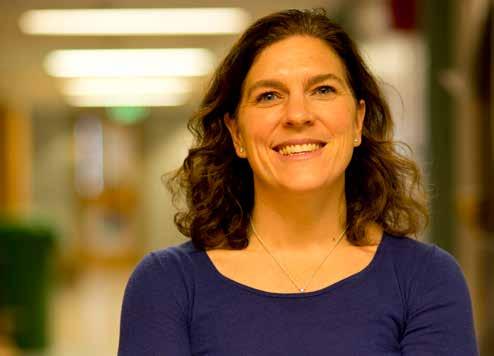
25 minute read
Research Spotlight On: Lisa Goodrich, Ph.D
Spotlight On: Lisa Goodrich, Ph.D.
Hearing Health Foundation’s Hearing Restoration Project (HRP) is the first international research consortium investigating how to regenerate inner ear sensory hair cells in humans to eventually restore hearing. Hair cells detect and turn sound waves into electrical impulses that are sent to the brain for decoding. Once hair cells are damaged or die, hearing is impaired, but in most species, such as birds and fish, hair cells spontaneously regrow and hearing is restored. Following a unique, overarching principle of cross-disciplinary collaboration, nearly instant data sharing, and using multiple animal models, the HRP is working to uncover how to replicate this regeneration process in humans. Lisa Goodrich, Ph.D., became the new scientific director of the HRP in January 2021, having since 2016 served as a member of HHF’s Scientific Advisory Board. A professor of neurobiology at Harvard Medical School whose lab focuses on how neural circuits develop and function, Goodrich received a B.A. in biological sciences from Harvard University and her doctorate in neuroscience from Stanford University. After completing postdoctoral training at the University of California, San Francisco (and Stanford, after the lab moved there), she joined the Harvard Medical School faculty in 2002. In mid-March 2021, Goodrich oversaw the annual HRP meeting, leading discussions of outcomes, plans, and structure.
Advertisement
Early Inspiration
When I was in high school, I was fortunate to spend two years with a special biology teacher who helped us to realize that science isn’t just about memorizing facts. She took us out to collect samples from a nearby lake, challenged our assumptions about how the world works, and encouraged us to design and do our own research in school. She even let me set up an aquarium full of breeding medaka in her classroom so I could study them for my project. I remember cutting lunch short so I could peek in and check on my fish. I was so excited when I saw the first eggs! Because of her, I entered college wanting to be a biology major and never looked back.
I have a sister with Down syndrome, so I have always been fascinated by how the brain develops. This was the focus of my graduate research. In my final year of graduate school I went to Seattle to visit my uncle, who introduced me to his friend Ed Rubel. I had lunch with Ed and Jenny Stone, who are past and current HRP members, and learned about the auditory system and hair cell regeneration. It made a big impression.
Several years later as a postdoc at UCSF, I reached out to Ed to learn how I might apply my knowledge of neural development to the auditory system. He invited me up to Seattle to learn some anatomy and also introduced me to folks at UCSF who taught me things like how to dissect the cochlea and record auditory brainstem responses. Around the same time, I got to know HRP member Andy Groves and Scientific Advisory Board member Doris Wu through our shared love for development. Having both trained in other fields as well, Andy and Doris echoed Ed’s encouragement and gave me the confidence to make the transition to the auditory system. All of these people have been wonderful sources of information, support, and advice ever since, and now as part of the HRP I feel incredibly fortunate to get to work with the people who played such an important role in bringing me into the field.
In the Lab
Our lab studies how neural circuits acquire their specialized, functional properties, largely in the auditory system. In particular, we are interested in the cellular and molecular mechanisms that create networks of neurons that reliably encode complex sound stimuli, from the initial detection in the cochlea to the first stage of processing in the auditory brainstem. For instance, we showed that there are multiple molecularly distinct subtypes of spiral ganglion neurons and that this diversification depends on signaling from the hair cell.
We are also fascinated by the stereotyped pattern of connections seen in auditory circuits—synapses are organized by neuron subtype along the base of the hair cell and are organized according to sound frequency along the dendrites of individual target neurons. Several projects are aimed at figuring out how these connections form and what happens to circuit function when they do not.
