Some, like the eighteenth-century German astronomer Caroline Herschel, are finally receiving interest in their own right. In the past ten years alone, at least six books have been published either devoted to her on her own or combining her achievements alongside those of her famous brother, William. For many years she had just been seen as the scribe of William’s astronomical notes. But even her note-taking made a difference.
‘Nearly everything that we have in the archives is written by her,’ says Prosser at the RAS, ‘and I have seen how she expands and clarifies on his observations. In fact, we have William’s original contemporary notes from the night that he discovered Uranus and then, nearly forty years later, he asked her to write up all of his observations and put them in a set of notebooks. And because she was there at the time, and because she knows what her brother means, she will make what he is doing more comprehensible. So she’s having that influence on the perception of his work.’
Women’s contributions to astronomy, in all their forms, hidden or not, are coming to the fore. The crater on the moon, C Herschel, celebrates Caroline in her own right and it’s clear there are many more women whose names will one day become a feature on another planet or make it on to the lunar surface.
As it happens, the astronomer who was responsible for sorting out a consistent nomenclature for the moon’s features was also a woman. Mary Adela Blagg, another pioneer among that first batch of female RAS Fellows in 1916, mapped the moon and – at a time when one crater could often have several names – created a clear system out of impending chaos.
What I love about all these different women is that they followed their passion. Whether they were a single mother in the nineteenth century or an educated priestess in what was very much a man’s world, these women were stars in so many ways and their legacy continues to shine.

Grace Cook in her back-garden observatory. From The English Mechanic and World of Science issue 2604 (19 Feb 2015)/ courtesy of The Royal Astronomical Society Library and Archives
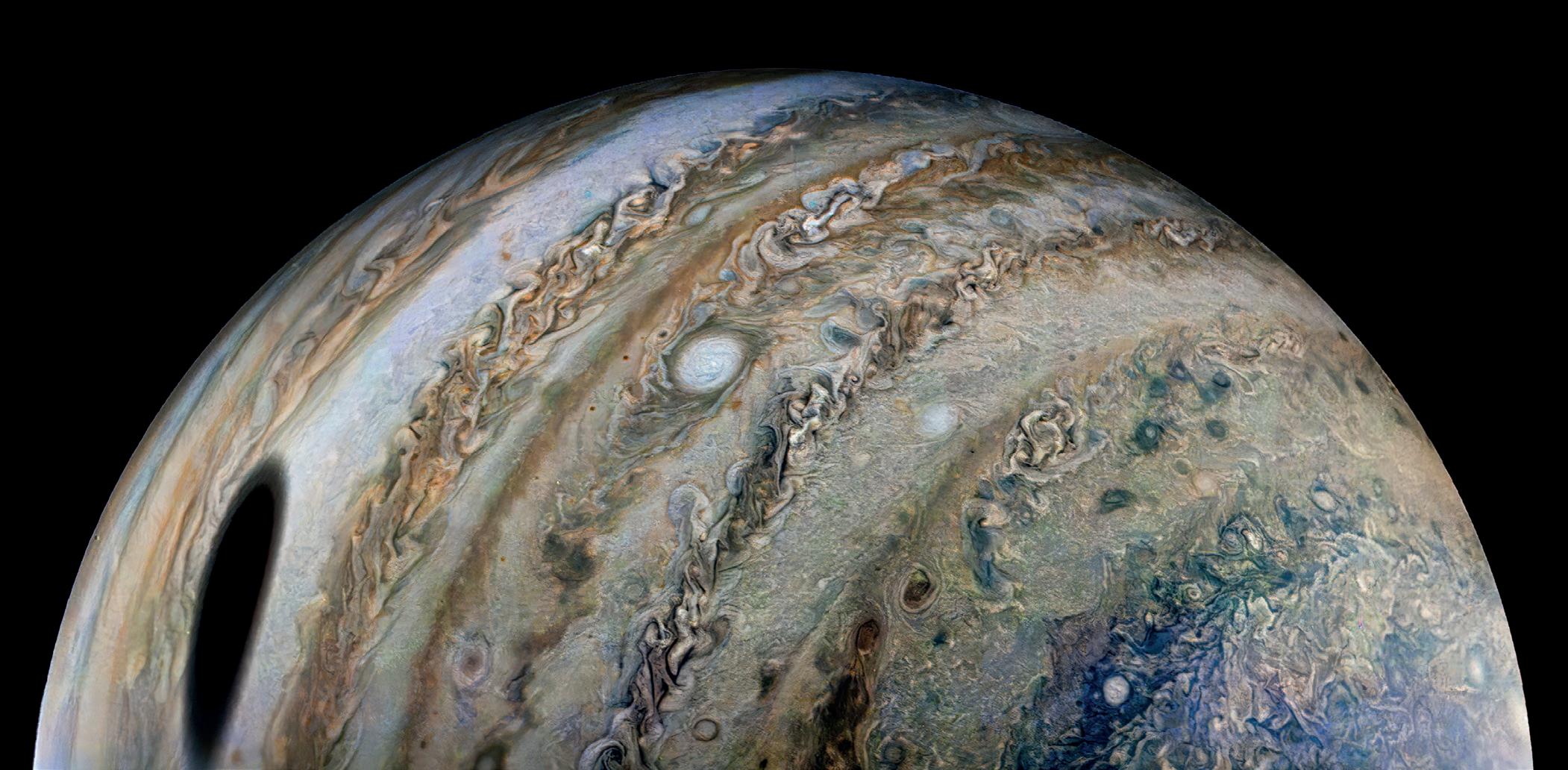
JUICE TO JUPITER
Sarah Wild looks forward to the launch of an ambitious mission to explore Jupiter’s icy moons. These mysterious worlds could be our best chance of finding life elsewhere in the solar system.
In early summer 2023, Professor Michele Dougherty will have one of the most nerve-racking hours of her life. She’s responsible for a crucial instrument on the European Space Agency’s billion-euro JUpiter ICy moons Explorer. JUICE, as it is known, is due to launch on the last ever Ariane 5 rocket and it will be shaken to within an inch of its life as it leaves the Earth and embarks on its eight-year journey to Jupiter. ‘You’ve spent ten years ensuring that this mission is going to be ready,’ explains Dougherty, who also headed up the science definition team that proposed the mission. ‘And then you watch it on top of a bloody huge rocket that you have no control over. Terrifying!’
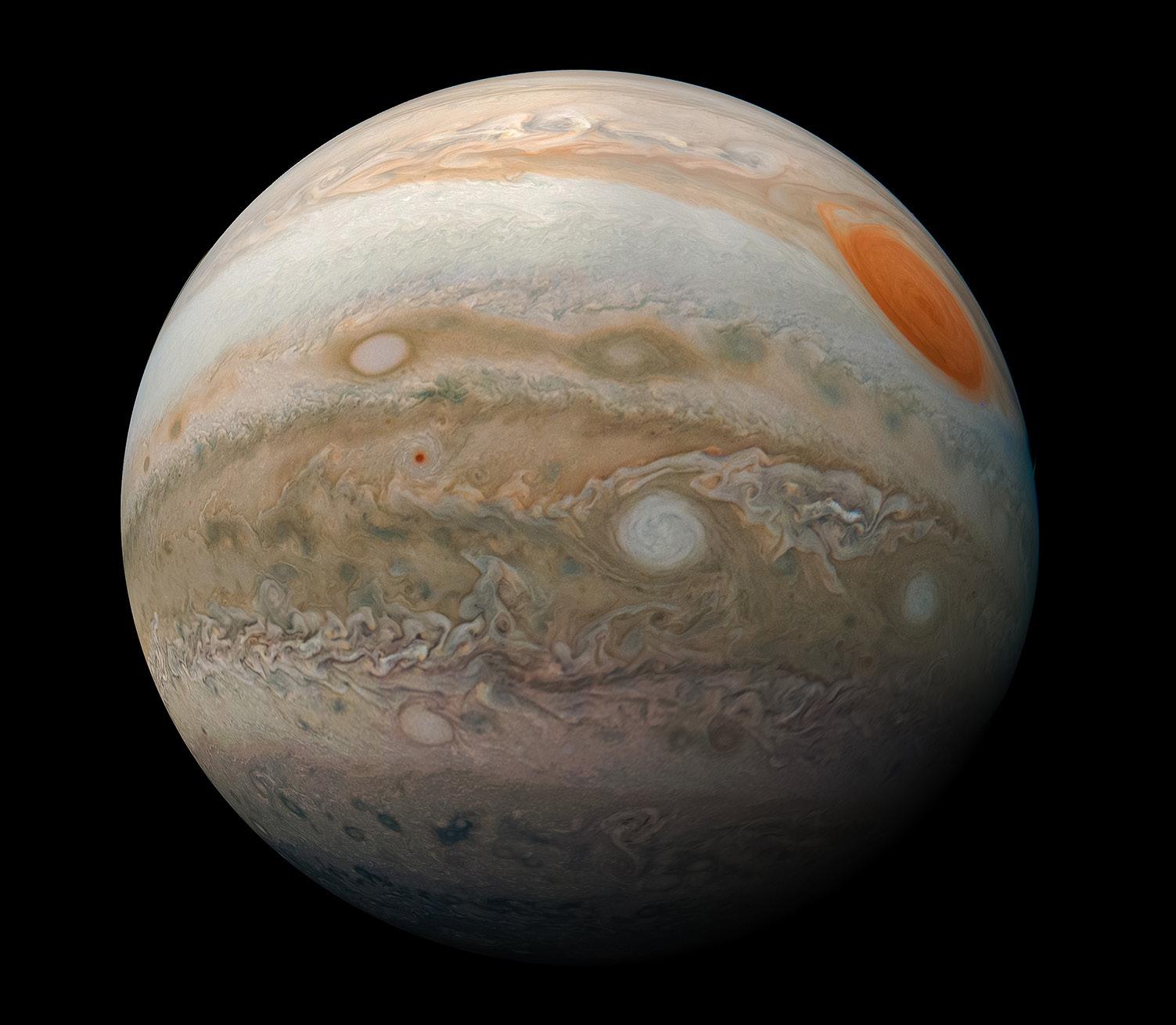
JUICE is ESA’s upcoming foray into the depths of the solar system, and it will focus on one of the major questions in planetary science and astrobiology: is there liquid water at Jupiter’s moons? And then, the crucial follow-up question: if there is water, could it support life? Life as we know it needs water. In the past, we thought that Earth was special because of its position in space. If our planet was closer to the sun, the water would evaporate and, if it were further away, it would freeze. This location is known as the ‘Goldilocks zone’ because, just as the preferences of the light-fingered little girl in the fairy tale, it is neither too hot nor too cold. Until recently, Mars had been the leading contender for finding life elsewhere in the solar system (see page XXX). Other scientists have pinned their hopes on planets outside our solar system but similarly in a Goldilocks zone around a star. But now icy moons have also emerged as prime candidates for finding liquid water, especially after the discovery of geysers of water vapour on Jupiter’s Europa, as well as on Enceladus, a moon of Saturn. So, JUICE will be flying by Ganymede, Callisto and Europa to understand what lurks beneath their surfaces.
