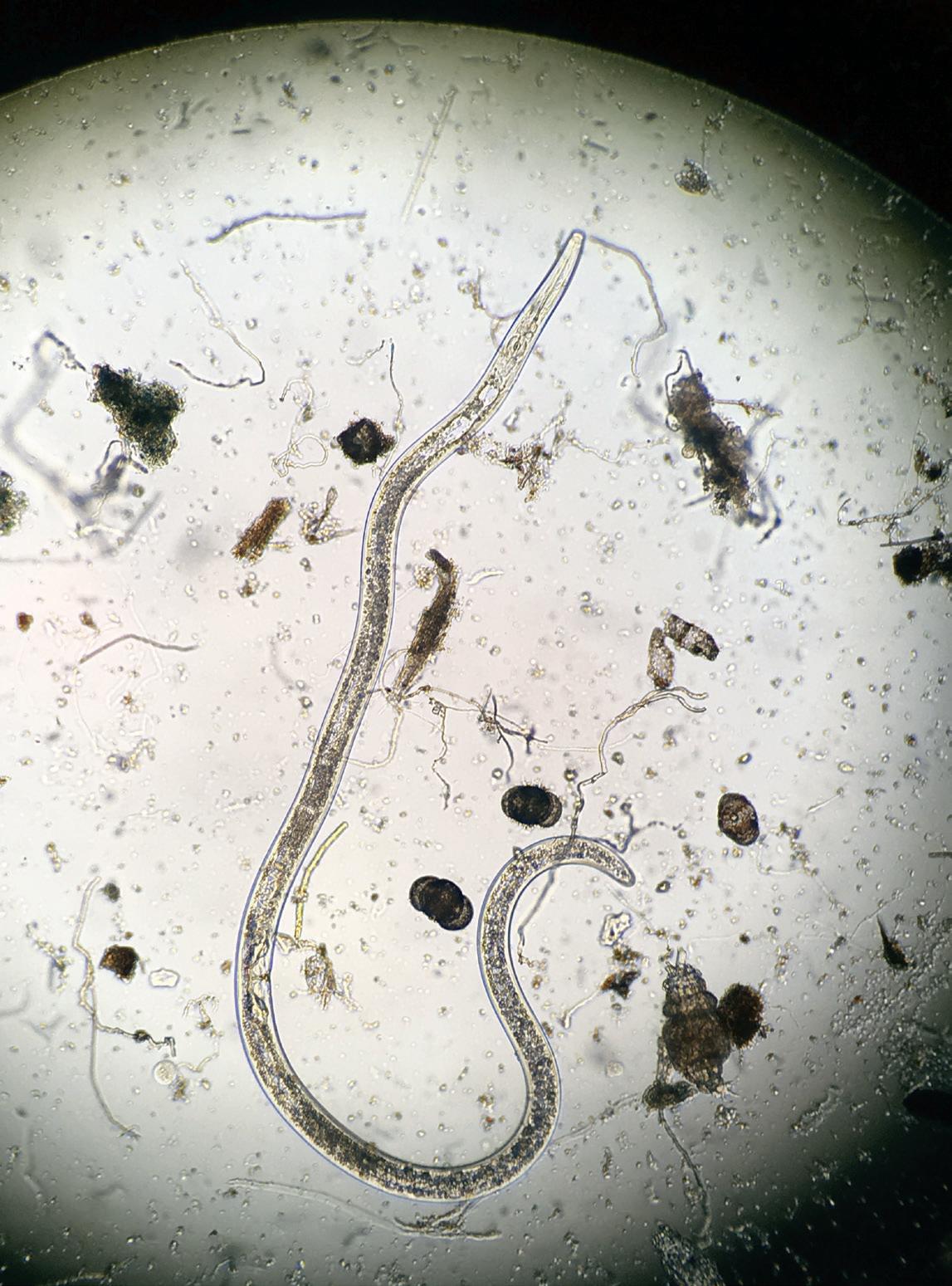
18 minute read
Nematodes:
A Challenge to Manage in Southern Turfgrasses
By Dr. Bruce Martin, Professor Emeritus – Clemson University
Advertisement
Adapted from: Bruce Martin. 2016. Nematodes in southern turf grasses. Golfdom 73 (5)
Nematodes are an understudied category of pests in turfgrasses, even though the tiny ‘roundworms’ are among the most abundant of animals on Earth. Nematodes inhabit many habitats and niches, and include free-living forms, truly aquatic species, and the parasites. The parasitic nematodes can be pests of invertebrate animals, such as insects as well as vertebrate animals, including humans. They began to be recognized as significant pests in the South after World War II when interest in golf accompanied the vibrant economy. Florida was the first state to report nematode injury in bermudagrass turf (Christie, et al 1954).
