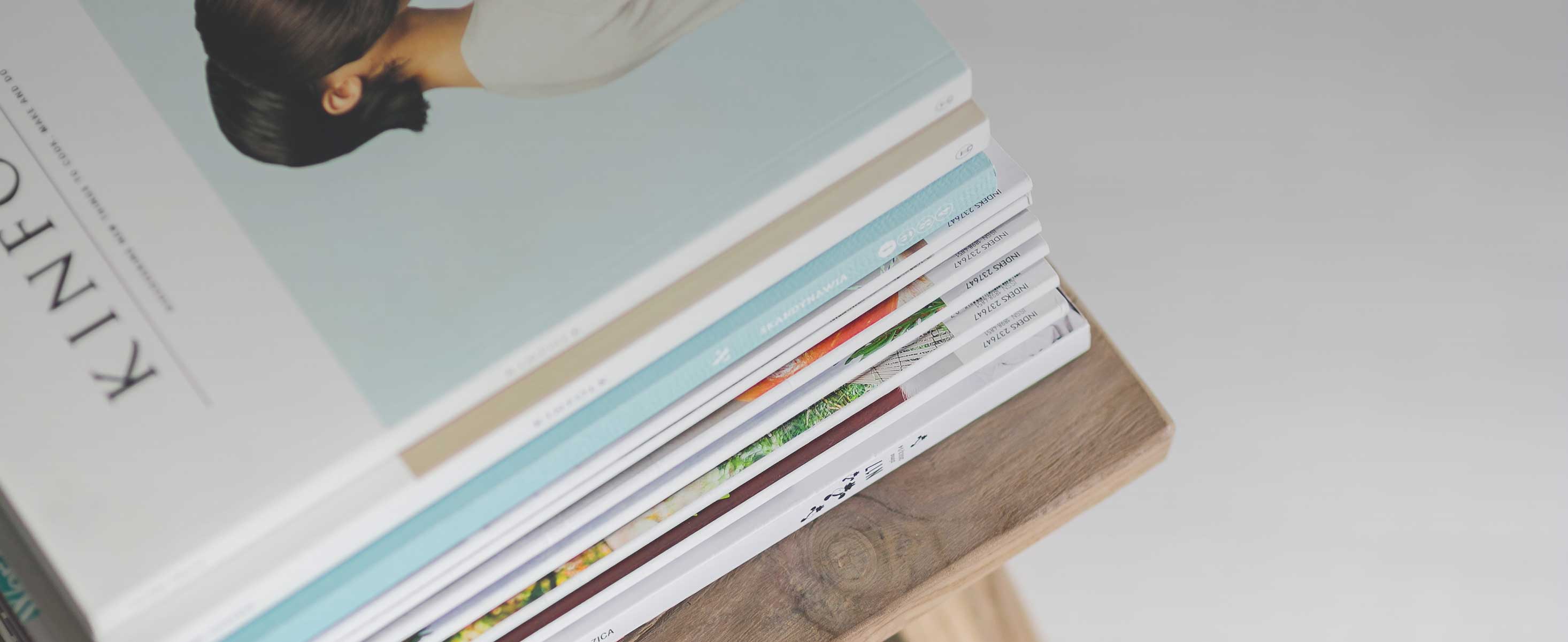
5 minute read
Developmental anomalies of the human stapes
Thaís Gomes Abrahão Elias, MD, PhD1, Felipe Santos, MD2
1 Department of Otolaryngology, Universidade Federal de São Paulo/Escola Paulista de Medicina (UNIFESP/EPM), São Paulo, SP, Brazil. 2 Department of Otolaryngology–Head and Neck Surgery Massachusetts Eye and Ear, Harvard Medical School, Boston, Massachusetts, USA.