PAGE 90: An image of Jupiter captured by Juno in February 2022. The dark shadow on the left was cast by Jupiter’s moon Ganymede. Image data: NASA/ JPL-Caltech/SwRI/MSSS. Image processing by Thomas Thomopoulos © CC BY OPPOSITE: An image of Jupiter’s Great Red Spot created using data from Juno’s JunoCam imager in 2019. NASA/ JPL-Caltech/SwRI/MSSS/Kevin M. Gill LEFT: A Juno-captured image of a cyclonic storm over Jupiter. Image data: NASA/JPL-Caltech/ SwRI/MSSS. Image processing by Kevin M. Gill, © CC BY
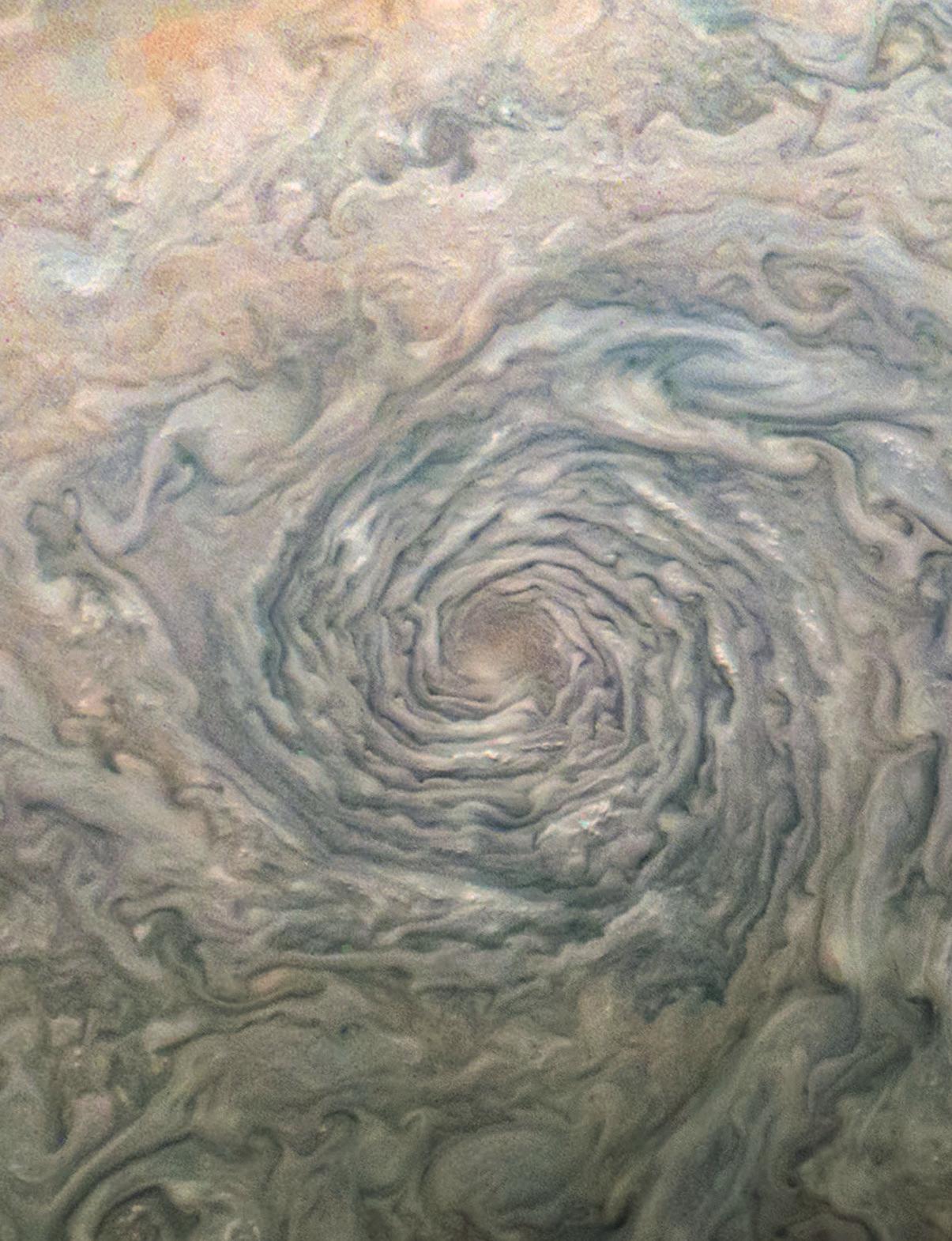
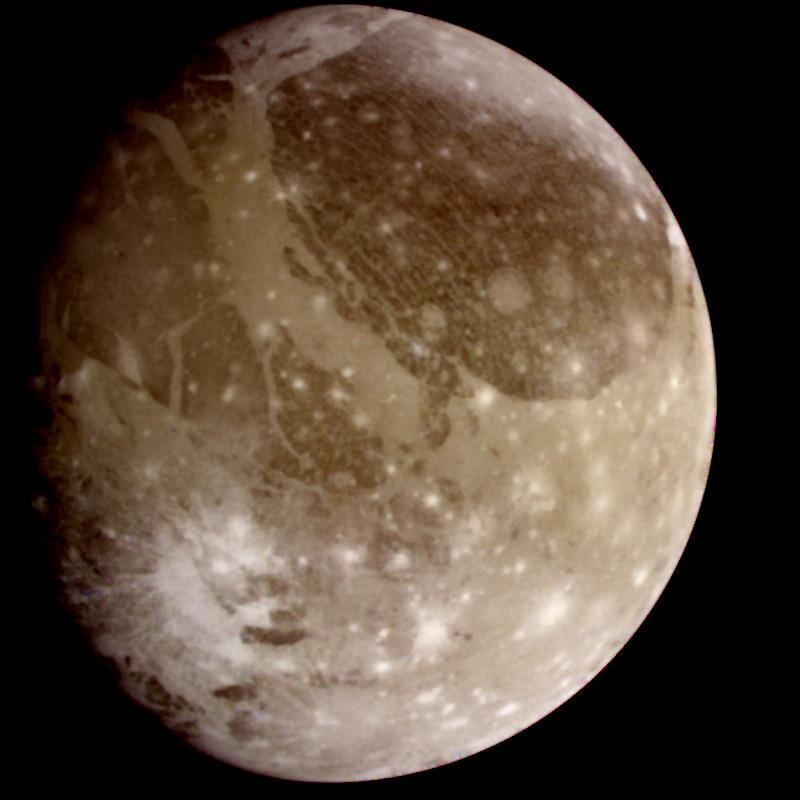
DEATH STAR
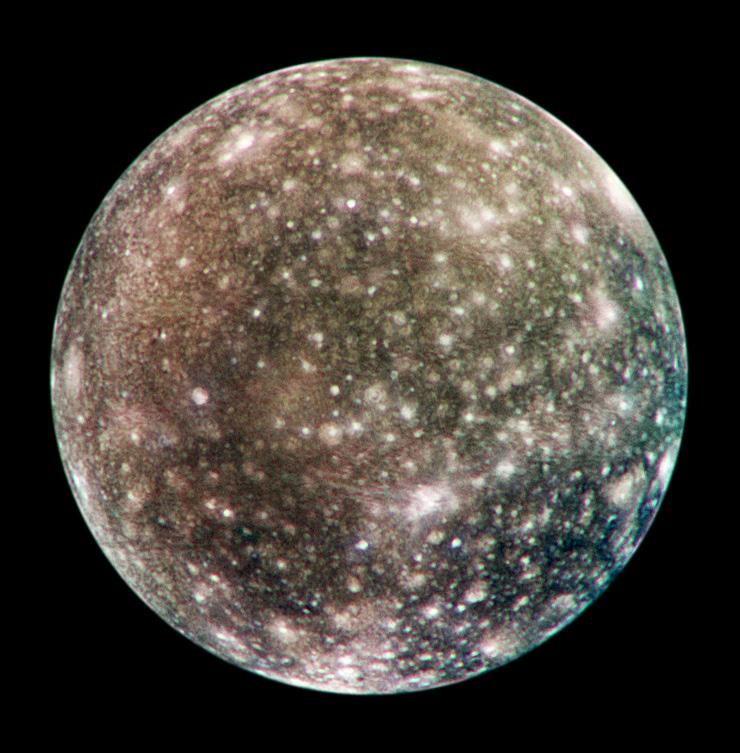
And each of these worlds is fascinating, mysterious and downright weird in its own right.
Ganymede, the solar system’s largest and most massive moon, disconcertingly looks like the Death Star from Star Wars. Its mottled brown and white surface is covered with impact craters from other smaller (but speedy) objects hitting it. Ganymede is the only moon with its own internal magnetic field, which has scientists looking at this large pale moon with a great deal of interest.
Meanwhile, Callisto orbits Jupiter like a dirty disco ball, with thousands of white craters sparkling against a dull rainbow of colour. Its surface is the oldest and most densely cratered in the solar system, and it could offer a unique perspective on how Jupiter’s large moons formed billions of years ago.
TOP LEFT: A natural-coloured view of Ganymede, taken from the Galileo spacecraft in 1998. NASA/JPL BOTTOM LEFT: The scarred surface of Jupiter’s moon Callisto, in an image taken by Galileo. NASA/JPL/DLR OPPOSITE: Jupiter’s icy moon, Europa. NASA/JPL-Caltech/ SETI Institute
Europa, which is slightly smaller than our own moon, resembles a giant scratched marble, and has the smoothest solid surface in the solar system. Scientists have confirmed the existence of water vapour on this moon and suspect a giant salty ocean lurks beneath an icy crust.
These moons are giving scientists ideas about other locations where they should be hunting for water. ‘So now there’s this vast region of space where you could potentially find life,’ explains Dougherty.
JUICE, with its array of scientific instruments, is ready to investigate Jupiter and these three icy moons. But first it has to get there in one piece.
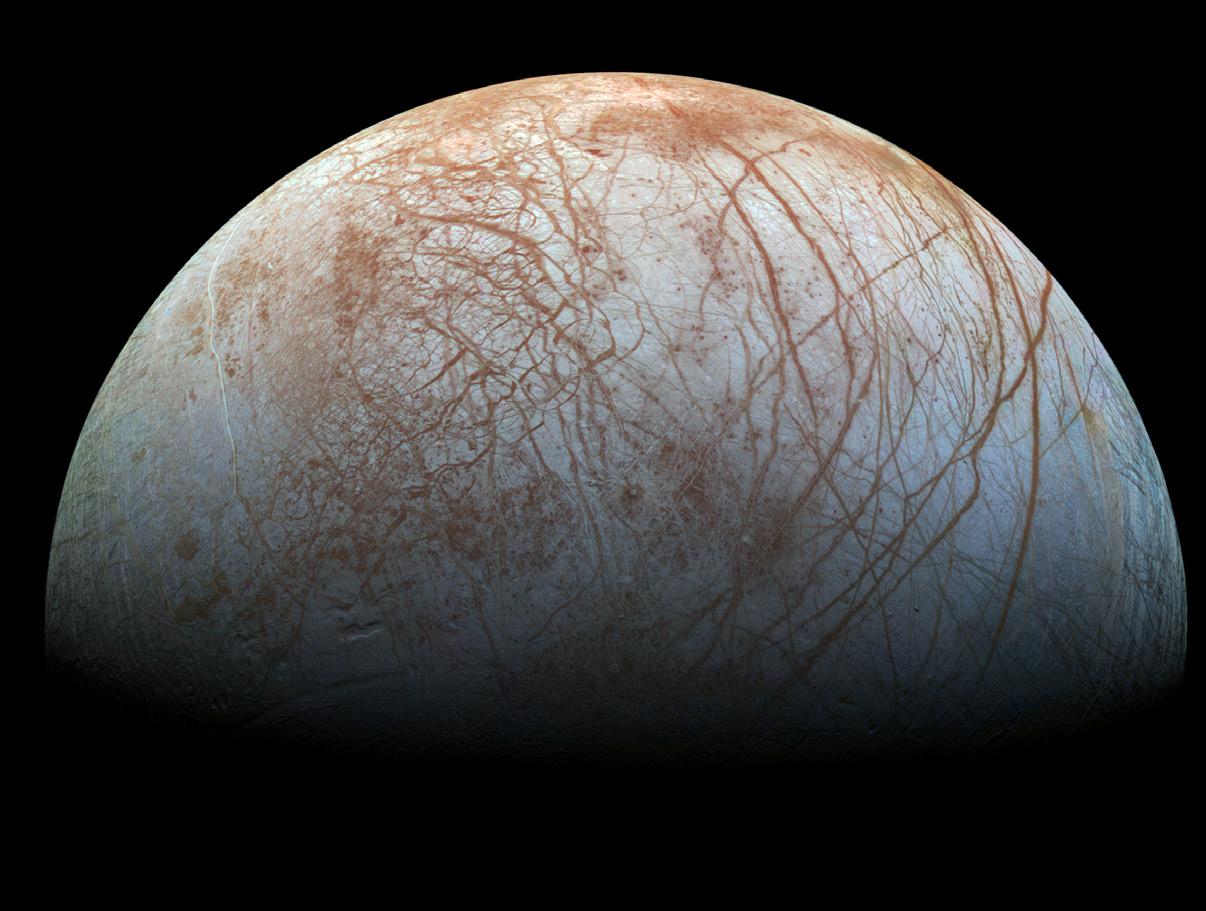
SPACE BUTTERFLY
BELOW: An artist’s impression of JUICE in its travels around the Jupiter system. © Airbus OPPOSITE: Testing the solar array ‘wings’, June 2022. © Airbus 2022 JB Accariez – Master Films
The space probe will not have enough fuel to make it to Jupiter; it will need more energy. So, instead of a direct flight to the solar system’s largest planet, JUICE’s eight-year journey will first involve flying by Earth, Venus, and Earth, before a final pass around Earth again. It’s a bit like swinging a rock at the end of a rope to make it go faster: JUICE will fly past planets in the solar neighbourhood, gaining energy, before its operators release the hypothetical rope and slingshot the spacecraft towards its final destination.
