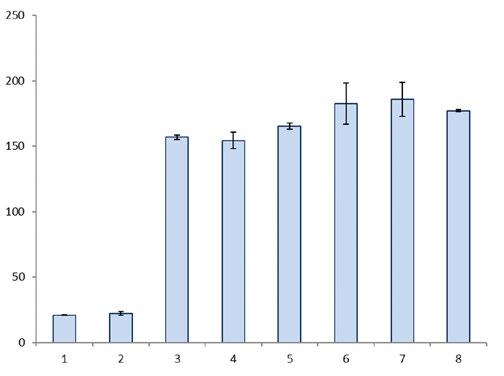
15 minute read
Science: Activities of exogenous enzymes in baked products
Activities of exogenous enzymes in baked products
The activities of various exogenous enzymes in baked products were systematically analyzed from dough preparation, through the baking process and into storage. The texture analysis methods that were developed allowed the events occurring during the development of firmness in the wheat crumb to be differentiated into processes taking place before or after baking. The presence of the enzyme preparations examined during the project showed no detectable functional effects during storage in the crumb that was already formed, due to either previous inactivation or a lack of functionality in the end product.
By Thekla Alpers, Sabina Paulik, Thomas Becker, Mario Jekle, Katrin Reichenberger, Sabine Lutz-Wahl, Lutz Fischer, Gerold Rebholz, Katharina A. Scherf
+Endogenous enzymes occur naturally in wheat flour and yeast, and added exogenous enzymes are responsible for changes in the constituents of wheat during the manufacture of baked goods. The activities of the endogenous enzymes in cereal flours vary depending on the wheat species and variety, environmental influences in the field, and the state of ripeness at harvest time. Exogenous enzymes are used in the manufacture of baked goods to balance out these differences, improve the properties of the dough and end products, and guarantee that the baked goods have a reproducible high quality. The enzymes used for this purpose come mainly from the oxidoreductase class (EC 1), e.g. glucose- or hexoseoxidases, and the hydrolase class (EC 3) such as amylases, xylanases and lipases [1]. The legal basis for using enzymes in foodstuffs
In the EU, adding enzymes to foods is permissible only if they conform to the current implementation status of Regulation (EC) No. 1332/2008. The conditions required for approval are their health safety for consumers, the technological necessity for their use, and the exclusion of consumer deception. According to Regulation (EC) No. 1332/2008, enzymes must be declared in the list of ingredients, with their class name and specific designation, if they are added to foodstuffs for technological purposes in their manufacture, processing, preparation, treatment, packing, transport or storage, and display a technological effect in the end-product. Insofar as enzymes are inactivated during processing and have no technological effect on the end product, they are exempt from the obligation to declare. In the case of baked goods, it is usually assumed that the added enzymes are inactivated as a result of heating during baking, and therefore need not be declared due to the absence of a technological effect in the end product.
Figure 1: Effect of various additives to the extraction buffer to determine a residual activity of the maltogenic amylase from G. stearothermophilus from yeast-leavened white bread using different additions to the extraction buffer (1: no addition, 2: with 1 M of maltose, 3: with 20% (w/v) of maltodextrin, 4-8: with 10% (w/v) of maltodextrin and various extraction times; 1-4: 1-hour incubation time; 5-8: 2-, 3-, 4- and 5-hour extraction time)
Properties and technological effect of amylases
α-Amylases (EC 3.2.1.1) belong to the endo-amylases that split α-(1,4)-glycosidic bonds in starch, and release lower molecular weight α-dextrins. In contrast, maltogenic amylases (EC 3.2.1.133) and malto-oligosaccharide-liberating amylases (e.g. EC 3.2.1.60 and EC 3.2.1.98) are exo-amylases that release maltose and other malto-oligosaccharides such as maltotetraoses or maltohexoses from starch. Pullulanases (EC 3.2.1.41) and iso-amylases (EC 3.2.1.68), which cleave α-(1,6)-glycosidic bonds and thus remove the side-chains of the amylopectin, are also relevant. β-Amylase (EC 3.2.1.2), on the other hand, hydrolyses the α-(1,4)-glycosidic bonds of the non-reducing ends of starch polymers, thus forming β-maltose and β-limit dextrins as the main products.