Although my lab does not study hair cell regeneration, my research benefits from the fantastic tools and resources that the HRP is producing. For example, the gEAR platform created through the HRP makes it possible for anyone to screen hundreds of published datasets, and not just those describing gene expression in the ear. This is one of the wonderful things about the HRP—the impact spreads throughout the field and beyond.
Collaboration
I am most excited by ideas, whether theoretical or practical. I love when I leave a seminar with a new method to try or when I read a paper that suddenly makes me think about our work in a different way. Insights can come from the most random places and without warning, so part of what motivates me every day is knowing that science creates opportunities to do things we never even imagined.
Working with my colleagues in the HRP consortium has exposed me to so many new ways of thinking about hair cell regeneration. I learn something every time we get together—the atmosphere is open, collegial, and inspiring. Rather than having to wait for the papers to be published or try to absorb everything in a 10-minute podium talk, we get to discuss the data as it is unfolding, when it is still possible to incorporate cool new ideas and take advantage of unexpected points of synergy. It is such a great way to do—and appreciate—science.
In the next few years, I hope we will have a molecular language for explaining the phenomenon of regeneration, both when it succeeds and when it fails. HRP consortium members, along with many others in the field, have been collecting and analyzing data from a wide range of systems where hair cells do or do not regenerate. We will learn a lot just by analyzing these data to find patterns within and across systems.
However, our collective experience and intuition as a field will play a huge role in helping us to make sense of what the computers tell us. This is why we want the data and resources to be readily accessible to anyone. It will take many groups working in parallel to find ways to convert supporting cells into hair cells, to hit the perfect balance of proliferation and differentiation, and to functionally integrate these new cells into the cochlea. The good news is we know it is possible because hair cells regenerate naturally in fish and chicks and even in newborn mice. By studying these systems, we can design a blueprint for what needs to happen and then use our rich understanding of the cells and molecules of the mammalian cochlea to make it happen.
Work and Play
These days a typical day for me is pretty simple. I wake up, feed my cats, and then sit in front of the computer typing or talking and often shooing away a cat. I wear many hats, so on any given day I might be recording lectures, grading assignments, talking to someone in my lab about their data, watching a seminar, working on a manuscript, or talking to the folks at Hearing Health Foundation.
I love to escape my brain by being active. Sometimes I squeeze in some yoga or a quick run, but the best days end on the tennis court, weather permitting. I’m not any good but that makes it even more fun. It’s like being a kid again, just delighting in occasionally hitting a ball just right. I am also a devoted reader of fiction, with a peculiar preference for very long books such as Donna Tartt’s “The Goldfinch.” It is very Dickensian, which I love. I think every academic should read “Stoner” by John Williams. It is a gem. And short!
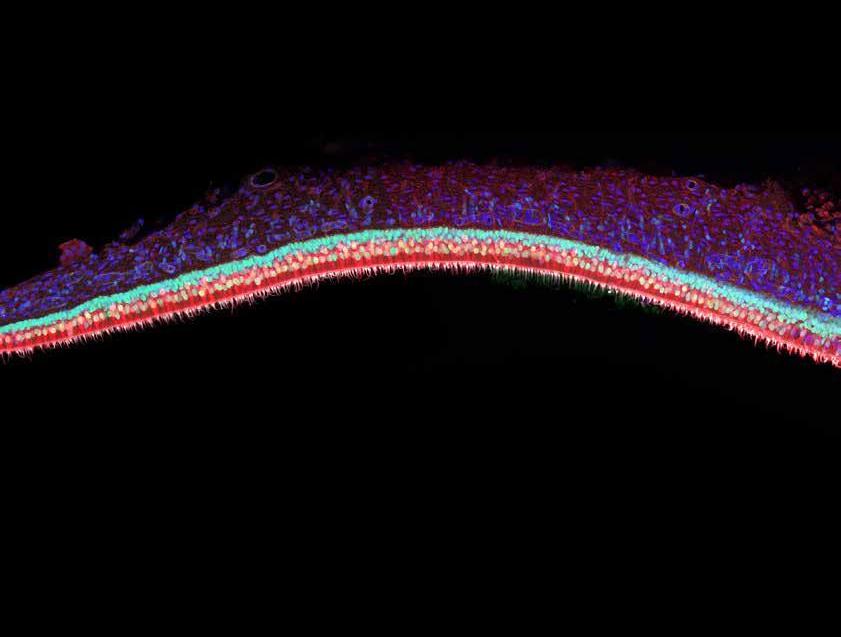
Hearing Health Foundation (HHF) improves the lives of people with hearing loss and related conditions by funding research, connecting researchers, publicizing new findings, raising awareness about hearing loss, and promoting hearing health.