JUICE looks like a giant space-bound butterfly. It has a central, shielded and insulated trunk; numerous antennas sprouting from the body, as well as an almost 11m (36ft) boom; and two giant solar ‘wings’ unfurled on either side. These look like massive crosses, each about the size of the front of a bus. They will be vital for harnessing the sun’s power from Jupiter’s distant neighbourhood, where solar energy is around twenty-five times weaker than on Earth.
‘There are many individual technical requirements that we have faced before on previous missions,’ says Cyril Cavel, project manager for Airbus, the company that built the spacecraft. ‘What makes JUICE so special is that, for the first time, we accumulate all of these design challenges on one single mission.’
For example, the spacecraft will endure a wide range of temperatures, from the toasty environs of Venus – around 230°C (446°F) – to chilly Jupiter’s -220°C (-364°F).
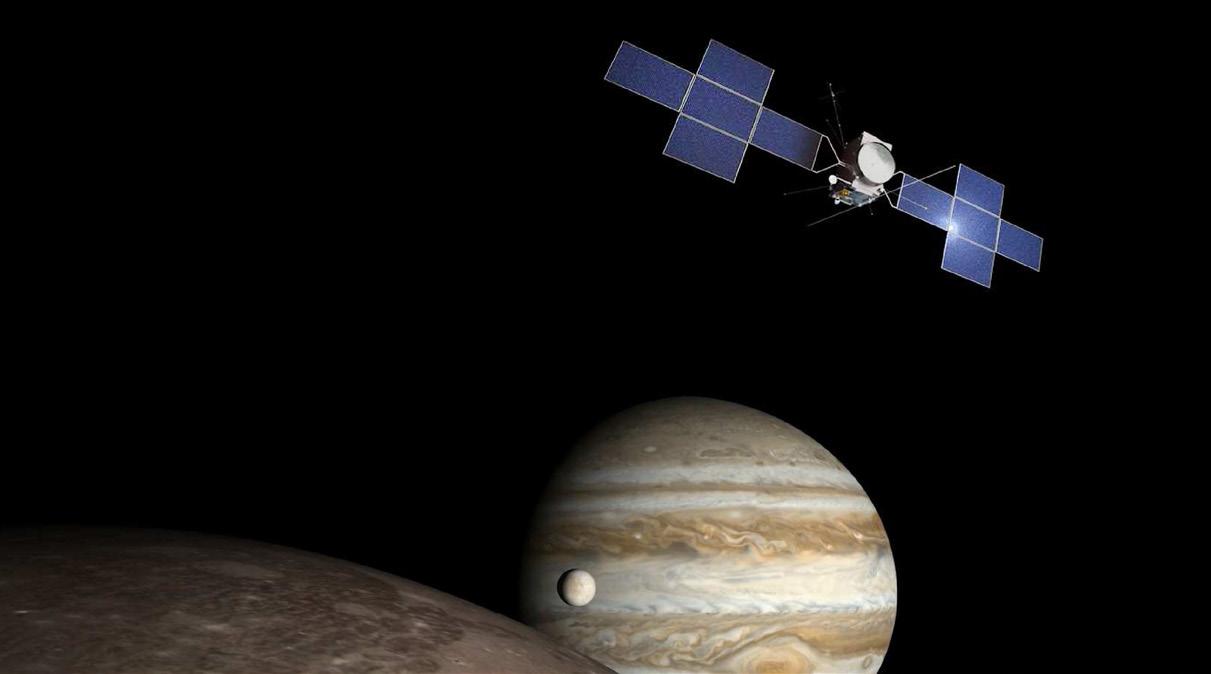
INTENSE RADIATION
Jupiter has the most intense radiation of any planet in the solar system because of its super-strong magnetic field – and this radiation will eat away at all exposed external surfaces. Added to the environmental challenges, some of the signals that the instruments want to measure, such as the magnetic field within the oceans of Ganymede, are very weak. This means that the spacecraft’s electric and magnetic fields must not interfere with the signals that the instruments are trying to detect. To get around these difficulties, JUICE’s body is swaddled in insulating material and shielded with lead foil, and the most sensitive instruments are stuck at the end of the spacecraft’s boom – much like a microphone on a boom on a movie set. There are also many points at which the spacecraft will be out of communication with Earth, deaf and blind to scientists’ instructions, so a lot of its functions – including self-diagnostics and problem-solving – are autonomous. In case something does go wrong, about half of the 6-tonne spacecraft’s weight is taken up with spares. ‘We have back-ups almost everywhere,’ says Cavel, adding that even where there aren’t back-ups, a plan can be made. ‘There is only one engine, and even if it fails, we have other thrusters to complete the mission.’
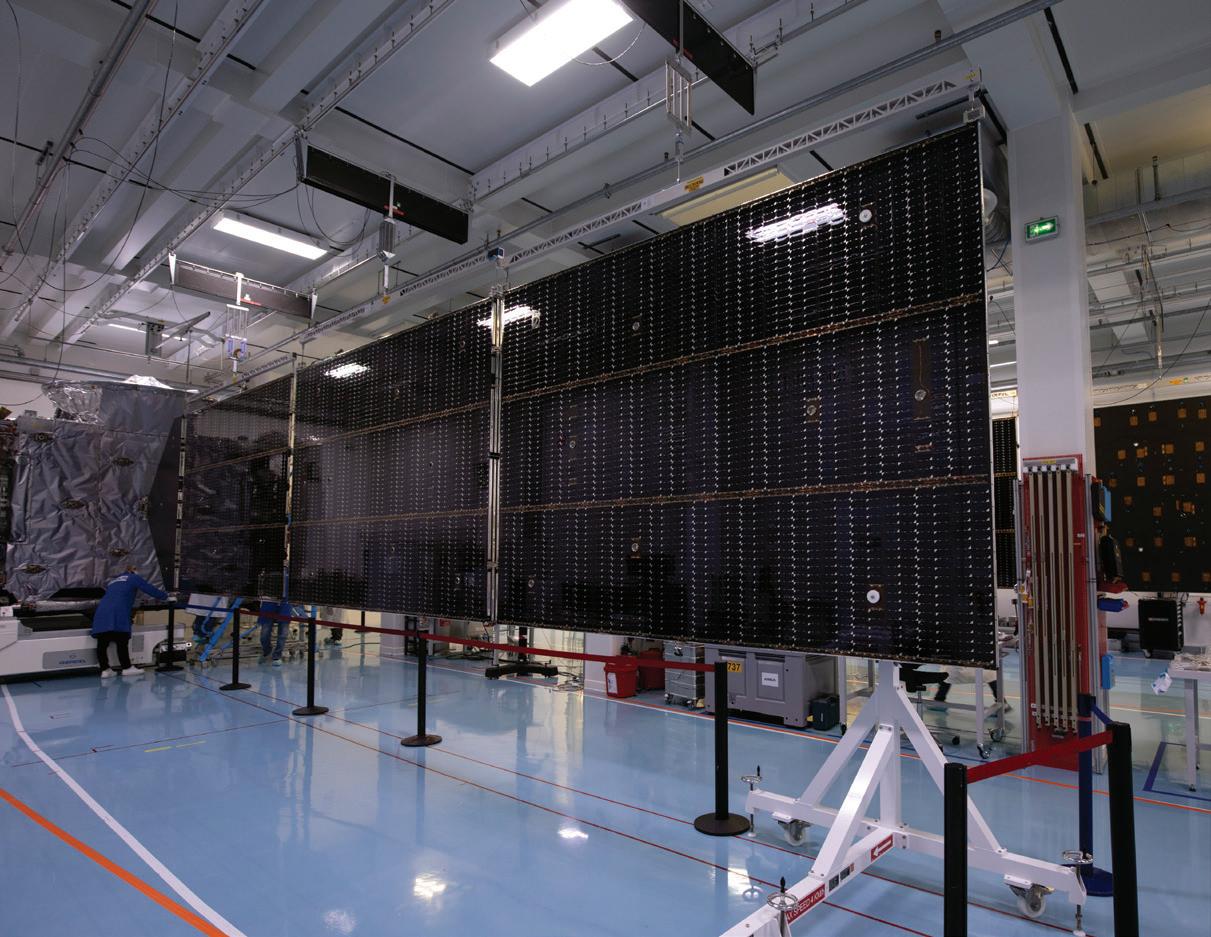
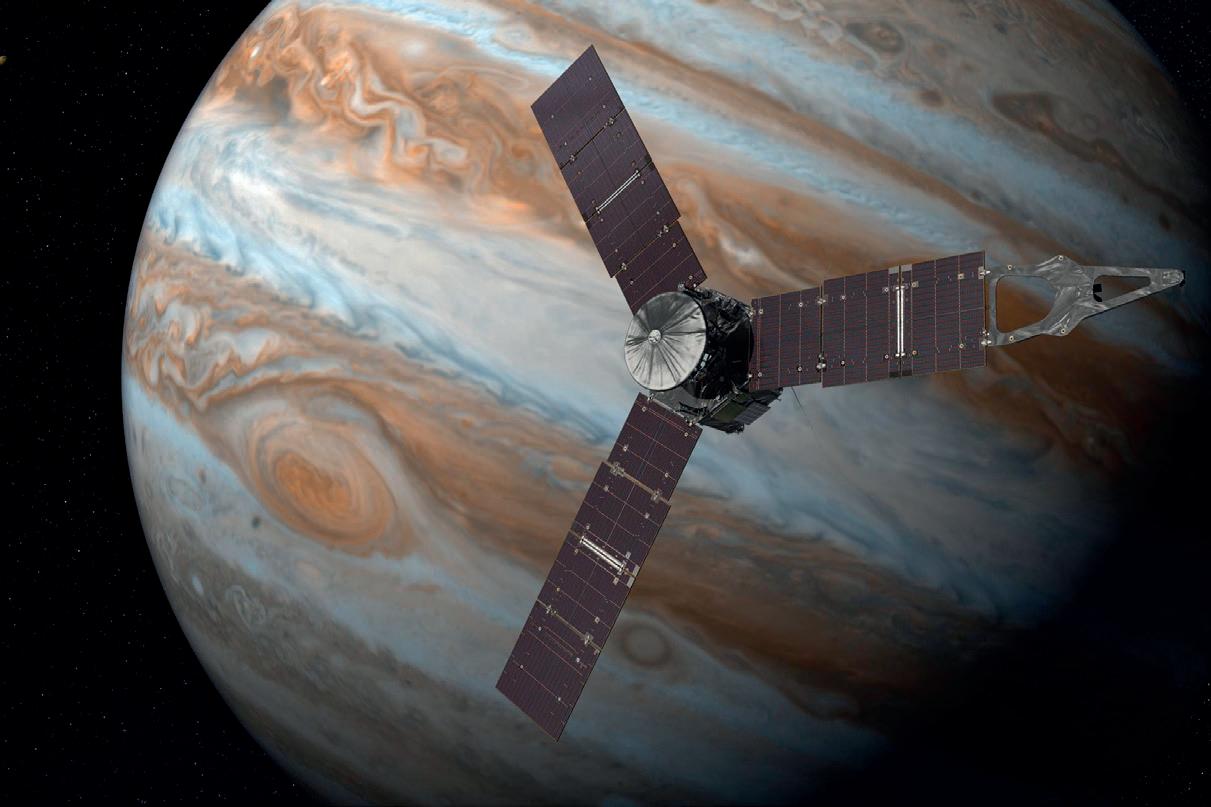
LIQUID WATER
When it finally arrives at Jupiter in 2031, JUICE will be building on the legacy of past missions, beginning with NASA’s Pioneer and Voyager probes in the 1970s. However, there have been only two previous dedicated missions to the gas giant: the 1989 Galileo probe and Juno, which arrived at Jupiter in 2016 and has been sending back spectacular images ever since. Juno is now on an extended mission until 2025, having completed its prime mission of thirty-five orbits of the planet ESA’s JUICE is fitted with ten instruments, some of which will be able to capture incredible images, from ultraviolet wavelengths to sub-millimetre wavelengths, as well as an optical camera that will show us what these surfaces look like to the human eye. The other instruments will collect as much information as the latest technology can offer: a radar to penetrate the crusts of three of its moons, and a spectrometer to hunt for chemical signatures and understand what the landscapes are made up of. Another tool will decipher their atmospheres, and others will work to unpick their magnetic fields. ‘We have selected the instruments to make sure that they work together, and we need all of them to answer the questions,’ says Olivier Witasse, JUICE project scientist at ESA. ‘We are going to be studying everything.’ And he really does mean everything – how Jupiter and its moons interact, the composition of their crusts, their atmospheres, their magnetic fields and how these fields interact, among other things. However, there is one area of study that stands out. ‘The most important part is to understand this liquid water. Is it 10 kilometres below the surface or 40, 50? We need to know to prepare for the next exploration.’ Jeffrey Kargel, a senior scientist at the Planetary Science Institute in Arizona, is excited to find out what the spectrometer will say about Europa’s ocean, particularly the salts it contains – which could be the difference between a balmy ocean where life could possibly flourish and acidic icy water in which most life would struggle to survive. In an arrangement as strange as Jupiter’s – with its wobbly magnetic field, entourage of seventy-nine moons and the tantalising prospect of liquid water – scientists are particularly interested in data about the magnetic fields that permeate the Jovian system, in part because this can provide answers that other metrics can’t. ‘Magnetic fields describe the entire plasma environment,’ explains Dougherty, who was principal investigator of the magnetometer on NASA’s Cassini mission to Saturn. Plasma is a superheated gas, and makes up most of the universe. ‘But they also allow you to see inside planetary bodies.’