The effect of amylases during the production of baked goods depends on their thermal stability and specificity, and on the products that are formed. In general, fungal amylases have low thermal stability, and their activity is lost after starch gelatinization during the baking process. On the other hand, a few bacterial amylases are stable
even at high temperatures, and can thus still be active after the baking process [2]. Because of the low endogenous α-amylase activity in wheat flours, one aim of the addition is to achieve an α-amylase-catalyzed increase in the content of fermentable and reducing sugars as a substrate for yeast or sourdough fermentation. However, the essential effect of α-amylase is to degrade starch polymers during gelatinization, and thereby influence the volume of the baked product and the crumb properties concerning softness and pore development. As a side-effect, more reducing sugars are available for the Maillard reaction, and thus for reactions yielding flavor and color.
The addition of amylases delays staling during storage (anti-firming effect). The mechanism at a molecular level has not yet been definitively explained. The fact that dextrins retard the retrogradation of amylopectin, or that modified starch itself displays different properties during retrogradation as a result of the α-amylase activity, are being discussed.
The aim of Research Project AiF (German Federation of Industrial Research Associations) 19543 N was to systematically analyze the activities of various endogenous and exogenous enzymes relevant in the manufacture of baked goods from dough preparation and processing to the end product, and during the storage period.
The thermal stability of various enzymes in the dough matrix
In the Department of Biotechnology and Enzyme Science at Hohenheim University, various enzyme assays were carried out to detect amylase, xylanase, glucose-oxidase and lipase activities, and to intercompare them in their sensitivity and applicability to detect possible residual activities in baked goods. The most suitable enzyme assays were then optimized, where necessary, with regard to their sensitivity. In this process, for example, it was possible to increase the sensitivity of the Betamyl-3 assay, which is used to detect exo-amylase activities, by more than 60-fold by increasing the assay run time and adjusting relevant parameters. After sufficiently sensitive activity assays for all the relevant enzyme classes had been found, a targeted search for possible residual activities became possible.
Among other things, the residual activity of the maltogenic amylase from Geobacillus (G.) stearothermophilus was studied in yeast-leavened white bread. First of all, using an enzyme dosage of 100 mg/kg (relative to the amount of flour), a new sample preparation method was developed to determine the amylase activity in white bread. For this purpose, white bread was freeze-dried, ground and extracted with buffer. Next, various additives to the extraction buffer were tested, e.g. maltose and maltodextrin. Figure 1 illustrates the specific activities achieved under the various conditions. It was possible to increase the extraction efficiency by more than 7-fold by adding 10% (w/v) of maltodextrin. Increasing the maltodextrin concentration to 20% (w/v) did not yield any further increase in extraction efficiency. In the last step, the extraction time was examined. In this way, it was possible to increase the extraction efficiency by a further 16% by lengthening the extraction time from one hour to three hours. This provided a suitable sample preparation method to determine the residual activity of maltogenic amylase in yeastleavened white bread. White breads were baked with three different dosages of maltogenic amylase (10, 50 and 100 mg/kg, relative to the amount of flour), and a white bread without added enzyme as a reference. Next, the white breads were examined for residual activity. This determination showed an average residual activity of 17.8% of the added maltogenic amylase activity. The standard deviation on all the measurements carried out was 1.2% [3].
In addition to maltogenic amylase from G. stearothermophilus, other amylases, lipases and glucose-oxidases from the baked products industry were examined with regard to their stability in dough under baking conditions. First of all, a method for extraction from dough was developed for each enzyme. These investigations took place in a speciallydeveloped heating method on a laboratory scale, which enabled the temperature profile inside a white bread loaf during the baking process to be imitated. This was done by heating each dough (5 g) in a glass vessel in an oil-bath at 110°C for 24 minutes, after which it was cooled. This method was used only to estimate the stability of the individual enzymes. This allowed a residual activity of 12.0% to be determined for the maltotetragenic amylase from Pelomonas saccharophila (formerly Pseudomonas saccharophila). Only very small residual activities (0.7% and 1.4%) were determined for the α-amylases from Bacillus subtilis and Aspergillus niger. Five different lipases were tested, including those from Thermomyces lanuginosus, Fusarium culmorum and Fusarium heterosporum. Of these, only the lipase from Thermomyces lanuginosus showed a small residual activity of 2.5%. In addition, four glucose- or hexose-oxidases from Chondrus crispus, Aspergillus niger and Trichoderma reesei were examined, from which only very small reactivities of less than one percent were detectable.