Complex and interconnected, hearing loss and its variants impact speech processing, brain function, cognition, interpersonal relationships, psychological well-being, and quality of life. HHF’s advances in one aspect or disease-specific area of hearing often benefit many others, creating pathways to better prevention methods, treatments, and cures.
» Fundamental studies of inner ear hair cell function may shed light on these mechanisms: how hearing loss occurs, how balance is disturbed, how tinnitus is generated, how the brain processes sound and speech, and how cognitive decline accelerates. As a consequence, advancing knowledge in one area of research benefits many related areas and promotes novel therapies.
» Breakthroughs in understanding sensorineural hearing loss also enlighten studies on tinnitus, hyperacusis, and auditory processing disorders because the biological systems that are involved— the inner ear, the brainstem, and parts of the brain such as the auditory cortex—are shared. » Developing new means to deliver therapeutic drugs to the inner ear across the blood-labyrinth barrier may lead to discoveries about that barrier that help scientists prevent damage to hearing as a side effect of other drugs (ototoxicity) or of infection.
» Work on the role of neural circuits in the auditory processing of speech may improve our understanding of hyperacusis, as these same neural circuits play a role in the brain’s sensitivity to sound.
» Discovering how to regenerate inner ear hair cells to restore hearing may also help medical researchers and clinicians treat tinnitus, which is often caused by these hair cells “mis-signaling” the brain.
Recent Research by Hearing Health Foundation Scientists, Explained
A Combination of Genes to Enhance Hair Cell Regeneration in the Adult Mammalian Cochlea
Hearing loss is often caused by the loss of auditory hair cells, due to loud acoustic signals or medications that have side effects in the ear. The loss of hair cells reduces the sensitivity of the ear and makes sounds like speech blurry and harder to comprehend. It is also the case that ears with trauma are more susceptible to further lesions because the natural protective mechanisms are impaired. Once lost, hair cells in the mature mammalian cochlea are not naturally replaced, making them perhaps the only type of epithelial cell (epithelium is a layer of cells that lines cavities and covers surfaces in the body) that is not replaced/regenerated. Due to the lack of spontaneous regeneration, the hearing loss caused by hair cell loss is permanent. One way to envision future biological/medical repair of the hair cell-depleted inner ear is to medically induce the generation of new hair cells.
Hair cells are surrounded by other cells that are generally called supporting cells. Both originate from a common ancestor cell in the embryonic ear. One idea is to identify molecular pathways that can “reprogram” supporting cells in deaf ears to turn them into new hair cells. This process is called transdifferentiation and implies a change in the identity of one cell type into another, manifested in the shape, molecular composition, and function. Transdifferentiation is a relatively rare biological phenomenon. Among the few examples of naturally occurring transdifferentiation in mammals are the balance organs in the inner ear, where the sensory cells are also hair cells and the transdifferentiation of supporting cells can replenish lost hair cells. The therapeutic strategy guiding our efforts for inducing transdifferentiation in the cochlea is guided by combining knowledge of molecular pathways active during development and during natural transdifferentiation in the balance organs.
The huge task is to identify genes or small molecules that can trigger the reprogramming of supporting cells into hair cells. This requires the identification of genes that promote the development of hair cells versus supporting cells. Several labs have performed experiments to express or activate genes that induce generation of hair cells, in supporting cells. In mature living mammals, induced expression of genes can be accomplished by gene transfer using inactivated viruses or by using drugs that regulate the molecular pathways that control these genes.
Research on transdifferentiation has focused on a gene called Atoh1 that is necessary for hair cell formation during development. Earlier work in my lab and elsewhere has shown that the artificial activation of Atoh1 with viruses can induce the appearance of cells that exhibit hair cell features in mature mammalian ears. While the data were promising and exciting, the efficiency was typically too low and the variability too large, indicating that a better approach that yielded a more efficient and reliable regeneration of hair cells is needed.