Her team is focusing on the magnetic field in Ganymede’s ocean, but that is a big ask: they need to be able to detect the ocean’s tiny magnetic field (about a nanotesla) against the background magnetic field of the rest of the moon, as well as Jupiter’s ever-changing field – which is more than a billion times stronger. ‘When I lose sleep at night, it’s about whether we’re going to be able to resolve the ocean signature of Ganymede,’ she says.
They will spend the next decade developing the computational models to separate the whisper of Ganymede’s oceanic magnetic field from the background roar. But Dougherty will have to be patient, something which she admits does not come easily to her. JUICE’s Ganymede gallivant is at the end of the mission – right before the spacecraft deliberately ploughs into the surface of that moon in 2035. That way, scientists will know where the craft crash-lands and make sure it doesn’t smash into a planet where there may be life. As a bonus: you also learn a lot of things about a planet’s surface when you hurl a heavy spacecraft at it.
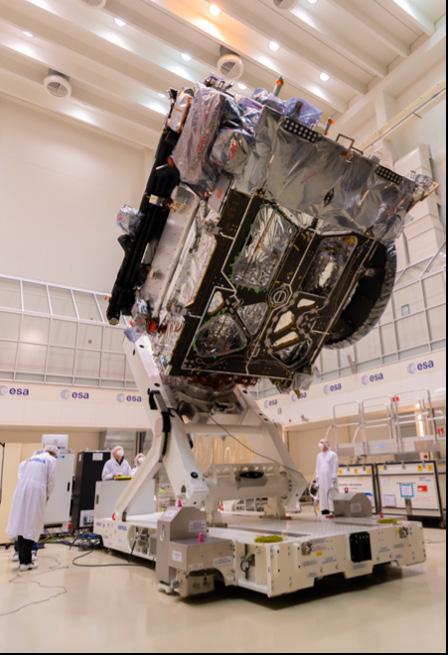
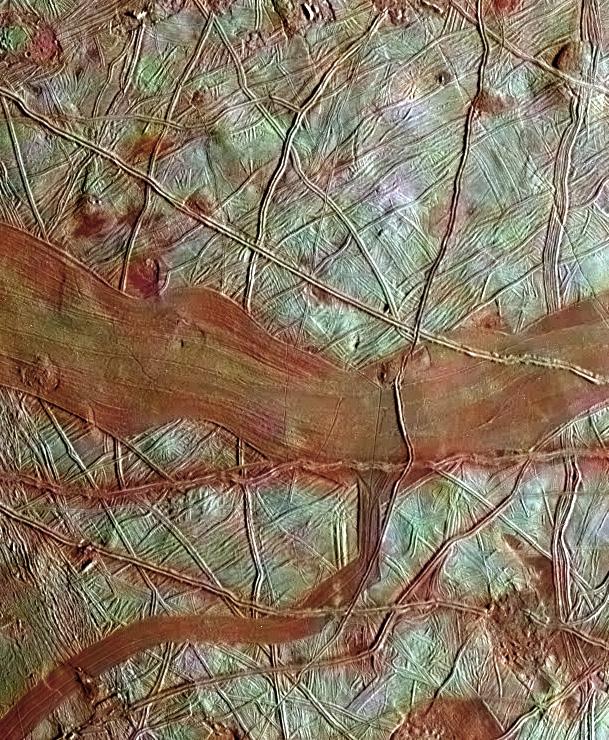
OPPOSITE: An artist’s rendering of Juno in its elliptical orbit. NASA/JPL-Caltech ABOVE LEFT: JUICE being worked on at the European Space Research and Technology Centre in the Netherlands in April 2021. ESA-SJM Photography ABOVE RIGHT: The cracked surface of Europa – does an ocean lurk beneath its icy crust? NASA/JPL-Caltech/SETI Institute
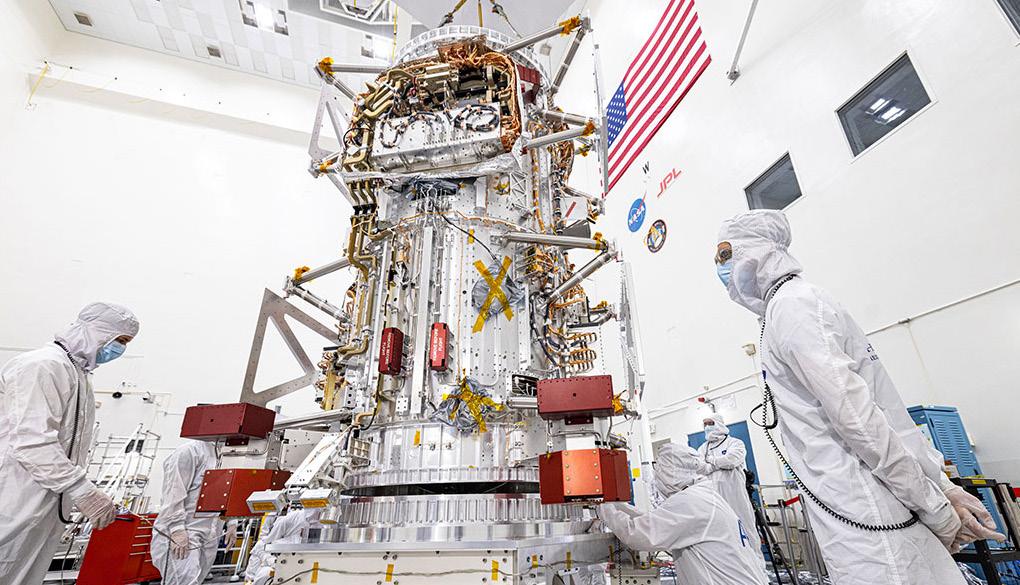
ABOVE: Europa Clipper mission completes the main body of the spacecraft. NASA/JPL-Caltech/Johns Hopkins APL/Ed Whitman BELOW: The main body of the Europa Clipper spacecraft being transported to the NASA Jet Propulsion Laboratory in California. NASA/JPL-Caltech/Johns Hopkins APL/Ed Whitman OPPOSITE: The solar system’s largest moon, Ganymede, captured alongside Jupiter by the Cassini spacecraft in 2000. NASA/JPL/University of ArizonaABOVE: NASA’s
WILD AND COOL
Even as JUICE is poised to change our understanding of the solar system and directly detect liquid water, planetary scientists and engineers already have their eyes on the next mission. ‘In the same way that Voyager 1 and 2 pointed to Galileo, and Galileo pointed the way to JUICE,’ says Kargel. Hot on JUICE’s heels, NASA’s Europa Clipper mission is due for launch in 2024. ‘We are very happy that there will be two spacecraft at the same time because, with two, you can do joint stuff and it’s great,’ says ESA’s Witasse. The JUICE mission will only make two flybys of Europa, which will comprise about 5 per cent of its science, whereas Europa Clipper will focus solely on Europa and the possible water beneath its icy crust. For Kargel, all the data from JUICE and then Europa will pave the way for the next step in planetary exploration. ‘We’ll be able to detect how thick the ocean is, how dense the ocean is, and how salty it is,’ he says, speaking faster. ‘We’ll then be able to determine if we ever have any hope of sending a probe down into the ocean – because that would be the ultimate, wild, cool thing to do.’ As long as nothing goes terribly wrong during the wild ride of launch.
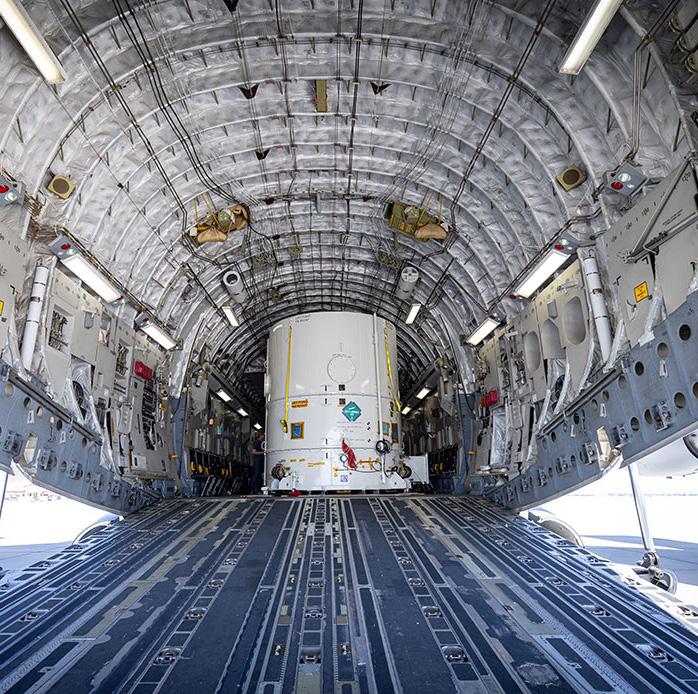
JUPITER AT A GLANCE
The first thing to know about Jupiter is that it is giant, really giant. It is twice as massive as all the other planets in the solar system combined. It’s also a gas giant, which is a large planet mostly made up of hydrogen and helium. Our solar system has two gas giants – Jupiter and Saturn – and, unlike Earth, they do not have hard surfaces. They have solid cores surrounded by swirling gases.