The influence of various amylases on the sugar content in bread crumb
The effects of adding various amylases on the release of mono-, di- and oligosaccharides was examined based on the sugar spectrum in the bread crumb of model toast breads two hours after baking, and after storage for up to 96 hours. Without the addition of amylases, no change in the contents of glucose, fructose, saccharose (sucrose), maltose, maltotriose and maltotetraose was apparent over a storage duration of up to 48 hours (Figure 2 A). When a bacterial α-amylase from Bacillus amyloliquefaciens was
added, a distinctly changed spectrum of mono-, di- and oligo-saccharides was evident compared to control bread without any addition (Figure 2 B) [4]. In addition to the sugars already present two hours after baking, maltotetraose was detected in the crumb after 22 hours of storage time, and maltopentaose, maltohexaose, maltoheptaose and maltooctaose after 48 hours. This preparation led to a 3.9-fold increase in maltose content compared to breads without any addition. The maltotriose content rose significantly, from 17.4 mg/g two hours after baking to 46.6 mg/g after a storage time of 96 hours. Maltotetraose also increased significantly from 6.9 mg/g after 22 hours to 43.0 mg/g after a 96-hour storage time. Moreover, the content of the sugars first identified and quantified after 48 hours of storage rose significantly up to 96 hours after storage: maltopentaose rose from 21.4 mg/g to 34.6 mg/g, maltohexaose from 31.4 mg/g to 48.0 mg/g, and maltoheptaose from 31.1 mg/g to 52.0 mg/g. A scarcely measurable amylase activity of 0.07 nkat/g in the crumb was detected by using the enzyme activity assay.
The use of a maltotetragenic amylase from Pelomonas saccharophila also caused a clearly recognizable change in the contents of mono-, di- and oligosaccharides compared to the control (Figure 3 C) [5]. This preparation also showed only a very small activity of 0.6 nkat/g in the crumb. Nevertheless, after a 48-hour storage time, maltopentaose, maltohexaose, maltoheptaose and maltooctaose were detected in addition to the sugars already detectable after two hours. A characteristic feature of the effect of this preparation was the significant increase in the maltotetraose content from 2.1 mg/g (after two hours) to 29.5 mg/g (after 96 h), with the result that maltotetraose was the main product from this time onwards.
In the case of breads manufactured with maltogenic α-amylase from Geobacillus stearothermophilus, the sugars glucose, fructose, saccharose (sucrose), maltose, maltotriose and maltotetraose were identified two hours after baking (Figure 3 D) [5]. In these cases, scarcely any changes in sugar contents were detected during storage. Only the maltotetraose content rose significantly from 0.6 mg/g (after two hours) to 22.9 mg/g (after 96 hours) during the observed period of time. This could be associated with the measured residual activity of 5.7 nkat/g, corresponding to 14% of the activity originally added. However, due to
Figure 2: Sugar content of the model wheat breads without the addition of an exogenous amylase (A), with the addition of a bacterial α-amylase from Bacillus amyloliquefaciens (B), with the addition of a maltotetragenic amylase from Pelomonas saccharophila (C), and with the addition of a maltogenic α-amylase from Geobacillus stearothermophilus (D). Samples were taken two hours, 22 hours, 48 hours and 96 hours after baking. Values are stated as the mean value ± standard deviation (n = 4). Significant differences between the various time points for each sugar are indicated by asterisks (p < 0.05). Dry matter = relative to dry matter.
