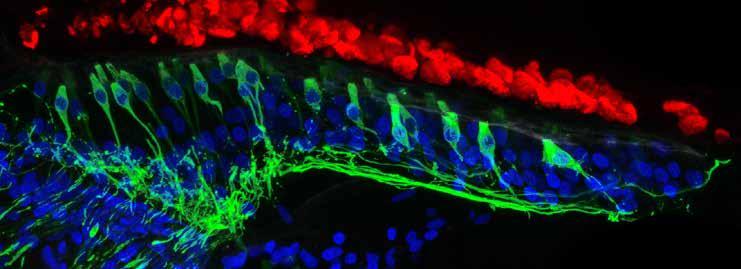
28 minute read
Research New Working Groups Reaffirm Team Science
New Working Groups Reaffirm Team Science Approach By Lisa Goodrich, Ph.D.
This year’s annual meeting of the Hearing Restoration Project (HRP) was held over two days in mid-March 2021 via Zoom, like most scientific (and other) events over the past year and a half, and was also the first annual meeting I oversaw as the HRP’s new scientific director. This presented an opportunity to assess and reimagine the group’s structures, processes, and ethos, even as the consortium’s end goal—to discover mechanisms to regenerate inner ear hair cells in humans to cure hearing loss and related disorders—remains the same.
Advertisement
A few years ago, the consortium decided to shift from proposals with multiple principal investigators (PI) to single-PI projects. The logic was straightforward: The outcomes of a few focused projects would help the HRP to refine its targets, and there is always a finite pool of funding available in any given year. The consortium and the Scientific Advisory Board (on which I sat before becoming HRP scientific director) must make difficult decisions about which experiments and projects are most urgent and promising.
At the same time, the HRP is founded on a firm belief in the advantages of team science and a conviction that collaboration and exchange produce the best results. In surveying the group’s recent accomplishments, we agreed that we needed a new approach to mine our rich datasets and begin to directly test ways to regenerate hair cells in vivo. We also reiterated our commitment to facilitate hair cell regeneration research more broadly by making the data available to anyone in the field, as enabled by the gEAR platform, whose creation the HRP funded.
We decided to form three working groups that will each submit one joint proposal per grant cycle. These working groups span the breadth of the HRP’s work as guided by its strategic plan: » The Cross-Species Epigenetic Analysis working group is producing epigenetic data sets for supporting cells in order to pinpoint the differences between successful regeneration in fish and chicks, and failure to regenerate (so far) in mammals; » The Integrative Analysis working group promotes data sharing, conducts cross-species analysis of data, and aims to more efficiently prepare data for publication; » The Reprogramming and Gene Delivery working group will test transcription factors to regenerate hair cells and develop new tools and methods to deliver these transcription factors to the inner ear.
Every HRP member has a role to play, and our new comfort with Zoom meetings will make it that much easier to bring HRP investigators and members of their labs together on a regular basis.
This restructuring of the HRP’s team science approach will reinvigorate the collaborative spirit and propel our work forward in new, creative ways. This renewed impetus should result more concretely in accelerated publication plans and, over a slightly longer term, additional major funding awards to members’ labs from the National Institute on Deafness and Other Communication Disorders to continue initial HRP research. I look forward to sharing details on the working groups’ projects and results as we embark on this exciting next phase of the HRP.
HRP scientific director Lisa Goodrich, Ph.D., is a professor of neurobiology at Harvard Medical School. For more, see hhf.org/hrp.
Recent Research by Hearing Health Foundation Scientists, Explained
Several Novel Findings Describing Cochlear Hair Cell Regeneration in Birds
Chickens are an important animal model for hearing restoration research because they are naturally capable of regenerating cochlear hair cells. This fact has been known for many years, but the mechanisms that initiate and control this regenerative ability are unknown. The funding provided by Hearing Health Foundation through the Hearing Restoration Project (HRP) has helped the development of a new research program in the Stanford University laboratory of Stefan Heller, Ph.D., focusing on chicken hair cell regeneration. Several years after its inception, this research is now bearing fruit.
In two high-profile publications in the journal Cell Reports in March 2021, a research team led by postdoctoral fellows Amanda Janesick, Ph.D., and Nesrine Benkafadar, Ph.D., reported several novel findings that lay the foundation for in-depth characterization of the cellular signaling that triggers and controls cochlear hair cell regeneration in birds. The work uses a recently developed technology called single-cell RNA-sequencing. This technology provides insight into changes in the activity of all genes as cochlear cells respond to hair cell damage and death.
