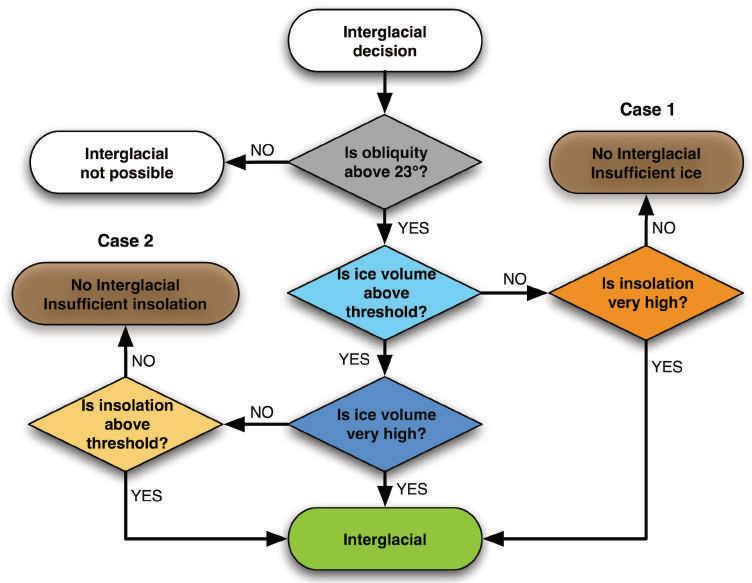
3 minute read
3.5 Conditions for the Dansgaard–Oeschger cycle
events. They also result more susceptible to positive melting feedbacks that increase in strength proportionally to the previous growth of the ice sheets. The feedbacks include rising sea levels, decreasing albedo, warming, dust accumulation, and increased volcanic activity due to ice unloading, leading to increasing CO2 levels. As a result both the chances of deglaciation and the speed of deglaciation when it finally takes place grow with the increase in global ice. The effect on interglacials is double. They changed their shape after the MPT with deglaciations becoming faster than glaciations, acquiring a sawtooth aspect. And their temperature amplitude increased. Some of the latest interglacials are the warmest in over 2 million years, while glacial maxima for the past 650 kyr are the coldest periods probably in 250 million years (Fig. 2.7). It is possible that the increase in amplitude of temperature oscillations since the MPT has contributed to stabilize the long-term average temperature of the planet, that showed a worrisome steep decreasing trend prior to the MPT (Fig. 2.2).
Obliquity opens windows of interglacial opportunity when it is above 23°, and there have been 24 such windows in the past million years (Fig. 2.13a, numbered colored bars). 13 of them produced what we consider an interglacial (!18O values of < 3.7‰; Fig. 2.13c, orange bars), while 11 of them did not (Fig. 2.13 blue bars). The average interglacial frequency is therefore one every 77 kyr. Interglacials cannot exist outside these windows and die a rather quick death when obliquity decreases below 23° (Fig. 2.13a, horizontal line). This is a rule without exceptions. It doesn't matter if 65°N July insolation is high, as it happened between O–22 and 21, or O–16 and 15, underscoring that precession-linked insolation is a secondary factor, as already established.
The second factor in importance is global ice-volume (Fig. 2.13c). It is found that global ice-volume strongly promotes interglacials at obliquity windows when benthic !18O is % 4.56‰ (LR04 stack; Fig. 2.13c, blue line and dark blue circles), but not when it is below that value (Fig. 2.13c, light blue circles). We know global ice-volume is more important than precession-linked high latitude summer insolation because high insolation does not produce an interglacial at times when global ice-volume is low in O–17, O–8, O–5, and O–3, and because high global icevolume can produce an interglacial at times when summer insolation is not particularly high like in O–11. Reaching ice-volume values higher than 4.55‰ benthic !18O takes longer than one obliquity window except when eccentricity is very low, and that is the main reason why obliquity oscillations fail to produce an interglacial. They lack sufficient extrapolar ice to recruit strong melting feedbacks that allow the melting of so much ice in so little time.
The third factor in importance is the one currently believed to be the most important and incorporated as such into climatic models, 65°N summer insolation. It is found that there are two critical levels in precession-linked insolation for interglacial determination. Values of 65°N July summer insolation above 549 W/m2 result in an interglacial at the obliquity window regardless of global ice values (Fig. 2.13b, dark red line and circles). But such values only happen every c. 400 kyr when eccentricity is at the highest values of the 405-kyr cycle. Values above 521 W/ m2 (Fig. 2.13b, orange line and circles) promote interglacials, while values below that hinder them, as in O–23, O–12, and O–2, when an interglacial would have been very useful to Neanderthals, that were crippled by the increasingly harsher glaciation.
The most effective time for a maximum effect from global ice-volume and high-latitude summer insolation differs. Global ice-volume has a maximum effect when it is high at the start of the obliquity window. If it is insufficient to trigger a termination then, it will continue growing, and ice growth within the obliquity window reduces the chance of an interglacial by reducing the time to pro-
Fig. 2.14 Interglacial flow chart
A simple flow chart incorporates the criteria deduced from the past 1 million years of glacial cycle. Interglacials do not take place when obliquity is below 23°. If obliquity is above 23°, interglacials require an ice-volume higher than the equivalent to 4.55‰ benthic !18O or a very high 65°N July summer insolation, above 549 W/m2. If insolation is lower, interglacials require an insolation above 521 W/ m2, or very high ice-volume, around 4.90‰ !18O. There are two scenarios when interglacials fail to take place. The most common is case 1, when ice-volume is not sufficiently high (8 cases). Case 2 is when there is sufficient ice but insolation is too low, associated to very low eccentricity (3 cases) .