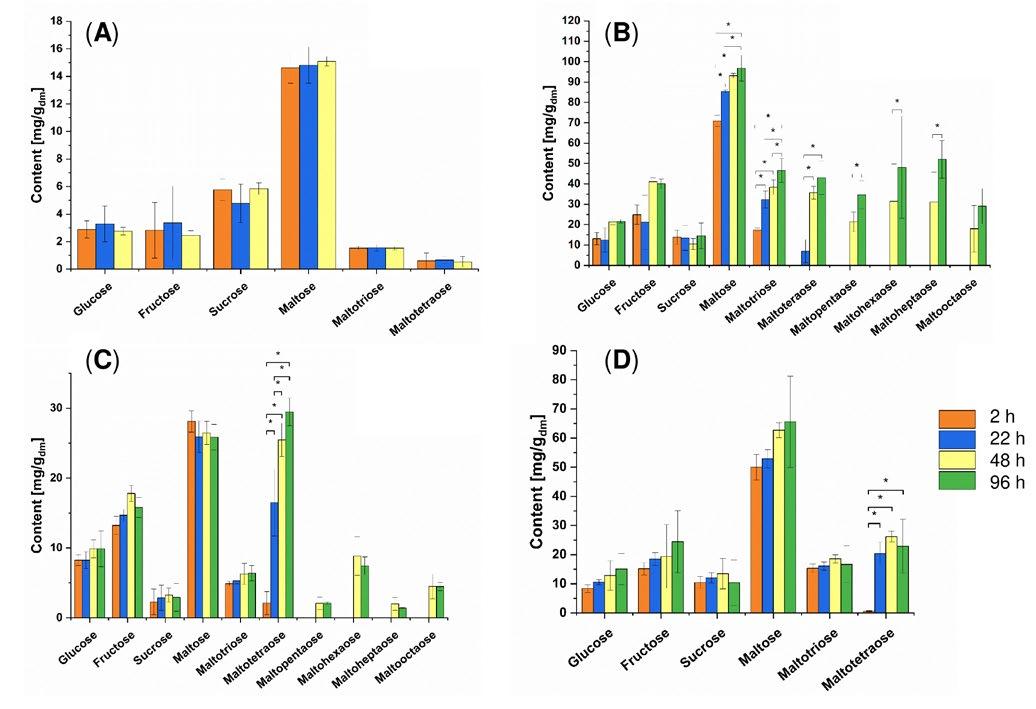
the altogether comparatively slight changes in the sugar spectrum, no technological activity of the amylases can be assumed here.
Development of texture analysis methods to determine enzymatically-induced bread crumb firming after baking
The research project presented in this paper examined primarily the effects of various exogenous amylases on enzyme-induced bread crumb firming after baking. Various novel texture analysis methods were developed to elucidate the causes of the anti-firming effect of exogenous amylases in baked products. The standard crumb texture analysis by means of a Texture Analyser comprises compressing two slices of bread, each 12.5 mm thick, by 40% in two cycles. In this process, among other things, information is obtained about the crumb hardness and crumb elasticity. What is analyzed here is the response of the crumb structure resulting from the proofing, baking and storage process to the pre-specified deformation. Thus all the influencing parameters that affect the crumb structure and material (e.g. gas volume fraction of the crumb, pore pattern, crumb matrix firmness) contribute to the texture result.
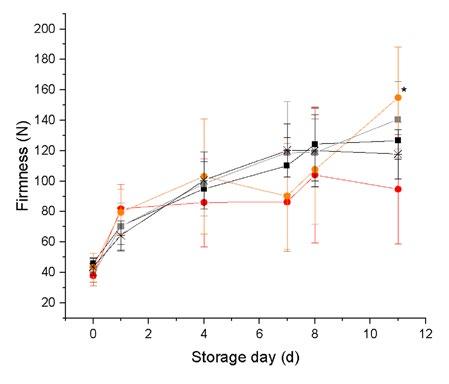
ADVERTISEMENT
Figure 3: Crumb firmness of reengineered crumb pellets (-✲-) with no additives, (-■-) with the addition of a maltogenic amylase from Bacillus stearothermophilus, (-■-) with the addition of the
determined residual activity of a maltogenic amylase (29%) in the
crumb after heating to 90°C, (-●-) with the addition of a bacterial α-amylase, and (-●-) with the addition of the determined residual
activity of a bacterial α-amylase from Bacillus subtilis (5%) in the crumb after heating to 90°C. The values are stated as the mean value ± standard deviation (n = 3). Significant differences between the various time points are identified by asterisks (p < 0.05).