To properly leverage single-cell RNA-sequencing technology, Janesick developed a new strategy to injure the chicken cochlea. The goal was to damage all hair cells “at once,” which is usually impossible with sound exposure or systemic injection of an ototoxic drug. However, using a surgical approach to the chicken cochlea, it is possible to inject a tiny amount of the drug sisomicin into the inner ear, causing all auditory hair cells to disappear within 24 hours.
Benkafadar took a closer look at the hair cells using single-cell RNAsequencing. She found that cochlear hair cells, when damaged and stressed by exposure to sisomicin, activate a particular set of genes related to cellular
Five days after ototoxic damage, the chicken regenerates sensory hair cells and neurons (green). Dead hair cell “ghosts” (red) float above the regenerating cells. Nuclei are marked in blue.
Support our research: hhf.org/donate.
A new hair cell type was discovered in the chicken inner ear. This finding will spur research toward uncovering the physiological function of this novel hair cell type. The work identified two distinct groups of supporting cells, which are the stem cells that give rise to new hair cells after damage.
stress. Her data show that hair cells “fight” to stay alive by invoking cellular repair mechanisms.
Benkafadar further discovered a mechanism that gets activated to cause hair cells to destroy their genetic material and subsequently disintegrate. This process, called programmed cell death, is well known and is generally a safety mechanism to prevent stressed cells from harming the body.
She found that in the chicken cochlea, hair cells “hang in there” for at least 16 hours before they dissolve and fall apart. During these 16 hours, the cells communicate with the remaining cells in the cochlea, the supporting cells. The researchers hypothesize that such signals ultimately instruct the supporting cells to commence the hair cell regeneration process. Ongoing work focuses on identifying the signals.
In total, this research created a comprehensive inventory of more than 15,000 individual activated and deactivated genes throughout the first 24 hours of hair cell demise. This rich source of important data is publicly available to the research community and will become the basis for future work that is now directly aimed at finding the relevant genes that initiate and control hair cell regeneration in birds.
In parallel, the HRP consortium is already working on comparing the identified genes with their mammalian equivalents, which will accelerate the translation of the findings toward future treatments.
A second aspect of the work that came from Janesick’s comprehensive inventory of the different cell groups of the chicken cochlea was the identification of many new marker genes for the different cochlear cell types. Two surprises resulted: 1) A new hair cell type was discovered in the chicken inner ear. This finding will spur research toward uncovering the physiological function of this novel hair cell type. 2) The work identified two distinct groups of supporting cells, which are the stem cells that give rise to new hair cells after damage. Interestingly, the two groups are spatially distinct, corresponding to two different places in the cochlea that utilize different mechanisms for hair cell regeneration. One mechanism is based on cell division, which is the desired strategy that the Heller lab focuses on for developing future therapeutics to regenerate hair cells in the mammalian cochlea.
“The HRP provided the funding to get this study started in an environment where it was impossible to obtain support for this work from the National Institutes of Health,” Heller says. “I hope that our data and the published results from the HRP consortium work will convince the NIH to support the necessary follow-up studies that will hopefully lead to new treatments for patients.” —Amanda Janesick, Ph.D., and Nesrine Benkafadar, Ph.D.
Amanda Janesick, Ph.D. (far left), and Nesrine Benkafadar, Ph.D., are postdoctoral fellows in the laboratory of Stefan Heller, Ph.D., a member of HHF’s Hearing Restoration Project and a professor of otolaryngology–head & neck surgery at Stanford University in California.
Elusive Cell Type in Fish Sensory Organs Discovered
One of the evolutionary disadvantages for mammals, relative to other vertebrates like fish and chickens, is the inability to regenerate sensory hair cells. The inner hair cells in our ears are responsible for transforming sound vibrations and gravitational forces into electrical signals, which we need to detect sound and maintain balance and spatial orientation.
Certain factors, such as exposure to noise or antibiotics, cause inner ear hair cells to die, which leads to hearing loss and vestibular defects, a condition reported by 15 percent of the U.S. adult population. In addition, the ion composition of the fluid surrounding the hair cells needs to be tightly controlled, otherwise hair cell function is compromised, as observed in Ménière’s disease.