Together with the Groves lab, we tested whether two hair cell genes, Atoh1 and Gfi1, in tandem could enhance regeneration efficiency. We generated viruses that included both genes and injected them into the inner ears of mice deafened by killing their hair cells. This allowed us to evaluate the combination of Atoh1 and Gfi1 in promoting regeneration in the adult deaf cochlea. Our December 2020 paper in Scientific Reports showed that the combination of Atoh1 and Gfi1 produces six times more new cells resembling hair cells than did Atoh1 alone. Although these new cells have many characteristics of hair cells, they do not appear like normal mature hair cells. We now face the task of identifying the means to enhance completion of their differentiation into mature functional hair cells. Combinatorial Atoh1 and Gfi1 treatment is thus a promising strategy to promote hair cell regeneration in the mature mammalian cochlea. —Yehoash Raphael, Ph.D.
Study coauthors and Emerging Research Grants alumni Andy Groves, Ph.D. (far left), and Yehoash Raphael, Ph.D., are members of HHF’s Hearing Restoration Project.
Brain Inflammation Is a Potential Target to Treat Tinnitus
More than 50 million Americans struggle with tinnitus, hearing a sound in the ears in the absence of an external source. A symptom rather than a disease itself, tinnitus in patients ranges from annoying to debilitating and has no cure. Some treatments help some people, but no one treatment seems to work for everyone. A major risk factor for tinnitus is hearing loss.
Shaowen Bao, Ph.D., an associate professor of physiology at the University of Arizona College of Medicine–Tucson, and colleagues are closing in on potential treatments for tinnitus by connecting brain inflammation to the condition. They found inflammation in a sound-processing region of the brain triggers evidence of tinnitus in mice that have noise-induced hearing loss, according to a study published in the journal PLOS Biology in June 2019. Coauthors include 2019 Emerging Research Grants scientist Hao Luo, M.D., Ph.D.
Recent studies indicate that noise-induced hearing loss causes inflammation—the immune system’s response to injury or infection—in the brain auditory pathway. How it contributes to hearing loss–related conditions, such as tinnitus, however, is not well understood. Bao and colleagues examined neuroinflammation (inflammation in the nervous system) in the auditory cortex of the brain following noise-induced hearing loss, and its role in tinnitus.
Their research showed mice with noise-induced hearing loss (under anesthesia) had elevated levels of molecules called proinflammatory cytokines and the activation of non-neuronal cells called microglia, two defining features of neuroinflammatory responses, in the primary auditory cortex in the brain.
The research also showed that the cytokine TNFalpha (tumor necrosis factor alpha), a cell signaling protein (cytokine) involved in systemic inflammation, is necessary for noise-induced neuroinflammation, tinnitus, and synaptic imbalance (an altered pattern of signaling between neurons). When the researchers used a pharmacological drug to block the TNF-alpha, the mice no longer showed signs of tinnitus.
Bao has been examining the role of TNF-alpha in tinnitus since 2011. “People have found clues for the cause of tinnitus but because many parallel components are involved, we would block one component, then we would have to block another, then another still,” Bao says.
“Neuroinflammation seems to be involved in many of these components. We hope blocking neuroinflammation will give us a better chance to block them all, thereby stopping the tinnitus.”
The findings suggest that neuroinflammation may be a therapeutic target for treating tinnitus and other hearing loss-related disorders. However, Bao says, “We have more work to do to confirm the mechanism that is causally linked to tinnitus and determine if the results translate to humans. While promising, we still have a long way to go from research to patient care.”
This originally appeared as a University of Arizona press release. A research associate at Wayne State University in Detroit, Hao Luo, M.D., Ph.D., is a 2019 Emerging Research Grants scientist generously funded by the General Grand Chapter Royal Arch Masons International.
Mass Spectrometry Imaging Reveals Blast Effect on Neurotransmitters in the Chinchilla Model
An imbalance in the excitation and inhibition of neurotransmitters is implicated in the pathomechanism (causes) of tinnitus, but more research has been warranted. To investigate, many studies have examined physiological changes due to an acute or chronic exposure to high levels of sound or using salicylate in an animal model. Many other studies target concentration levels of specific molecules using indirect, noninvasive methods in humans and using both invasive and noninvasive methods in animals. These methods have identified the neurochemistry underlying physiological abnormalities induced by tinnitus, and have begun to probe the many molecular mechanisms that occur along the auditory pathway.