Since that time over 60 years ago, locations throughout the United States reported on nematodes associated with damaged turf. Initial reports were usually surveys of the genera and species of nematodes associated with various turfgrasses, and included familiar nematodes such as Belonolaimus longicaudatus (sting), Hoplolaimus galeatus (lance), Trichodorus and Paratrichodorus spp. (stubby root), and others such as Helicotylenchus (spiral), Criconemella (ring), Criconemoides (sheathoid), Hemicycliophora (sheath), Tylenchorrhynchus (stunt), Meloidogyne (root-knot) and Hypsoperine (false root- knot nematode). Surveys are still important, with the understanding that identification now utilizes traditional and modern molecular identification tools.
For example, a survey was conducted in North and South Carolina golf courses (Zeng et al. 2012) which revealed 24 nematode species belonging to 19 genera and 11 families. Helicotylenchus dihystera, Mesocriconema xenoplax (a ‘ring’ nematode), Hoplolaimus galeatus, Tylenchorhynchus claytoni (a ‘stunt’ nematode), Belonolaimus longicaudatus, Meloidogyne graminis and Paratrichodorus minor were the most prevalent and abundant species in golf course turfgrasses in both states. Twelve species were new records of plant parasitic nematodes in turfgrasses in both NC and SC. Further work, such as the development of molecular tools to quickly identify species of root-knot (Ye, et al, 2015), will lead to a better understanding of these particular pests and their impact on golf course turfgrasses and turfgrass in general.
These reports and the experiences of plant scientists and turfgrass managers confirm the role of nematodes as significant but often overlooked pests in turf. As soilborne pests, nematodes are ‘out of sight’ and all too frequently ‘out of mind’. These pests are root parasites and their effects on turf can mimic other causes of weak turf, which likely leads to uncertainty in diagnosing their true nature as primary pathogens. Sometimes nematodes are never recognized as the true cause of a problem. In most state labs, the primary plant problem diagnostic lab is not coupled with nematode identification services. Nematode identification and enumeration require different techniques and experiences than those typically employed in diagnostic labs for fungal pathogens. This means that the diagnostician should also be trained in nematology and be familiar with the symptoms they can induce and have an appreciation of the potential contribution of nematodes as pests. In any case, clients of nematode identification labs should develop a relationship with the service and provide as thorough information as possible to aid the labs in making the right diagnosis. This is especially important in the Southern states where nematodes are recognized and known to be important. Because of the warming climate, nematodes are increasingly recognized in other states in cool season grasses where heat and drought stress are more common in summer months.
Alabama turfgrass managers are very fortunate to have a nematology lab in their state. Located at Auburn University, the Alabama Cooperative Extension System operates a Plant Diagnostic Lab that includes the services of nematode testing providing identification and numbers of each type per sample. The nematology lab performs soil nematode analysis for $10 per sample. Your local ACES office can provide the test kit boxes and forms for such tests.
In the Southern states, arguably, the most important nematodes include sting, root-knot species, and lance nematodes. This is not to say that others, if counts from assays are high enough, cannot also cause significant damage or contribute to damage from other stresses. These might include nematodes such as stunt, stubby root, ring and spiral nematodes (Dickerson, et al, 2000).
Generally, nematodes are broadly recognized as ‘ectoparasites’ and ‘endoparasites’. Ectoparasitic nematodes remain outside the host roots and feed by piercing plant cells with their needle-like stylets. Endoparasitic nematodes actually penetrate the plant roots and can move through root systems (‘migratory endoparasites’), while feeding. Others, such as the root knot nematodes, can penetrate and remain immobile (‘sedentary endoparasites’). Lance nematodes are an example of ‘migratory’ semi-endoparasites which can occur in root system as well as the soil around the roots. Knowing these details has implications on sampling and also on difficulty in control with traditional nematicides.
Sting nematodes
Belonolaimus longicaudatus is a large nematode, reaching sizes of about 1/8-inch long when mature (Figure 1). Size may particularly matter in the case of the stylet of this nematode, which is long and allows the nematode to feed deep within root tissues (Figure 2). This nematode feeds on root meristem tissues (root tips) and this fact likely helps explain why it causes damage even at low threshold numbers (most labs indicate a damage threshold of about 20 per 100 cc of soil). Feeding by this nematode, when numbers are high enough, leads to a shallow, stunted root system. Wounds from the nematode feeding allow other soil organisms to colonize and further debilitate the host plants (Figure 3). Sting nematode is an ectoparasitic feeder: its body remains outside of the host tissues as it feeds, and it can move short distances to reach other roots for further feeding. As an ectoparasite, it is presumed to be more susceptible to nematicides. Sting nematode is a southern nematode in the United States: it does not survive in frozen soils for long periods of time, and also is limited to soils of at least 80% sand. Belonolaimus and related species also occur in other parts of the world in turf and are recognized as major pathogens. It has been pointed out before (Peacock, 1989), and is obvious, that sand-based rootzones are naturally ideal habitats for sting and other nematodes. Besides being good habitats for the nematodes themselves, sand-based rootzones are inherently nutrient deficient and prone to drought stress. Therefore, root parasites, such as nematodes, are particularly important in sandy soils.