While prosthetics like cochlear implants can restore some level of hearing, it may be possible to develop medical therapies to restore hearing through the regeneration of hair cells. Investigator Tatjana Piotrowski, Ph.D., at the Stowers Institute for Medical Research in Missouri, is part of the Hearing Restoration Project of Hearing Health Foundation, a consortium of laboratories doing foundational and translational science using fish, chicken, mouse, and cell culture systems.
“To gain a detailed understanding of the molecular mechanisms and genes that enable fish to regenerate hair cells, we need to understand which cells give rise to regenerating hair cells, and related to that question, how many cell types exist in the sensory organs,” Piotrowski says.
The Piotrowski Lab studies the regeneration of sensory hair cells in the zebrafish lateral line. Located superficially on the fish’s skin, these cells are easy to visualize and to access for experimentation. The sensory organs of the lateral line, known as neuromasts, contain support cells which can readily differentiate into new hair cells. Using techniques to label cells of the same embryonic origin in a particular color, other research had shown that cells within the neuromasts derive from ectodermal thickenings called placodes.
It turns out that while most cells of the zebrafish neuromast do originate from placodes, this isn’t true for all of them. In a paper published online in Developmental Cell in April 2021, Piotrowski and team describe their discovery of the occasional occurrence of a pair of cells within post-embryonic and adult neuromasts that are not labeled by lateral line markers.
When using a technique called Zebrabow to track embryonic cells through development, these cells are labeled a different color than the rest of the neuromast. “I initially thought it was an artifact of the research method,” says Julia Peloggia, a predoctoral researcher at The Graduate School of the Stowers Institute for Medical Research, co-first author of this work along with another predoctoral researcher, Daniela Münch, who says it is common in transgenic animal lines that the labels don’t mark all of the cells, especially when looking just at the nuclei of cells.
The pair agreed that it was difficult to discern a pattern at first. “Although these cells have a stereotypical location in the neuromast, they’re not always there. Some neuromasts have them, some don’t, and that threw us off,” Peloggia says. A normal invasive (that is, non-metastatic) behavior of cells after embryonic development is not often observed. Future research by the team will focus on identifying triggers for such behavior and the function of these newly differentiated, migratory, and invasive ionocytes, including how this process may relate to hair cell regeneration.
Support our research: hhf.org/donate.
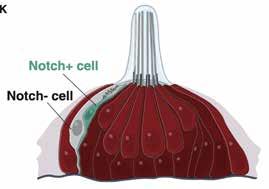
A newly discovered cell type in lateral line neuromasts, Nm ionocytes, invade mature sensory organs in a salinity- and pH-dependent manner, a process named adaptive cell invasion. This process allows for physiological adaptation of sensory organs to fluctuating environmental conditions.
By applying an experimental method called single-cell RNA sequencing to cells isolated by fluorescence-activated cell sorting, the researchers identified these cells as ionocytes—a specialized type of cell that can regulate the ionic composition of nearby fluid. Using lineage tracing, they determined that the ionocytes derived from skin cells surrounding the neuromast. They named these cells neuromast-associated ionocytes.
Next, they sought to capture the phenomenon using time-lapse and highresolution live imaging of young larvae. “In the beginning, we didn’t have a way to trigger invasion by these cells. We were imaging whenever the microscope was available, taking as many time-lapses as possible—over days or weekends— and hoping that we would see the cells invading the neuromasts just by chance,” Münch says.
Ultimately, the researchers observed that the ionocyte progenitor cells migrated into neuromasts as pairs of cells, rearranging between other support cells and hair cells while remaining associated as a pair. They found that this phenomenon occurred all throughout early larval, later larval, and well into the adult stages in zebrafish. The frequency of neuromast-associated ionocytes correlated with developmental stages, including transfers when larvae were moved from ion-rich embryo medium to ion-poor water.
From each pair, they determined that only one cell was labeled by a Notch pathway reporter tagged with fluorescent red or green protein. To visualize the morphology of both cells, they used special electron microscopy to generate high-resolution, three-dimensional images.
They found that both cells had extensions reaching the apical or top surface of the neuromast, and both often contained thin projections. The Notchnegative cell displayed unique “toothbrush-like” microvilli projecting into the neuromast lumen or interior, reminiscent of that seen in gill and skin ionocytes.
“Once we were able to see the morphology of these cells—how they were really protrusive and interacting with other cells—we realized they might have a complex function in the neuromast,” Münch says.