This is where the use of mass spectrometry imaging (MSI) has been a powerful new approach for tinnitus research. MSI combines the highly specific in vivo detection of neurotransmitters with the visualization made possible by microscopy—allowing for the direct mapping of molecules throughout sections of tissue. This MSI method of global analysis is a promising platform to bridge the gap in many clinical and translational studies.
Also of note is that the direct link from an animal model of noise-induced tinnitus to the human pathophysiological response has not been perfect, with many mouse, rat, and guinea pig models having been investigated. The chinchilla model, however, has similarities to human hearing frequencies and how it transmits (conducts) sound from the ear to the brain.
For our study in Experimental and Molecular Biology in April 2021, we produced relative quantitative imaging of the excitatory neurotransmitter glutamate and the inhibitory neurotransmitter GABA in the chinchilla central auditory neuraxis, which includes the auditory cortex, cochlear nuclei, and inferior colliculus, the primary centers of the auditory pathway.
The ultra-high resolution, quantitative imaging was performed using Fourier transform ion cyclotron resonance mass spectrometry. Our research provides insights about the homeostasis (state of equilibrium) of GABA/glutamate within whole-brain sections of the chinchilla in order to investigate the pathomechanism of blast-induced tinnitus.
The data obtained was formed into “intensity map” images showing how much and where the molecule was detected in sections of the brain containing the auditory cortex, cochlear nuclei, and inferior colliculus. After comparing the blast overpressure–exposed subject to a control, we observed that exposure to acute levels of noise disrupted GABA molecule levels. Three days postexposure, we saw a unilateral variance of the inhibitory GABA in the auditory cortex and a global depletion of GABA in the inferior colliculus, while levels of the excitatory glutamate stayed relatively constant.
Our results showing the depletion of GABA in the auditory cortex and inferior colliculus in the blast overpressure–exposed chinchilla model is in agreement with evidence of a GABA reduction observed utilizing electrophysiology and noninvasive measures in humans and rats. By adding this directly measured data, we strengthen the hypothesis that neurotransmitter disruption is linked to the persistence of tinnitus. Future studies using these methodologies in the chinchilla model will include more subjects at multiple time points and exposure levels, as well as profile additional molecular changes to help uncover the pathomechanism of tinnitus. —Vijaya Prakash Krishnan Muthaiah, Ph.D.
Vijaya Prakash Krishnan Muthaiah, Ph.D., is an assistant professor in the department of rehabilitation sciences at the University at Buffalo, the State University of New York. He is a 2019 ERG scientist who was generously funded by the General Grand Chapter Royal Arch Masons International.
Evaluation of a New Method for Measuring Pediatric Hearing
Little is known about auditory development during the toddler and preschool years. The primary reason for this gap in knowledge is due to difficulty obtaining reliable behavioral data from young children. Recent work in my laboratory has focused on developing a method for testing this population called the Play Observer–Based, Two Interval (PlayO2I) Method.
In our method, a child is trained to perform a playbased task when a signal is heard (e.g., put a block into a bucket). An experimenter—called an observer—watches the child’s behavior to determine when the signal was played. In contrast to clinical testing methods, the signal is randomly placed in one of two intervals for each trial by the computer program. Based on the child’s behavior, the observer determines the interval with the signal. The benefit of this approach is it is time efficient and can guard against some forms of bias. Additionally, because the child is unaware that there are two intervals, it minimizes the cognitive demand for the task.
Our December 2020 paper in the American Journal of Audiology examined the interrater reliability of this method for a group of 2- to 4-year-old children who are developing typically. Children were asked to detect a puretone signal in one of two conditions: fixed-level testing, where the signal is presented at the same clearly audible intensity throughout testing; or variable-level testing, where the signal is presented at different levels that are near the child’s anticipated threshold.
All test sessions were video recorded and were then coded by three observers (one real-time, two offline). Comparing data from the three observers from each test session, we answered two research questions: How often do the observers agree on which interval the signal occurred in? Is the resulting summary data for the test session—total percent correct score or threshold—similar for the three observers?