Jupiter looks like it has cream, orange and brown stripes, which are caused by windy clouds of ammonia and water vapour that float in a firmament of hydrogen and helium. It has a giant red spot that is bigger than Earth but is actually a mammoth storm that has rampaged for centuries.
A swarm of moons surrounds Jupiter, almost eighty of them, and while Jupiter’s tumultuous exterior cannot support life, many of its satellites may be able to. Like Saturn, Jupiter has rings, but they are much fainter than Saturn’s and made of dust, not ice.
So far, nine spacecraft have visited Jupiter. Seven flew by and two have orbited the gas giant. NASA’s Juno mission, the most recent, arrived at Jupiter in 2016.
There are a number of trips planned to our largest planet. As well as JUICE and NASA’s Europa Clipper mission, China is also eyeing Jupiter and talking about launching a space probe. If JUICE finds proof of liquid water on Jupiter’s icy moons, we may see even more visits to our Jovian neighbour.
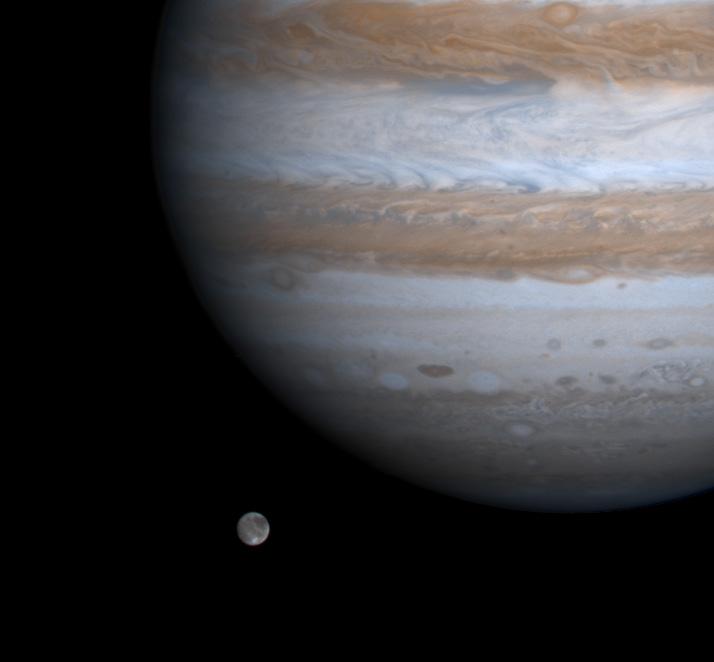
ASK DR BECKY
What’s at the centre of the universe? What’s inside Mars? How long would you survive in space without a suit? The Supermassive Podcast’s resident astrophysicist, Dr Becky Smethurst, tackles your big questions on the nature of the cosmos.
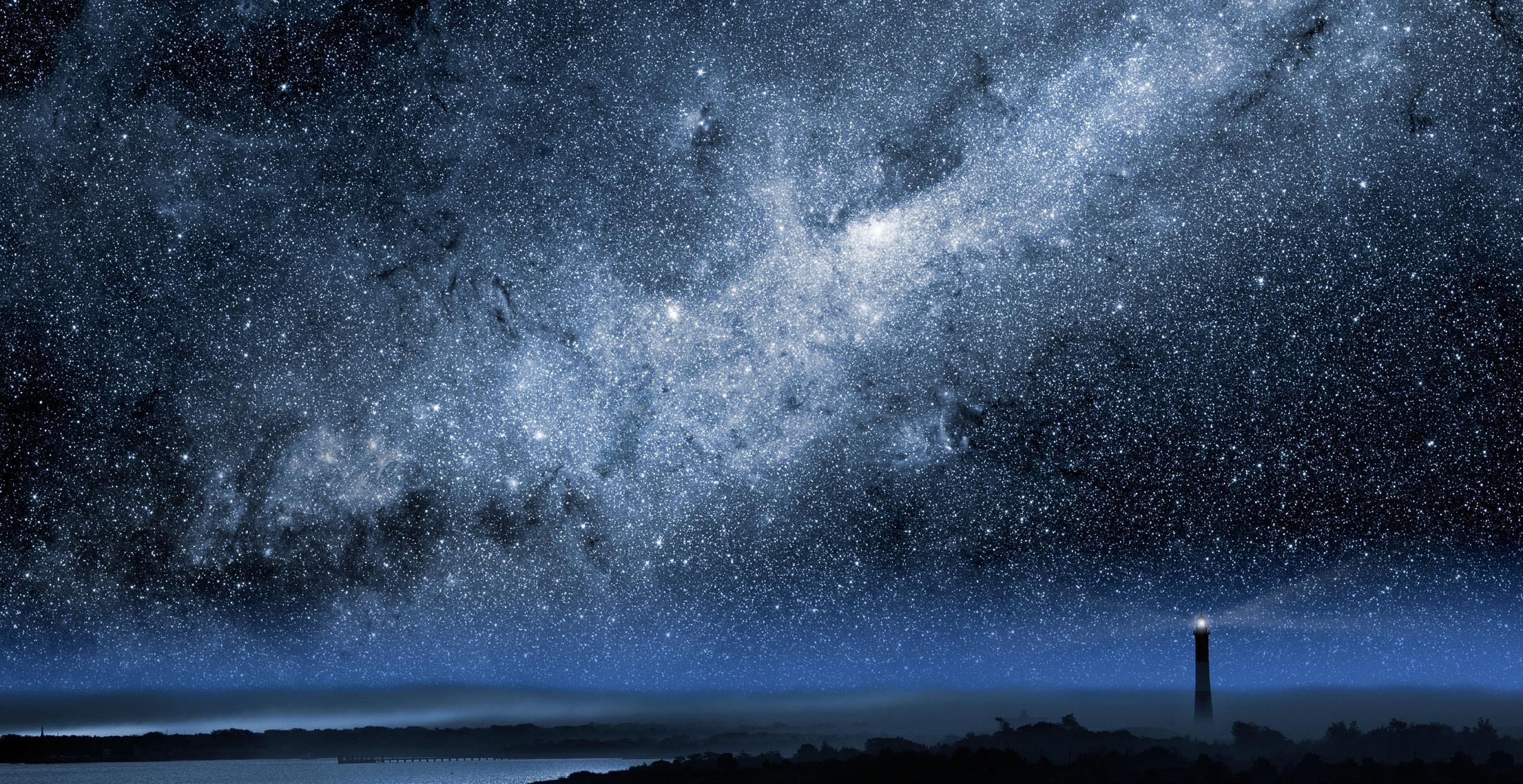
We now have images of two different black holes. But we’re told that light can’t escape black holes, so how do we get pictures of them?
The Event Horizon Telescope has recently captured images of the supermassive black hole at the centre of the Messier 87 galaxy and our own galaxy, the Milky Way. Although we can’t see the actual black holes, the telescope is so powerful that it’s been able to detect the light coming from the material spiralling around them. As hydrogen gas gets ever closer to black holes, the extreme gravity accelerates it to tremendous speeds. This gives the gas molecules more energy so that they also become hot and start to glow. The bigger the A dramatic view of the Milky Way over Fire Island, New York. B.A.E. Inc./Alamy
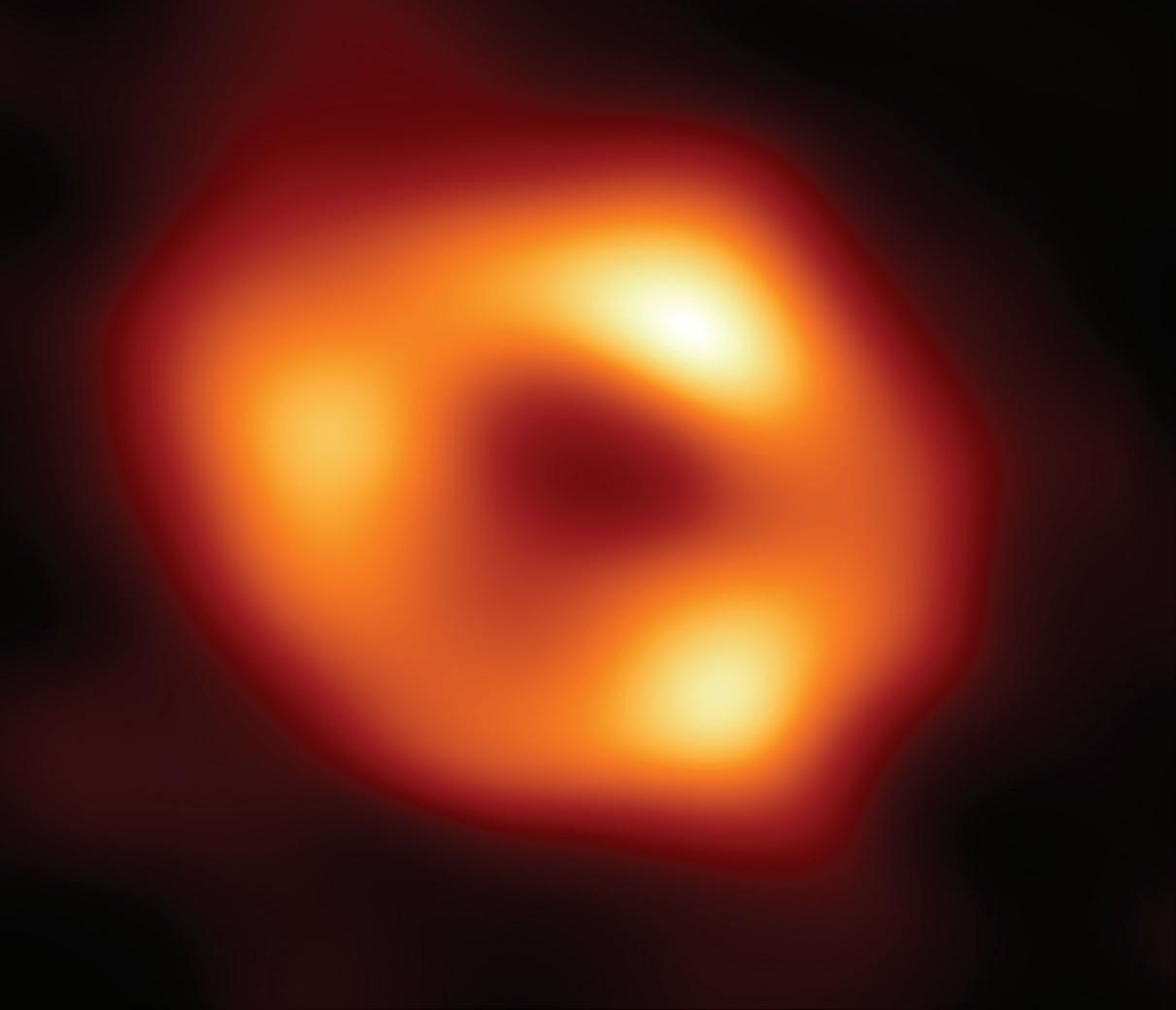
black hole and the more hydrogen gas there is, the brighter the surrounding regions surrounding the black hole will be. This light covers the entire electromagnetic spectrum, from X-rays and ultraviolet to visible and infrared. But the region in the very middle of this turbulent and hot material is the black hole itself, from which light cannot escape. So, while we see the ring of material spiralling around it, we also see the ‘shadow’ of the black hole in the middle. The black on the outside of the ring is empty space, whereas the black on the inside of the ring is where so much matter has been squished into such a small space that not even light can escape. The Event Horizon Telescope is made up of radio telescopes around the world. Radio telescopes can be combined in such a way that they mimic a telescope that is the size of the Earth (see page 000). Previously, we only saw the centre of galaxies as a fuzzy blob but now we can resolve that blur into a ring. The bright blobs in the ring are called hotspots. This is where there is a slightly denser patch of material swirling around the black hole and therefore giving off more light. Although at first glance these images just look like blurry, blobby doughnuts, they reveal so much about the nature of black holes and the strength and behaviour of gravity around them. They will allow us to do detailed tests on our theories of gravity to work out if we’ve missed something in the bigger picture of how the Universe works.