www.heuft1700.com
THIS IS BAGUETTE.more
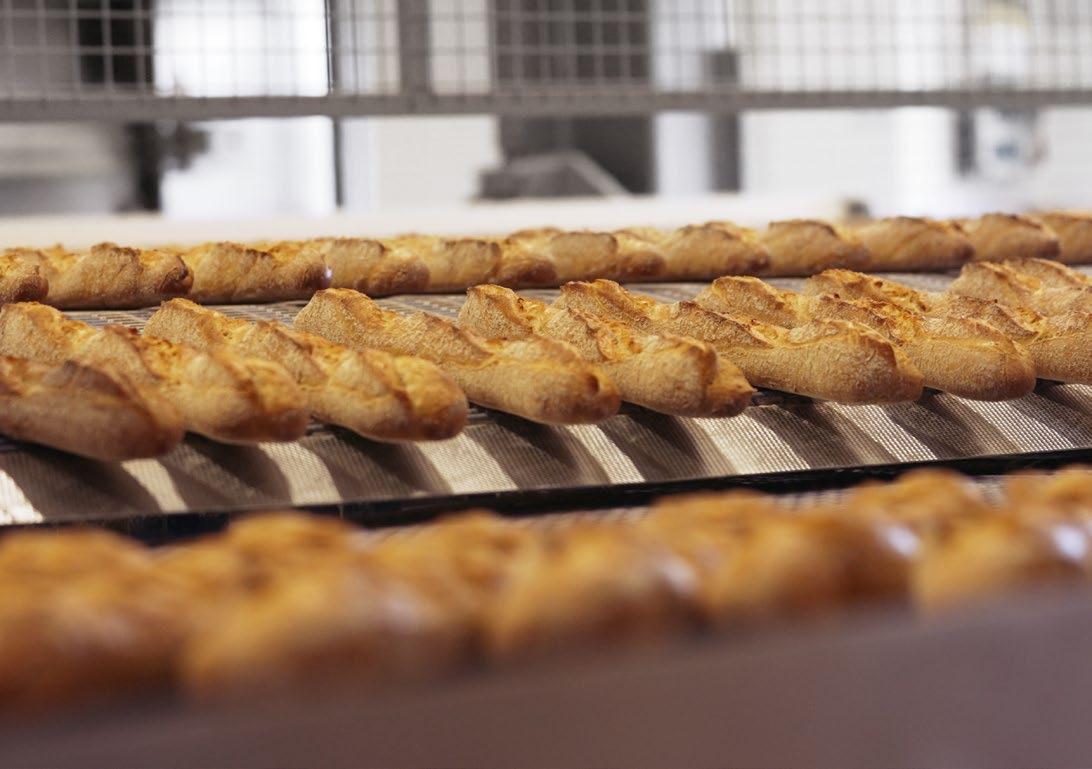
BAKED IN AN INDUSTRIAL HEUFT THERMAL OIL OVEN.
Theoretically, therefore, if amylases are used, a changed crumb firmness could be due, for example, to a changed pore pattern resulting from an increased provision of fermentable sugars. Furthermore, theoretically, a modified crumb formation process based on the amylolytic degradation of starch polymers by amylases during the fermenting and baking process, or even amylolytic activity after baking, also come into question as the cause. In the context of the research project, the current crumb texture analysis method was further developed to achieve the stepwise control and reduction of these material-related dependences and complexities.