Peloggia adds, “Our studies are the first to show that ionocytes invade sensory organs even in adult animals and that they only do so in response to changes in the environment that the animal lives in. These cells therefore likely play an important role allowing the animal to adapt to changing environmental conditions.”
Ionocytes are known to exist in other organ systems. “The inner ear of mammals also contains cells that regulate the ion composition of the fluid that surrounds the hair cells, and dysregulation of this equilibrium leads to hearing and vestibular defects,” Piotrowski says.
And while ionocyte-like cells exist in other systems, it’s not known whether they exhibit such adaptive and invasive behavior.
“We don’t know if ear ionocytes share the same transcriptome, or collection of gene messages, but they have similar morphology to an extent and may possibly have a similar function, so we think they might be analogous cells,” Münch says. Peloggia adds that their discovery of neuromast ionocytes will let them test this hypothesis, as well as test how ionocytes modulate hair cell function at the molecular level.
Next, the team will focus on two related questions—what causes these ionocytes to migrate and invade the neuromast, and what is their specific function? “Even though we made this astounding observation that ionocytes are highly motile, we still don’t know how the invasion is triggered,” Peloggia says. “Identifying the signals that attract ionocytes and allow them to squeeze into the sensory organs might also teach us how cancer cells invade organs during disease.”
A normal invasive (that is, non-metastatic) behavior of cells after embryonic development is not often observed. Future research by the team will focus on identifying triggers for such behavior and the function of these newly differentiated, migratory, and invasive ionocytes, including how this process may relate to hair cell regeneration.
Peloggia plans to investigate what triggers the cells to differentiate, migrate, and invade, and Münch will focus on characterizing their function. “The adaptive part is really interesting,” Münch says. “That there is a process involving ionocytes extending into adult stages that could modulate and change the function of an organ—that’s exciting.”
This is adapted from a press release from the Stowers Institute for Medical Research, at stowers.org/news. HRP member Tatjana Piotrowski, Ph.D., is an investigator at the Stowers Institute for Medical Research in Missouri, where study coauthors Julia Peloggia and Daniela Münch are predoctoral researchers in the graduate school.
Given their adaptive and invasive behavior, these cells likely play an important role allowing the animal to adapt to changing environmental conditions.
Research Calls Attention to Dangerous Noise Levels in Gyms
Fitness center instructors often turn up music volumes significantly during classes—sometimes loud enough to cause hearing damage—based on an assumption that participants will work out more intensely when volumes are raised. A new University of Maryland School of Medicine (UMSOM) study, however, found that those who attend indoor cycling (spinning) classes do not lower the intensity of their workouts when the volume is reduced to a safer decibel level. The findings were published in the January–March 2021 issue of the journal Noise & Health.
“Our findings make a strong case for reducing music volumes in fitness classes to protect against hearing loss without sacrificing the intensity of the workout,” says study corresponding author Ronna Hertzano, M.D., Ph.D., an associate professor of otorhinolaryngology–head & neck surgery at UMSOM. “In fact, our study participants reported that they preferred the reduced sound level during their workouts.” An avid gym-goer, Hertzano is a member of HHF’s Hearing Restoration Project.
Support our research: hhf.org/donate.
Previous research suggests that the average sound levels in group fitness classes frequently exceed 90 decibels, as loud as an approaching subway train, and often exceed 100 dB, as loud as a power lawnmower. The National Institute of Occupational Safety and Health recommends that a noise exposure of one hour not exceed 94 dB, and exposures to levels of 100 dB should not exceed 15 minutes to protect against permanent hearing loss.
For the new study, the researchers selected a gym in the local Baltimore area and conducted surveys on participants, age 31 on average, who signed up for one-hour spinning classes. Music volumes in the spinning classes during the study ranged from 93 to 101 dB. In the classes that were 2 to 3 dB lower than the loudest classes—which translates to about a 50 percent reduction in power and a 20 percent reduction in perceived loudness—participants reported no differences in their exercise intensity. Those in classes with the lowest volume experienced a slight reduction in exercise intensity, but only two of these participants reported below-average exercise intensity.
Overall, more than one in four study participants reported experiencing auditory symptoms following their spinning class, including ringing in their ears or muffled hearing. For those in classes with the highest sound levels, nearly one-third of participants reported that the sound level was too high, and nearly one-third reported that they would prefer a decrease of the music level. Only three of the participants reported using hearing protection, such as earplugs, during the study.