How much time will it take for the James Webb Space Telescope (JWST) to collect enough data for a single image?
It depends on what JWST is looking at. Bright objects close by, such as the planets in our solar system or nearby bright stars, won’t need much exposure time before enough light is collected above the background noise to get a clear image. However, something fainter that is much further away – such as a distant galaxy billions of light-years from the telescope – will need a much longer exposure time. To give you a rough idea how long JWST will take to collect enough light, let’s compare it to its predecessor, the Hubble Space Telescope. Back in 2003, Hubble took one of the most detailed images ever of a tiny patch of sky, which is now known as the Hubble Ultra Deep Field. It took Hubble a total combined time of just over 1 million seconds, or eleven and a half days, to collect enough visible light from the faintest and most distant objects. It then stared at the same patch of space detecting infrared light which took a total observing time of 200,000 seconds, or around another two and a half days. The JWST also detects infrared light and has some overlap in wavelength range with Hubble. However, because the JWST has a much bigger mirror and can therefore collect more light, an exposure time of two and a half days on Hubble will take around three hours with Webb. OPPOSITE: The stunning first image of the supermassive black hole at the centre of our Milky Way galaxy, released in May 2022. EHT Collaboration BELOW: A JWST alignment image released in May 2022. This shows part of the Large Magellanic Cloud, in a close-up from JWST’s Mid-Infrared Instrument test image. NASA/ESA/CSA/STScI
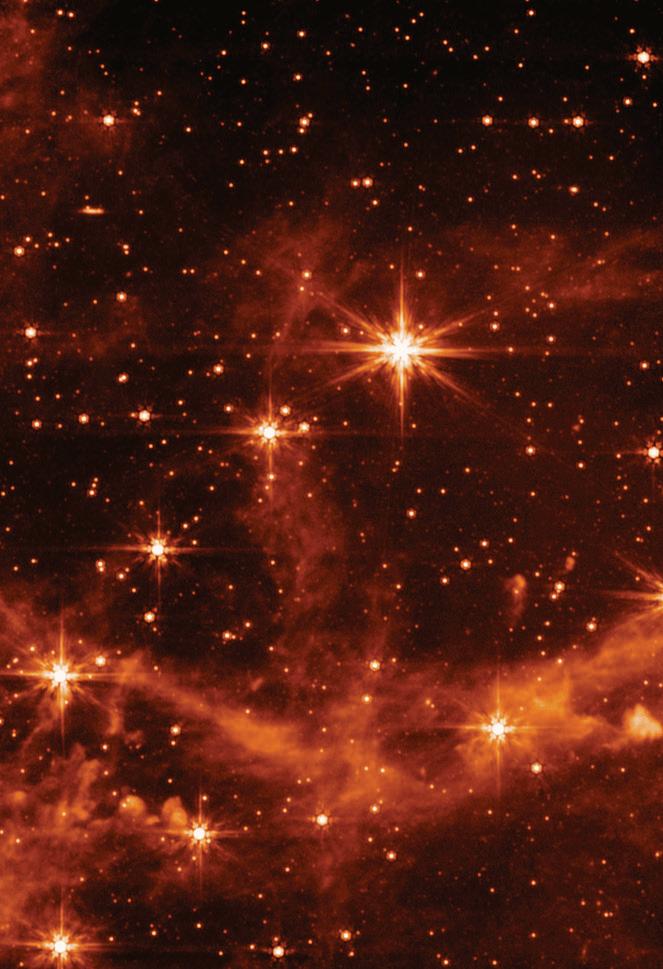
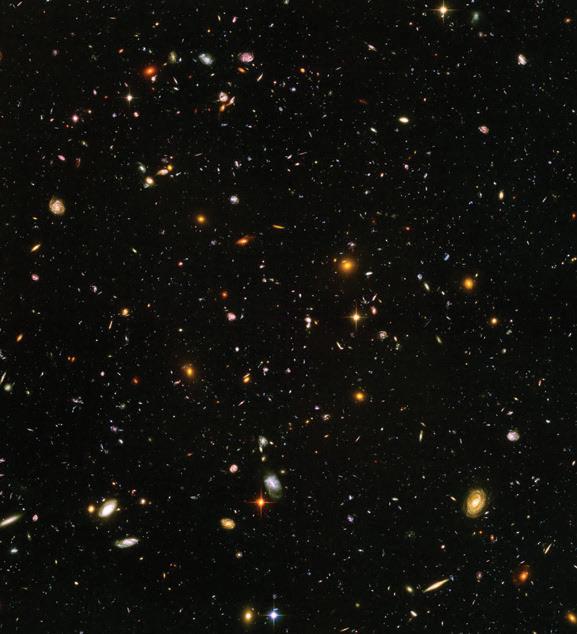
That’s the good news. But getting time on telescopes is an incredibly competitive process. Most telescopes are oversubscribed by a factor of five to ten compared to how much time is available to use them.
When we astrophysicists put together an observing proposal to request to use a telescope, we employ what is known as an ‘observing time calculator’ tool. These calculators let you input the brightness of the object you intend to observe, along with its coordinates and a target ‘signal to noise ratio’ that you want to achieve. They then output an estimate for how long you will need to look at an object to get a decent image. With that information to hand, you can tot up how much time you will need on the telescope to do your science and hope your application is successful.
I’m confused about the idea of an expanding universe. We are told that our neighbouring galaxy, Andromeda, is going to collide with the Milky Way. How is this possible if everything is expanding away from everything else?
Space is expanding, but objects like galaxies are not in fixed positions in space. The expansion of space is a never-ending battle between the expansion outwards and gravity pulling inwards. When objects are close together, gravity dominates over the expansion. This is why the Earth is not getting further away from the Sun as space expands; it’s held in orbit by gravity as space expands around it. The same is true for the billions of stars, gas and dust held together in galaxies, such as in the Milky Way.
However, when objects are further apart from each other, such as the billions of lightyears that separate galaxies, gravity is weaker and the expansion dominates, expanding the space between them and causing them to get further apart. The majority of galaxies we see in the sky all appear to be moving away from us due to the expansion of space between us and them. The Andromeda galaxy, however, bucks that trend.
Andromeda is our closest massive neighbour galaxy at roughly 2.5 million light-years away, and it contains over a trillion stars – many more than the Milky Way’s roughly 100 billion stars. The gravitational attraction between the Milky Way and Andromeda is strong enough to pull them closer together despite the expansion of space between them. It means that in roughly 2–4 billion years’ time, the two will merge together to become a much larger blob of a galaxy, dubbed Milkomeda.
In this forthcoming merger, it’s very unlikely that any two stars will collide, since there’s just so much space between them. The nearest star to the Sun is four lightyears away, or 38 trillion kilometres (23.6 trillion miles), whereas the stars themselves are only between 1 and 10 million kilometres (0.6–6.2 million miles) wide. You could fit 27 million Suns end to end in the distance between it and its nearest star. So, the odds of any two stars coming close enough to collide is vanishingly small just because of the huge scales involved.
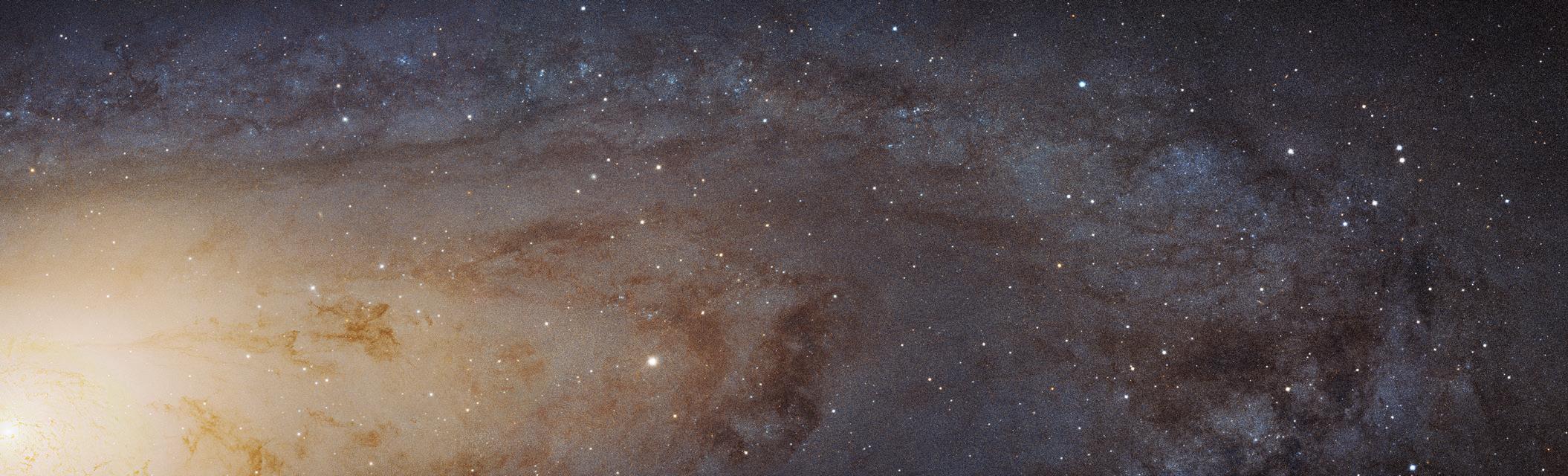
OPPOSITE: The Hubble Ultra Deep field – a view of nearly 10,000 galaxies taken in 2003. The smallest, reddest galaxies may be among the most distant known, existing when the universe was just 800 million years old. NASA, ESA, and S. Beckwith (STScI) and the HUDF Team LEFT: A bird’s-eye view of part of the Andromeda galaxy taken by Hubble in 2017. It is the sharpest image ever taken of our galactic next-door neighbour. NASA, ESA, J. Dalcanton, B.F. Williams, and L.C. Johnson (University of Washington), the PHAT team, and R. Gendler
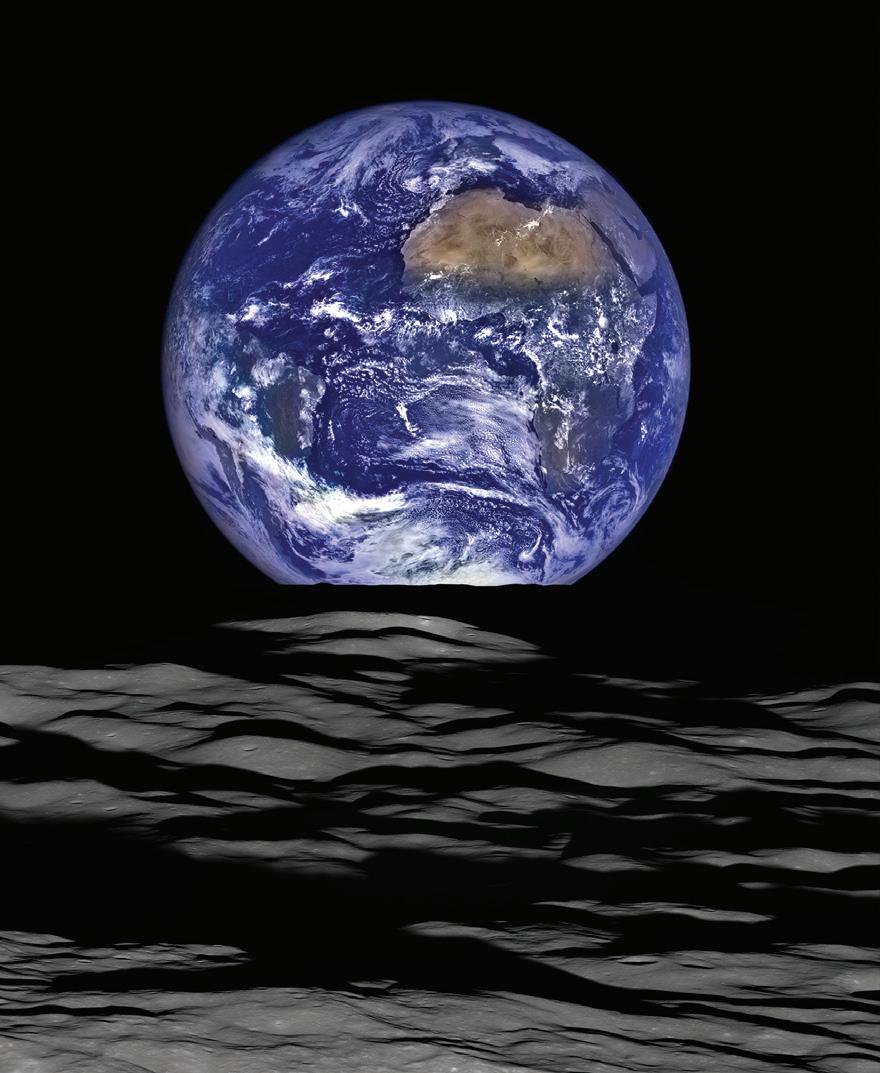
LEFT: A unique view of Earth taken from the vantage point of the Moon, captured by NASA’s Lunar Reconnaissance Orbiter. NASA/Goddard/Arizona State University BELOW: The PDS 70 system, located nearly 400 light-years away, featuring a star at is centre and at least two planets orbiting it. The exoplanet PDS 70c is surrounded by a ring of debris (the dot to the right of the star) that could form into an exomoon. ALMA (ESO/ NAOJ/NRAO)/Benisty et al.) OPPOSITE: An artist’s impression of WASP-12b, the hottest known exoplanet in the Milky Way with a temperature of 2,500oC (4,600oF). The planet is so hot it has puffed up to the point where its outer atmosphere spills onto the star. NASA, ESA, and G. Bacon (STScI)
Have we found any exoplanets with moons, and are they comparable to the size that the Moon is to Earth?