Conductive hearing loss can be the result of congenital hearing bone (ossicle) malformations that are estimated to be present in one per 15,000 births1. The stapes is the smallest bone in the human body. It is shaped like a stirrup and conducts sound vibrations from the incus to the inner ear. It is composed of a head or capitulum, which articulates with the incus through the incudo-stapedial joint, and two limbs, or crura, that connect to a footplate. The footplate is the base of the stapes that sits in the oval window connected to the otic capsule by an annular ligament. The annular ligament allows vibration of the footplate thereby transfering sound to the scala vestibuli. Congenital malformations of the stapes can result in conductive hearing loss. Recent animal studies2-9 have begun to shed light on how the ossicles—including the third bone of hearing, the stapes—are formed. The stapes can be divided into anatomic subunits including the capitulum, crura and inner footplate that are of neural crest origin, and the outer footplate, annulus and oval window that are of mesodermal origin5,9. We recently completed a study to determine if human stapes abnormalities can be explained by disruptions in the known dual neural crest and mesoderm origin of the stapes. In order to conduct this study, we identified temporal bones with congenital ossicular anomalies in the Massachusetts Eye and Ear temporal bone collection. The clinical history was collected during life through enrollment in the National Institute on Deafness and Communication Disorders (NIDCD) National Temporal Bone, Hearing and Balance Pathology Resource Registry. Each case could be classified into one of four malformation types based on our current understanding of the embryologic origin of the subunits of the stapes and timing of development. Figure 1 shows a representative example of each malformation by type.
In the first pattern, an early disruption of neural crest cell derived stapes subunits affects the development of the mesoderm derived outer footplate, annular ligament and oval window. In the second, there are more restricted developmental anomalies of the neural crest and mesodermal derived subunits. In this second group, the capitulum and crura are dysmorphic or monopodal and, while the footplate may be fixed, the footplate and oval window are otherwise developed. The third and fourth types of malformations exhibit a more restricted dysmorphology, which suggests—albeit not conclusively—the disruption of events occurring later in stapes development than seen in the first and second group. The third group consists of anomalies affecting the endochondral ossification of the neural crest derived portion of the stapes including capitulum, crura and inner footplate. Finally, the fourth and most common category is isolated fixation of the stapes. This points to disruption of an event restricted to the mesodermal derived annular ligament.
Malformations of the human stapes follow consistent patterns of early or late disruptions of the stapes subunits of mesodermal and/or neural crest origin. This categorization can serve to inform radiographic interpretations of stapes morphology. Recognition of anomalies affecting both the neural crest and mesoderm derived subunits point to more global disruptions and, in turn, isolated footplate fixations point to a more restricted process that may be amenable to surgical correction. While the molecular events, including temporal coordination, that lead to a normally formed stapes are not yet fully understood, the observed patterns of human stapes malformation can be consistently classified into one of four patterns of developmental disruption.
REFERENCES
1. J. B. Farrior, “Surgical management of congenital conductive deafness,” Southern Medical Journal. 1987; vol. 80, no. 4, pp. 450–53. 2. Ulrike J. Sienknecht, “Developmental origin and fate of middle ear structures”. Hearing Research. 2013; 301:19 - 26. 3. Jaskoll T, Maderson P. A histological study of the development of the avian middle ear and tympanum. Anat. Rec. 1978; 190: 177-200. 4. Moore KL, Persaud T, Torchia MG. The Developing Human. Clinically Oriented Embryology, 10th edn. Philadelphia: Elsevier. 2015. 5. O’Gorman S (2005) Second branchial arch lineages of the middle ear of wild-type and Hoxa2 mutant mice. Dev Dyn. 2005; 234: 124–31. 6. Smith G. The middle ear and columela of birds. Q J Microsc Sci. 1904; 48:11–22. 7. Noden DM. The role of the neural crest in patterning of avian cranial skeletal, connective, and muscle tissues. Dev Biol. 1983; 96:144–65. 8. Couly GF, Coltey PM, Le Douarin NM. The triple origin of skull in higher vertebrates: a study in quailchick chimeras. Development. 1993; 117: 409–29. 9. Thompson, H., Ohazama, A., Sharpe, P.T., Tucker, A.S., 2012. The origin of the stapes and relationship to the otic capsule and oval window. Dev. Dyn. 241, 1396-1404.