Results from this study confirmed strong interrater reliability for the PlayO2I Method. Reliability estimates were affected by the signal intensity level and the type of behavior provided by the child. Resulting summary data was similar across the three observers for both test conditions evaluated. These results indicate that the PlayO2I method is a powerful tool for researchers who are evaluating hearing abilities in young children. —Angela Yarnell Bonino, Ph.D., CCC-A
Angela Yarnell Bonino, Ph.D., CCC-A, is an assistant professor in the department of speech, language, and hearing sciences at the University of Colorado Boulder. She is a 2017 ERG scientist who was generously funded by the General Grand Chapter Royal Arch Masons International.
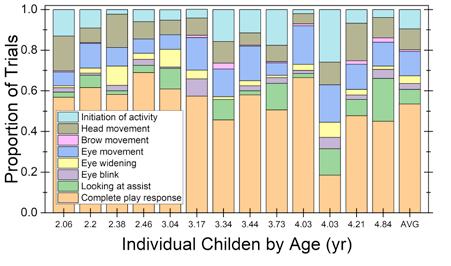
Based on data from the offline observers, Bonino and team computed the proportion of trials in which each of the eight auditory behaviors were coded for the variable-level condition. The pattern of behavior is shown for individual children, rank ordered by age. For reference, the average pattern of performance is provided in the last column. Trials in which no behavior was observed were excluded.
Deciphering a Mechanism Behind Bone Conduction Hyperacusis
Bone conduction hearing is commonly used for practical purposes: Standard hearing tests measure bone conduction thresholds to assess sensorineural hearing; bone conduction hearing aids help patients with conductive and mixed hearing loss; and bone conduction earphones of various types are increasingly popular. However, the underlying mechanism of bone conduction sound transmission to the inner ear has been elusive and poorly understood because bone conduction sound transmission is complex—multiple frequency-dependent mechanisms may be involved.
An understanding of bone conduction has been further hindered due to the inability to measure bone conduction sound transmission directly in the inner ear. Also unclear is how some abnormalities can affect bone conduction hearing. One example is bone conduction hyperacusis (hypersensitive bone conduction hearing)—an unnerving symptom experienced by patients with superior canal dehiscence (SCD), an atypical thinness or incomplete closure of one of the bony canals in the inner ear.
However, recent developments now allow the experimental measurement of intracochlear sound pressures during bone conduction in fresh human cadaveric specimens, which can provide detailed information regarding inner-ear sound transmission and estimate hearing function.
For our October 2020 study in Scientific Reports, we measured bone conduction–evoked sound pressures in two of the fluid-filled canals of the cochlea, the scala vestibuli and scala tympani. We estimated hearing at the base of the cochlea using the cochlear input pressure drive (the differential pressure across the cochlear partition that separates the canals), before and after creating an SCD.
Consistent with clinical audiograms, an SCD increased the bone conduction–driven cochlear input drive below 1 kHz. However, an SCD affected the individual canal pressures in unexpected ways: The SCD increased the scala vestibuli by 5 to 20 decibels significantly between 250 and 550 hertz, but had little effect on the scala tympani.
These findings are inconsistent with the inner-ear compression mechanism that some have used to explain bone conduction hyperacusis. We developed a new computational bone conduction model based on the innerear fluid-inertia mechanism, and the simulated effects of the SCD were similar to the experimental findings. This experimental-modeling study suggests that inner-ear fluid inertia is an important mechanism for bone conduction hearing. It also suggests that the SCD facilitates the flow of sound volume velocity through the cochlear partition at low frequencies, resulting in bone conduction hyperacusis.
The intracochlear pressure measurements verified with the modeling analysis provide a new understanding of bone conduction sound transmission and the mechanism underlying SCD-induced bone conduction hyperacusis. —Xiying Guan, Ph.D.
A 2016 ERG scientist funded by Hyperacusis Research Ltd., Xiying Guan, Ph.D., is an instructor in the department of otolaryngology–head and neck surgery, Harvard Medical School, and an investigator at the Eaton-Peabody Laboratories, Massachusetts Eye and Ear.