So far, we only have twenty candidate exomoons. These are moons in orbit around planets beyond our solar system. However, we only have a direct image of one of them. Scientists have proposed the existence of the others to explain data collected by telescopes, such as the Kepler space telescope, observing the brightness of stars.
If an exoplanet passes in front of a star during its orbit, the brightness of the star will dip by a tiny fraction, which we can then detect. If there is also a moon in orbit around that exoplanet then it will cause a secondary dip in the brightness. From how much the brightness of the star dips for the pass of each object, we get an estimate of how big the exoplanet and exomoon are. But even if we detect these secondary dips in the brightness of the star, there could be other explanations for that change. In 2021, astronomers working with the ALMA array of radio telescopes claimed to have taken an image of an exomoon forming around the exoplanet PDS 70c. Although it just looks like a fuzzy blob, the astronomers managed to model the light detected and suggested there was a ring of debris around the exoplanet that could form into an exomoon. If you’re looking for a planet–moon system most like our own, you are going to have to be patient for a while longer yet. With only a handful of candidate exomoons, it’s difficult to make any concrete claims for their size relative to their planets. Our Moon is around 1.2 per cent the mass of Earth, and there have been a few exoplanets with masses larger than Jupiter that have candidate exomoons with a similar mass ratio. Astronomers have, for example, suggested that WASP-12b, which is an exoplanet roughly one and a half times the mass of Jupiter but which orbits very close to its star, has an exomoon that could be up to 6.4 times the mass of the Earth, putting the mass ratio of 1.4 per cent at a similar value to the Earth–Moon system.
The idea of exomoons around massive gas giant exoplanets close to their stars is an intriguing one for the existence of life beyond Earth. If the exomoon is rocky and found in the habitable (or Goldilocks) zone around the star (not too hot and not too cold), then the conditions for life could be just right. Given how many ‘hot Jupiters’ we have detected (Jupiter-sized planets orbiting closer to their stars), it could be that the majority of life in the Universe is found on moons, rather than planets.
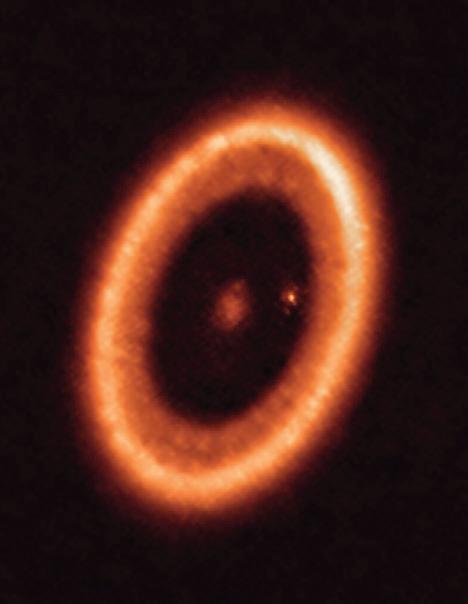
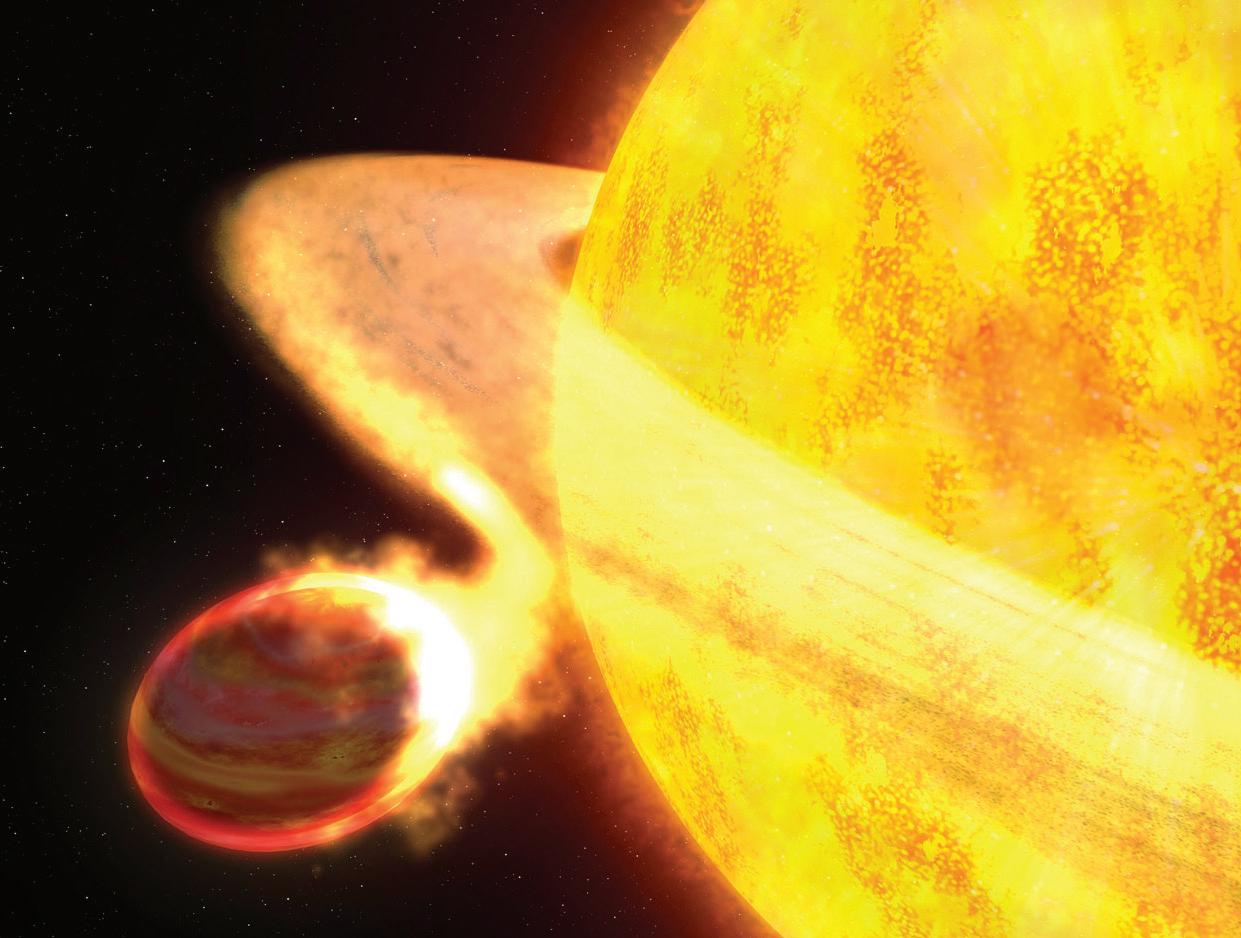
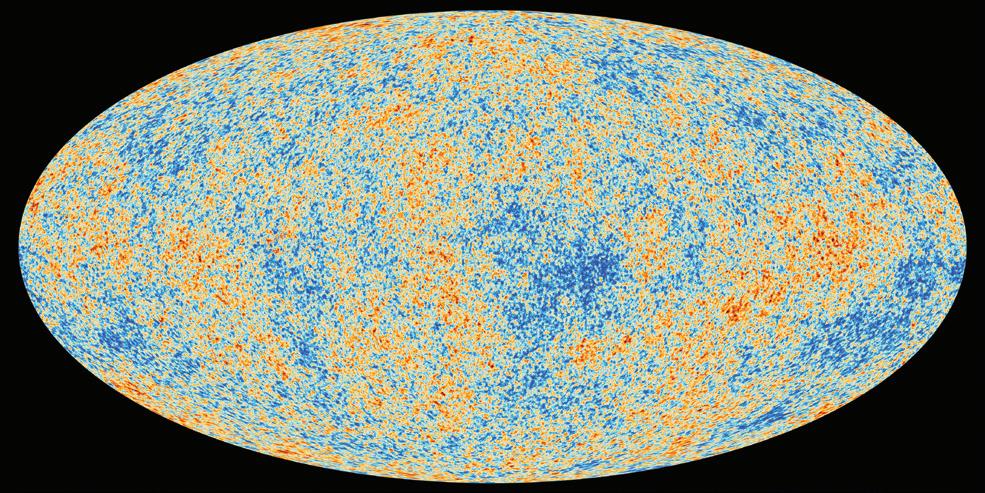
If we can’t see the centre or the edge of the Universe, then how can we possibly guess its lifespan?
So, first and foremost, there is no centre of the Universe. The Big Bang happened everywhere, all at once. Expansion is happening everywhere as well. Although it appears that, as we look out, we see everything moving away from us, that’s just our perspective. It’s nothing to do with the Universe itself. If you hopped to another galaxy, you’d see exactly the same thing. We are each at the centre of our own observable Universe because we can see the same distance in all directions around us. So, essentially, you are the centre of the Universe! We don’t need to see the edge of the Universe either (if there is one!) to work out how long it’s been around for or what’s going to happen in the future. The greater the distance you look in the Universe, the more expansion you see. So, with every extra million kilometres you step, galaxies appear to be moving away faster than those closer by, because there’s more space that’s expanding between us and them. Therefore, when we measure the expansion, we’re measuring how much the speed changes (in, say, kilometres per second) for every kilometre you go. Since we know how big the Universe is from side to side, we can work out how long it must have been expanding for at the rate that we measure to give us an estimate for the Universe’s age.
What would happen to your body in space without a working suit or suitable protection?
A lot of people think that the big issue in space is the temperature, that space is incredibly, incredibly cold. While it’s true that the average temperature of space is around 2.7 degrees above absolute zero (-273°C/459°F), if you went out into space with no suit, you would actually feel warm if in the sunshine.
The reason for this is that space is a vacuum. There are no air molecules and air molecules are essential for heat transfer. In air, molecules collide all the time and they gain and lose energy – that’s how heat is transferred away and to you in Earth’s atmosphere. But because space is a vacuum, that doesn’t happen. The only way for energy to be lost in space is by infrared radiation and it would take a very long time for heat to radiate away from your body and to freeze in space. Which is also why, if you went out into space with no suit at all, you might feel warm if you were in the sunshine because you’d be getting hit by infrared radiation from the Sun which transfers energy.