In a first step, the dependence of the pore pattern on the yeast was overcome by producing chemically-leavened breads. To standardize the deformation of the material, the sample weight of crumb material was kept constant, and uniaxial compression was carried out until the material was pore-free. However, to study the influences of constituents on crumb firming behavior, it was necessary to introduce hypothetically crumb-modifying materials after the baking process. The challenge in this respect is to distribute the additives homogeneously into the crumb structure that has already formed. For this purpose, a process for the texture analysis of reengineered, pore-free crumb pellets was developed at the Chair of Brewing and Beverage Technology. This involves initially freeze-drying a chemically leavened crumb, then grinding it in a thermally gentle process, and finally subjecting it to defined rehydration and pelleting. The resulting homogeneous, pore-free, reproducible pellets can be subject to storage tests in a defined environment, and texturally analyzed. The decisive advantage of this methodology lies in the rehydration of the crumb material. Moreover, it allows constituents to be incorporated into the crumb, which are consequently able to develop their effect exclusively during storage. Every influence whatsoever on the crumb during the dough-crumb transition is excluded by using crumb material produced in a standardized way. The methodology was used to analyze the effects on firming behavior of the possible presence of (residual) maltogenic amylase from Bacillus stearothermophilus and of an α-amylase from Bacillus subtilis in a standard wheat crumb. In this respect, pellet storage experiments (Figure 3) showed that the aging behavior of crumb pellets supplemented with a maltogenic amylase from Bacillus stearothermophilus after baking does not differ from the firming behavior of a standard crumb (firming = crumb solidification during the storage time). Thus the hypothetical presence of this active maltogenic amylase during storage does not appear to have any functionality-modifying influences on the crumb material. In contrast to this, there would be a tendency for reduced firming behavior to be observed in the event of 100% activity of an α-amylase from Bacillus subtilis in the baked product.
Authors
Thekla Alpers1, Sabina Paulik1, Thomas Becker1, Mario Jekle1,2 , Katrin Reichenberger3, Sabine Lutz-Wahl3, Lutz Fischer3 , Gerold Rebholz4, Katharina A. Scherf4,5
1Chair of Brewing and Beverage Technology, TUM School of Life Sciences, Technical University of Munich, Freising, Germany 2Institute of Food Science and Biotechnology, Department of Plant-based Foods, University of Hohenheim, Stuttgart, Germany 3Institute of Food Science and Biotechnology, Department of Biotechnology and Enzyme Science, University of Hohenheim, Stuttgart, Germany 4Leibniz-Institute for Food Systems Biology at the Technical University of Munich, Freising, Germany 5Department of Bioactive and Functional Food Chemistry, Institute of Applied Biosciences, Karlsruhe Institute of Technology, Karlsruhe, Germany Contact: katharina.scherf@kit.edu
Literature
1. Goesaert, H., Brijs, K., Veraverbeke, W.S., Courtin, C.M., Gebruers, K., Delcour, J.A. (2005): Wheat flour constituents. How they impact bread quality, and how to impact their functionality.
Trends in Food Science and Technology, 16, 12-30. doi: 10.1016/j.tifs.2004.02.011 2. Bowles, L.K. (1996) Amylolytic enzymes. In: R.E. Hebeda, H.F. Zobel (Eds.), Baked goods freshness: Technology, evaluation, and inhibition of staling. Marcel Dekker, New York, NY, pp. 105-129 3. Reichenberger, K., Luz, A., Seitl, I., Fischer, L. (2020): Determination of the direct activity of the maltogenic amylase from Geobacillus stearothermophilus in white bread. Food Analytical
Methods 13: 496-502. doi: 10.1007/s12161-019-01673-7 4. Rebholz, G.F., Sebald, K., Dirndorfer, S., Dawid, C., Hofmann, T.,
Scherf, K.A. (2021): Impact of exogenous α-amylases on sugar formation in straight dough wheat bread. European Food Research and Technology 247, p. 695-706. doi: 10.1007/s00217020-03657-y 5. Rebholz, G.F., Sebald, K., Dirndorfer, S., Dawid, C., Hofmann, T.,
Scherf, K.A. (2021): Impact of exogenous maltogenic α-amylase and maltotetraogenic amylase on sugar release in wheat bread. European Food Research and Technology 247: 14251436. doi: 10.1007/s00217-021-03721-1 6. Paulik, S. and Jekle, M. (2019): Novel approach to investigate the mechanical properties of crumb matrix during storage –
Re-engineering of gas-free crumb pellets. Food Chemistry, 288, 333-340. doi: 10.1016/j.foodchem.2019.03.014