“We also found that participants were most likely to report that the music level was satisfactory in classes where sound levels were lowest,” Hertzano says. “Importantly, the gym elected to maintain the music at the softer level after we made them aware of our study results.”
Lawrance Lee, a fourth-year medical student at UMSOM; Benjamin Shuster, a research fellow in the department of otorhinolaryngology–head & neck surgery at UMSOM; and Yang Song, Ph.D., a postdoctoral fellow in the Institute for Genome Sciences at UMSOM, all served as lead coauthors on the paper. Study researchers also included Sharon Kujawa, Ph.D., of Massachusetts Eye and Ear in Boston and a member of HHF’s Council of Scientific Trustees. —Deborah Kotz
This is adapted from a press release from the University of Maryland School of Medicine, at medschool.umaryland.edu/news. Hearing Restoration Project member and a 2009–10 Emerging Research Grants scientist Ronna Hertzano, M.D., Ph.D. (far left), is an associate professor of otorhinolaryngology–head and neck surgery at the University of Maryland School of Medicine. Council of Scientific Trustees member and 1999 ERG scientist Sharon Kujawa, Ph.D., is an associate professor of otolaryngology–head and neck surgery at Harvard Medical School and the director of audiology research at Mass Eye and Ear.
For references, see hhf.org/summer2021-references.
Measuring Children’s Ability to Hear Speech in Different Competing Backgrounds
Young children spend much of their day listening in noise. However, it is clear that, compared with adults, infants and children are highly susceptible to interference from competing background sounds. The ability to listen in competing backgrounds develops slowly over childhood, but the course of development is dependent on the type of background. For example, the developmental trajectory is more pronounced and prolonged for listening for a speech target in a competing speech background than in a steadystate noise background.
Data from infants and school-aged children clearly shows there is substantial improvement in the ability to hear speech embedded in competing backgrounds between these two time points. However, little is known about how the course of development unfolds during the toddler and preschooler years.
Building on our recent methodology improvements for testing young children, the goal of this study was to examine age-related changes for 2- to 15-year-old children in the ability to detect a word presented in one of two competing background sounds: speech (comprising two competing talkers) or a steady-state noise. Children were tested with an observer-based testing method in which the child is trained to perform a play-based response when the target is heard. Based on the child’s behavior, an experimenter determines, of the two possible time intervals, which interval contained the signal.
Consistent with previous studies, results from our research, published in Ear and Hearing in April 2021, indicate that children had poorer thresholds than adults. Moreover, the child–adult differences were substantially larger for the speech than the noise condition. Based on the 0.05 quantile cutoff from the adult data (the dashed horizontal lines in the figure), adultlike performance was achieved at 6 years of age for the noise condition and at 15 years for the speech condition.
A unique contribution to the literature from our study was that we documented limited improvement in thresholds between 2.5 to 5 years. This finding suggests that the large improvement seen between infants and school-aged children must be the result of auditory development that happens prior to 2.5 years of age. Results from this work also confirm that our testing method can be used to reliably collect behavioral data from toddlers and preschoolers for complex listening tasks.
Now that we have established normative data for this task, future work in our laboratory will measure thresholds for these stimuli with children with developmental disabilities. Albeit limited, previous studies suggest that children with developmental disabilities—specifically children with autism spectrum disorder or Down syndrome—have poorer performance for complex listening tasks than age-matched, neurotypical peers.
Documenting performance across the two background types is expected to advance our theoretical understanding of auditory development as well as pave the way for the creation of new clinical tools for monitoring hearing abilities in children with developmental disabilities. —Angela Yarnell Bonino, Ph.D., CCC-A
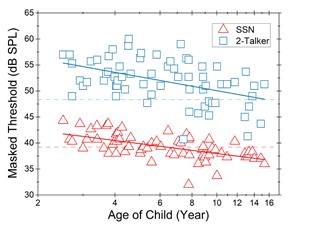
This chart shows individual thresholds as a function of child age (in log10 scale). Thresholds in the speech-shaped noise (SSN) condition and the two-talker speech condition are shown in red triangles and blue squares, respectively. The solid line represents the linear function for the child data: red for the noise condition, and blue for the speech condition. For each condition, the dash line shows the 0.05 quantile cutoff based on adult thresholds.
A 2017 Emerging Research Grants (ERG) scientist generously funded by the General Grand Chapter Royal Arch Masons International, Angela Yarnell Bonino, Ph.D., CCC-A, is an assistant professor in the department of speech, language, and hearing sciences at the University of Colorado Boulder.