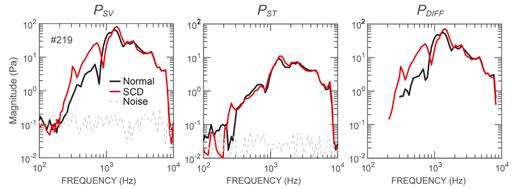
Magnitude frequency responses of the scala vestibuli (PSV ), scala tympani (PST ), and cochlear input pressure drive (PDIFF ) during bone conduction stimulation in a representative ear. Black lines are the pressures under typical conditions; red lines are the pressures after a superior canal dehiscence (a hole in one of the inner ear’s bony canals) was produced. This generally increased PSV below 1 kHz. Interestingly, the effects on PST were generally smaller.
Hearing Difficulties in Noise Traced to Altered Brain Dynamics Following Cochlear Neural Degeneration
When the axons (nerve fibers) of neurons (nerve cells) are damaged or dysfunctional, the sensory information that is relayed from sensory organs, such as the ear, to the brain is reduced and limited—like dead pixels in a computer display. In the auditory nerve, it is type I spiral ganglion neurons (SGNs) that transmit virtually all signals from sensory hair cells in the cochlea to neurons in the auditory brainstem. These type I SGNs are the most vulnerable element in the inner ear, sensitive to environmental effects and aging.
Seminal work by M. Charles Liberman, Ph.D., and Sharon Kujawa, Ph.D., a decade ago revealed that acoustic overexposure can damage afferent cochlear neurons even when cochlear hair cells remain intact. Since then, work in over a dozen animal species has demonstrated a marked reduction of type I SGN functionality following exposure to ototoxic drugs or environmental noise. In humans, postmortem analysis of the inner ear shows that approximately 40 percent of SGN axons have already degenerated by age 50, well in advance of comparable losses in other inner ear cell types.
Even a small fraction of functional pixels would be sufficient to determine whether a computer was on or off. The difficulty would come in the recognition of a complex image. Eliminating as much as 95 percent of type I SGNs in mice has minimal effect on their ability to hear in quiet backgrounds. The challenge is hearing in noisy environments. In humans, evidence suggests that difficulty hearing in noisy, social settings—a symptom of hidden hearing loss—may reflect premature auditory nerve degeneration.
In our study in the journal Neuron in March 2021, Daniel Polley, Ph.D., and I report finding a deterioration in perception in noisy environments after inducing bilateral moderate auditory nerve degeneration in adult mice. We found that the resulting lesions produced surges of noisy, synchronized activity in the auditory cortex that preceded the mice’s failures to detect target sounds in noise.
Specifically, cochlear neuropathy produced distinct forms of cortical (brain) plasticity in both excitatory and inhibitory neurons that culminated in net hyperactivity, increased neural gain, and reduced adaptation to background noise. This pattern was not present in the typical-hearing mice, whose cortical responses maintained a typical balance of excitation and inhibition. In the typical-hearing mice, the lack of a target-evoked response predicted behavioral misses; in contrast, in mice after cochlear neuropathy, random surges of cortical activity before the tone was presented reliably predicted impending failures to detect sound— revealing a source of internal cortical noise underlying difficulties in the perception of external noise.
One encouraging finding is that the negative effects of auditory nerve damage may be overcome by promoting brain plasticity. Intensively training mice as well as humans with or without hearing impairment on sound-innoise discrimination tasks improved their ability to focus on a target sound (one speaker) amid competing sounds (multiple speakers). This raises the exciting possibility that the maladaptive cortical plasticity and perceptual impairments that our study details may be at least partially mitigated by auditory training. —Jennifer Resnik, Ph.D.
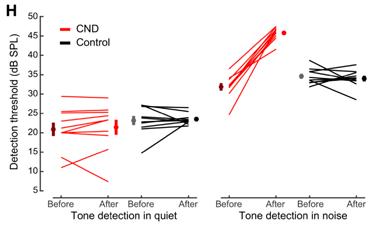
Cochlear neural degeneration (CND) in adult mice disrupts behavioral detection of tones in noise but not in silence.
A 2017 ERG scientist funded by Hyperacusis Research Ltd., Jennifer Resnik, Ph.D., is a senior lecturer at Ben-Gurion University of the Negev in Israel. 2020 ERG scientist Ross Williamson, Ph.D., supported this study with data collection software, and 1999 ERG scientist Sharon Kujawa, Ph.D., is a member of HHF’s Council of Scientific Trustees.