So, if it’s not the cold that will kill you, what will? Well, it’s that lack of air in the vacuum again. If you were being flung out from your craft’s airlock into space, your instinct might be to take a big deep breath, but as the pressure drops, the air in your lungs would compensate for that pressure drop by expanding to bursting point. Scuba divers will be familiar with this concept and it’s the reason why you should never hold your breath underwater while scuba diving in case you change depth (and therefore water pressure) very quickly. The whole ‘no air’ thing isn’t ideal but the main problem of being in a vacuum is that the boiling temperature of water is lowered. So, all of the water in your body would start to evaporate. You’ll get incredibly dry eyes and an incredibly dry mouth, but also the water in your bloodstream will start to boil. With nowhere to go, it will get trapped under your skin so that it looks like your skin is bubbling. Along with the water, the oxygen in your blood will also evaporate and your blood will become deoxygenated in about fifteen seconds. When deoxygenated blood hits your brain, you will pass out. So, thankfully, you wouldn’t experience any of these unpleasant sensations, but your fellow astronauts would only have a minute or so to save you.
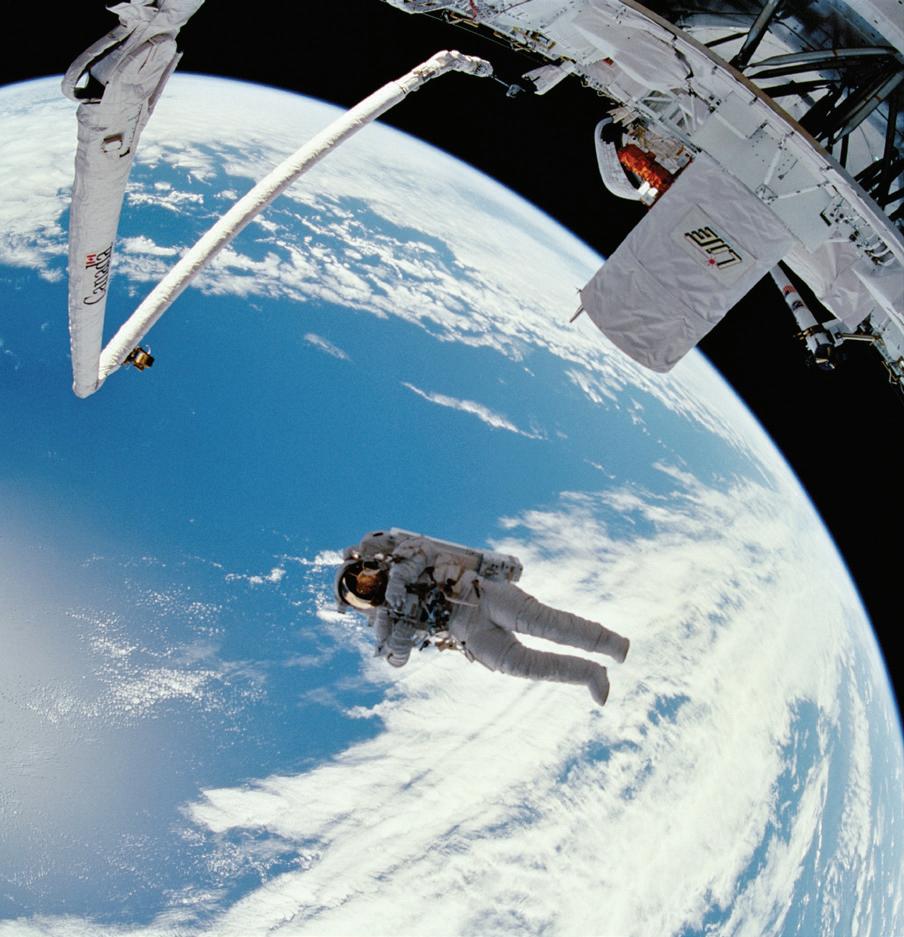
OPPOSITE: This map of the universe was produced by ESA’s Planck mission. It shows the oldest light in our universe, called the cosmic microwave background. This was imprinted on the sky when the universe was 370,000 years old. The colours represent tiny temperature fluctuations that correspond to regions of slightly different densities – the stars and galaxies of today. ESA and the Planck Collaboration LEFT: Astronaut Carl J. Meade performs an untethered spacewalk in 1994. NASA
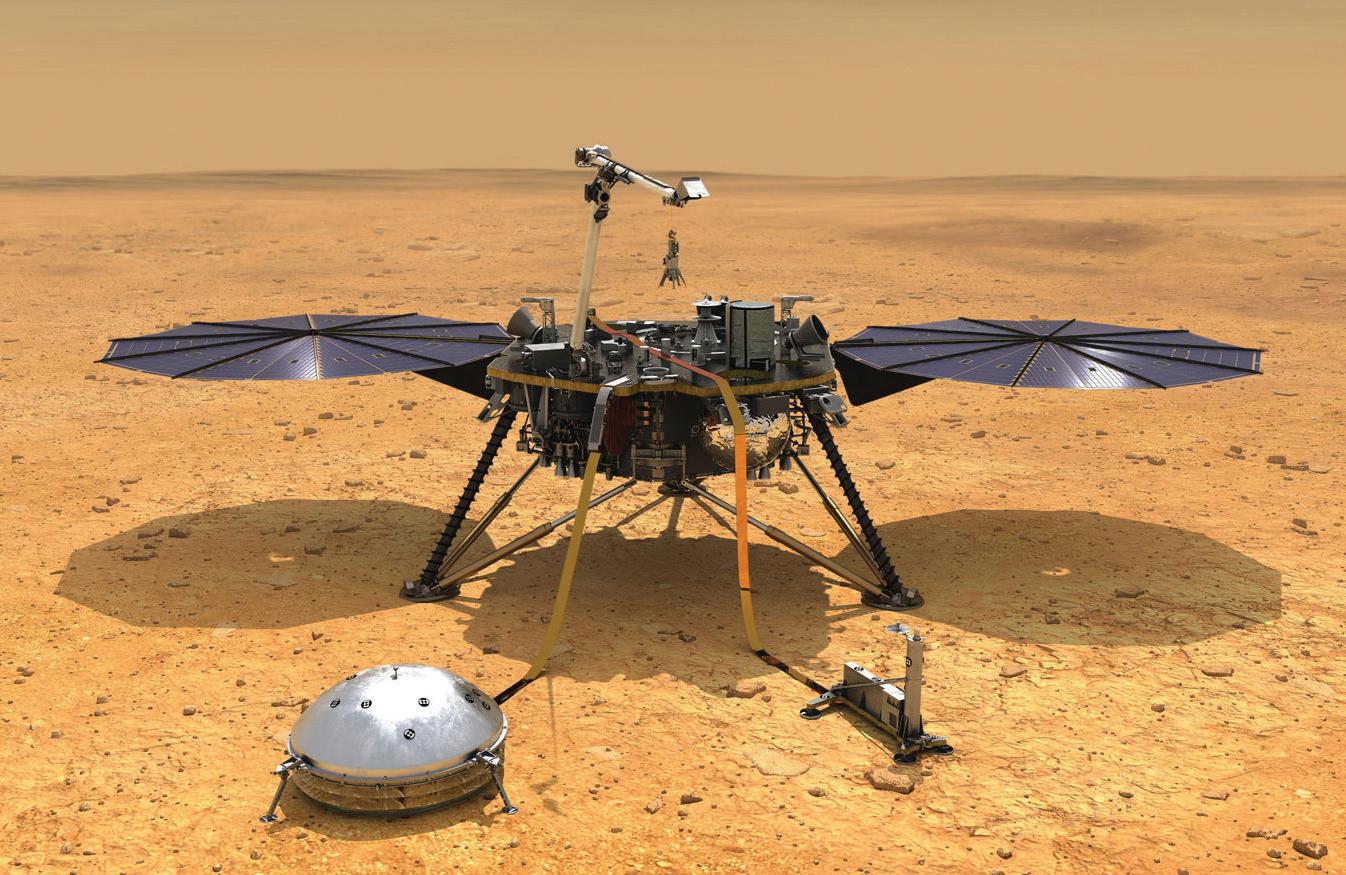
What is the most current model of the internal structure of Mars and how does that compare to Earth?
An illustration of the InSight lander on Mars, showing its instruments deployed on the Martian surface. NASA/JPL-Caltech
Thanks to NASA’s InSight lander, which touched down on Mars in November 2018, we now have a much better idea of the interior structure of the planet. InSight is fitted with a seismometer to detect marsquakes – equivalent to our own earthquakes. Geologists use earthquakes to determine the internal structure of our planet by detecting how they travelled through the various layers of solids and liquids under the crust. Starting at the surface and travelling inward, we know that the Earth has a rocky crust which, on average, is between 35 and 200km deep (22–124 miles), a liquid mantle of magma, surrounding a fluid outer core made mostly of iron and nickel. Finally, in the middle, Earth has a solid iron and nickel core which is around 20 per cent of the total radius of the planet. Mars has its similarities. The latest data from the InSight lander suggests its crust is anywhere from 20 to 40km (12–25 miles) thick, again with a liquid mantle below it, surrounding a liquid iron core. Mars’s core, however, makes up just over half of the entire planet, which is very different to our planet. Even marsquakes are generated differently to earthquakes. On Earth, tectonic plates move and slip past each other to create an earthquake. However, Mars is not as geologically active and so does not have tectonic plates. Instead, its rocky crust has been cooling and therefore shrinking since it formed billions of years ago. As it shrinks, if there are any faults in the rock, the pressure releases and a marsquake is generated. It’s intriguing to think about how the different interior structures of Earth and Mars led to their conditions today. The liquid metal in Earth’s core is key to having a protective shield, our magnetic field. It’s so hot that the electrons and protons in atoms are no longer bound together. As heat is transferred around by convection, these charged particles move in patterns that generate the Earth’s magnetic field which protects us from high-energy charged particles from the Sun. These get funnelled to the poles and interact with elements in the atmosphere to produce the aurora. We think that once upon a time Mars’s atmosphere was thick and lush, providing the perfect conditions for life. However, Mars doesn’t have a very strong magnetic field, suggesting its liquid core does not have the same movement of charged particles as Earth’s. Without a strong magnetic field, Mars has been bombarded with solar radiation that has slowly stripped the Red Planet of its atmosphere, leaving a planet that now appears to be a barren wasteland. It’s why the Perseverance rover is currently on the Martian surface hunting for fossilised life from a time when the conditions on Mars may have been more favourable. If we do ever find life on Mars, the big question will be whether it is at all similar to the life we see on Earth: does it have a common origin? Did comets and asteroids perhaps bring the building blocks for life as they collided with each of the planets? Or did those same impacts throw material off Earth into space which made its way to Mars to seed life, or maybe the other way round? By investigating Mars, we might just be able to answer one of the biggest questions humanity has ever asked: where did we all come from?
When we look at the oldest galaxies we can see, is there reason to believe they formed or behaved any differently from the newer ones?
Astronomy is like a time machine. If you look at things a long way away, then – because it takes photons of light from those objects so long to reach us – you are seeing them as they were a long time ago. And in the case of the most distant galaxies, that’s over 13 billion years ago! We are seeing what the ancestors of galaxies close to us today will have looked like. They haven’t yet settled into the kind of shapes that we see today. They don’t have either the characteristic spiral or elliptical shape that we associate with nearby galaxies and instead tend to be a lot smaller.
The big galaxies we see around us are ones that have merged and collided with neighbours to develop their shape. Early galaxies are different, but that’s because they have not yet evolved. There aren’t galaxies like this around in the same way today. This is also a way of proving the Big Bang theory is correct. It shows that the Universe was definitely very different in the past because the galaxies looked so different.