The Gene C1ql1 Is Expressed in Adult Outer Hair Cells of the Mouse Cochlea
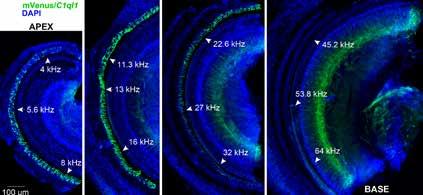
A novel strain of mice has been created such that a fluorescent protein called mVenus, which is similar to the jellyfish protein called green fluorescent protein, is made to be present inside any cell that expresses the gene C1ql1. In the mouse cochlea, this reveals that C1ql1 is expressed in the outer hair cells. A dye called DAPI stains each nucleus blue.
Hearing depends on the transduction of sounds into neural signals by the inner hair cells of the cochlea. The cochlea also has outer hair cells with unique electromotile properties that increase auditory sensitivity, but they are particularly susceptible to damage by intense noise exposure and ototoxic drugs. Although the outer hair cells have synapses that allow communication with neurons that project to the brain, the function of this neuronal circuit and what auditory information it is sending to the brain is unclear. A previously proposed possible function for this circuit is that it may have a function in the detection of auditory pain, which would help us better understand hyperacusis, a condition in which a person experiences pain at a much lower volume level than listeners with typical hearing.
As a postdoc, I had shown that the gene C1q-like 3 (C1ql3) and the C1QL3 protein it creates are important in allowing neurons to communicate with one another in the brain. Other research found that another gene in the same family, C1q-like 1 gene (C1ql1), is expressed specifically in outer hair cells, and not inner hair cells.
These findings prompted a new investigation into the possible functions of the C1QL1 protein in the auditory system and a possible role at the synapses that may be contributing to the perception of auditory pain. We set out to investigate the function of C1QL1 in the cochlea using a mouse model where the gene is knocked out and no C1QL1 can be made. We hoped to find that removing this gene would provide evidence that the outer hair cell circuit to the brain contributes to the perception of auditory pain.
Surprisingly, we found C1QL1 expression in the cochlear tissue of adult mice, but not in neonatal or developing mice, indicating that the protein is not involved with the development of any aspect of the auditory system. This developmental regulation is surprising as both C1QL1 and the related C1QL3 have synaptogenic functions—the formation of synapses between neurons in the brain, especially during development—but C1QL1 expression is absent during the primary postnatal cochlear period of synaptogenesis. This suggests that the C1QL1 protein is not required for initial synaptogenesis in the cochlea. We also did not find that the absence of C1QL1 altered an animal’s apparent perception of auditory pain.
A promising future direction is to conduct similar experiments in older animals, as we found clues that the C1QL1 protein contributes to the maintenance of the outer hair cell synapses. Our research also alerts the larger cochlea scientific community about the expression of the C1ql1 gene specifically in outer hair cells, which may be useful for future experiments designed to better understand the function of these mysterious cells critical for hearing. —David Martinelli, Ph.D.
A 2019 ERG scientist generously funded by Hyperacusis Research Ltd., David Martinelli, Ph.D., is an assistant professor of neuroscience at the University of Connecticut Health Center.
Creating Complex Virtual Environments to Potentially Help Characterize Dizziness Symptoms
Persistent postural-perceptual dizziness (PPPD) is typically defined as having symptoms of dizziness and imbalance for three months or longer when lab test results cannot pinpoint a cause. In collaboration with researchers at the University of Haifa in Israel, our lab investigated two different virtual reality paradigms in an attempt to shed light on the mechanisms underlying PPPD.
In our first study published in the Journal of Vestibular Research in March 2021, we asked people with PPPD and healthy controls to stand in a heel-to-toes position, or one foot in front of the other in a line (“tandem”). Using a head-mounted display (HTC Vive), we showed white dots against a black background, like stars in a night sky, that were static or moving during 20-second trials. On half the trials, participants performed a standard mental task (subtracting by units of three). We compared the two groups in terms of changes in postural sway and head movement in response to these tasks.
The results showed that people with PPPD moved their head and swayed more than the controls on easier tasks, but this difference decreased as the challenge increased. Healthy controls demonstrated increased movement as task difficulty increased, while people with PPPD did not. It may be that patients with PPPD minimize their head movement to facilitate better stability overall.
Our second paper published in the Journal of NeuroEngineering and Rehabilitation also in March 2021, involved a translation of a well-known dynamic test of balance and fall risk prediction, the Four-Square Step Test, into a virtual environment. The traditional FSST is typically conducted in a well-lit room with no visual distractions, and it only measures duration (speed in seconds). We showed that the FSST-VR allows for modification of the visual environment and measures duration as well as identifying movement strategy and quantifying head movement.
People with PPPD and healthy controls performed the FSST task inside a virtual subway scene with three levels of visual complexity. Performance duration of both real and virtual tests was similar between groups. We did see moderate associations between head kinematics and selfreported overall anxiety, balance confidence, and dynamic balance that differed between groups, specifically during high visual load environments.
Namely, in the PPPD group, increased anxiety, reduced balance confidence, and slower speed on the Timed Up & Go test (sitting in a chair, standing up, walking to a point 10 feet away, walking back, and sitting again) were associated with a smaller range of head motion. This suggests that people with PPPD who are more anxious and less confident limit their movement, adopting a “high risk” stiff-motion strategy. In contrast, controls who are more anxious and less confident were associated with the opposite tendency—an increased range of motion.
Findings from both studies need to be replicated in larger groups of patients. The portability and simplicity of the head-mounted display setup may allow for a simple clinical translation and potentially serve as a differential diagnosis for PPPD and other vestibular disorders. —Anat V. Lubetzky, Ph.D.
A 2019 ERG scientist, Anat V. Lubetzky, Ph.D., is an assistant professor in the physical therapy department at New York University.
For references, see hhf.org/summer2021references.
Support our research: hhf.org/donate.
Study Explains “Cocktail Party Effect” In Hearing Impairment
Plenty of people struggle to make sense of a multitude of converging voices in a crowded room. Commonly known as the “cocktail party effect,” people with hearing loss find it’s especially difficult to understand speech in a noisy environment.
New research suggests that, for some listeners, this may have less to do with actually discerning sounds. Instead, it may be a processing problem in which two ears blend different sounds together—a condition known as binaural pitch fusion.
The research, coauthored by scientists at Oregon Health & Science University (OHSU) and VA Portland Health Care System, was published in the Journal of the Association for Research in Otolaryngology in April 2021.
Lina Reiss, Ph.D., the study’s lead author, attributes these difficulties to abnormally broad binaural pitch fusion in people with hearing impairment. The new study suggests that, for people with hearing impairment, fusing of different sounds from both ears leads to sound blending together in a way that is often unintelligible.
Reiss, who has a hearing loss herself, previously coauthored research in 2017 that first demonstrated broad binaural pitch fusion in hearing impairment. Together with her other 2017 study showing blending of the fused pitches, Reiss’s investigations suggested the possibility that similar fusion and blending could occur with sounds in speech.
To test this theory, the OHSU and VA team recruited 11 people with typical hearing and 10 with hearing loss. Participants were fitted with headphones in a doublewalled, sound-attenuated booth. Two vowel sounds were played simultaneously through the headphones, with a different vowel sound played to each ear, and with voice pitch varying between male and female voices. Participants were then asked to respond on a touchscreen to identify the specific vowel sounds.
Using statistical analysis, the investigators definitively revealed that people with hearing loss experienced an abnormal fusion of speech across both ears, even for different voice pitches. When different vowel sounds were fused, participants heard an entirely new vowel sound. For example, the vowel “ah” (as in “hot”) spoken by a female talker would fuse with the vowel “ee” (as in “heed”) spoken by a male talker, and be heard as “eh” (as in “head”).
“Abnormal binaural fusion may provide a new explanation for the difficulties that hearing-impaired listeners have with understanding speech in multitalker environments,” the authors conclude in the paper.
Reiss calls it a breakthrough, suggesting the possibility of new therapies to improve the perception of speech among the millions of people worldwide with hearing impairment. “This suggests more targeted rehabilitation strategies to improve speech perception in noise,” she says. —Erik Robinson
This is adapted from an OHSU press release, at news.ohsu.edu. Lina A.J. Reiss, Ph.D., is an associate professor of otolaryngology–head and neck surgery, and of biomedical engineering, in the Oregon Hearing Research Center at the OHSU School of Medicine. A 2012–13 ERG scientist, Reiss wrote about her experience with hearing and balance issues in the Summer 2020 issue of Hearing Health magazine.