FIGURE 1: Sting nematode adult female

FIGURE 2: Sting nematode head showing stylet

FIGURE 3: Stubby root nematode damage on St. Augustinegrass
Root-knot nematodes
Nematodes in the genus Meloidogyne are the root-knot nematodes. At least 5 species have been identified in turf, including M. graminis, M. incognita, M. naasi, M. microtyla, and M. marylandi. In bermudagrass turf, it appears M. graminis and M. marylandi are commonly identified. M. naasi and M. microtyla are sometimes identified in cool season grasses as well as warm season grasses. Root-knot nematodes are sedentary endoparasites. The female root-knot nematodes spend the majority of their life cycles inside roots. Newly hatched juveniles and males occur outside of the roots when eggs hatch from egg masses that are deposited outside the root surface. Some larvae can hatch inside roots and go on to colonize roots without migration outside the root. Root-knot larvae penetrate roots and induce the plant root cells on which they feed to enlarge and become metabolic sources of food for the nematode rather than for normal plant growth processes. Root-knot females within infected roots swell and become gourd-shaped when mature, producing eggs contained in a gelatinous egg sack (Figure 4); egg sacks and mature female nematodes may break through the root cortex and become visible with a microscope. Results from control trials have also generally shown root-knot nematodes to be more difficult to manage than sting nematodes, probably due to their high reproductive capacity (multiple generations, high counts) per season and the protection their bodies enjoy as endoparasites within roots. Root-knot nematodes are encountered in many soil textures and are not limited to sandy soils. Their damage potential, however, is likely higher in sandy soils due to the inherent higher risk of drought and nutrient deficiency stresses.

FIGURE 4: Gelatinous egg sacks of rootknot nematode on dwarf Bermudagrass
Lance nematodes
Lance nematodes belong in the genus Hoplolaimus; the most commonly encountered lance nematode in turf is H. galeatus although other species such as H. stephanus are recognized recently. More research is needed in specific identification of lance nematode populations in turf, as the morphological differences among some species are not obvious. Lance nematodes are migratory endoparasites in their feeding habits. They move inside roots and outside, and feed seemingly indiscriminately on roots, not necessarily targeting specific regions of roots like sting or stubby root nematodes. In one study in Kansas, it was shown that juvenile lance nematodes were most abundant within the roots, while more adults were encountered outside the root system (Settle, et al 2007 ). Lance nematodes have a high reproductive capacity, and in some locations, counts can easily exceed several thousand per 100 cc of soil. Generally, lance nematodes have relatively high damage thresholds, particularly in Northern states in cool season grasses where environmental stress is less severe than in Southern states.
Nematode Distribution, Sampling and Thresholds
Plant parasitic nematodes are obligate parasites of their host plants. They must feed on these hosts in order to thrive, survive and reproduce. In most cases, we see separate sexes within a species of nematode with males and females. Typically, the development of a nematode from egg to adult is about a month. Similar to insects, as the nematode feeds and grows, it sheds the chitin-based body wall several times to accommodate the increases in size as the nematode grows to adult. Some nematodes, such as the root-knot nematodes, lay high numbers of eggs and their populations can reach very high numbers in favorable conditions. Others, like sting nematodes, may not lay as many eggs, and populations may not reach nearly as high as those with high reproductive capacities. Nevertheless, some of these, like sting in particular, cause significantly more damage per individual nematode.
Recognition of nematodes as pests, observations of their damage and experiments have resulted in publication of ‘damage thresholds’ (Dickerson, et al 2000). These are the numbers of nematodes of a particular species per volume of soil or weight of roots and are used to compare the potential of particular nematodes to cause real damage. Generally, they are reported as numbers of individual worms per 100 cc or per 500 cc of soil or per a certain fresh weight of roots. Threshold numbers are meant to be used as guidelines in assisting a diagnosis concerning the role of nematodes in a particular problem. As general guidelines, the numbers do show relatively how different nematodes compare as to their pathogenicity or ability to cause damage. As surveys and research have improved our knowledge in some cases, thresholds may be refined to include different species or to better reflect the relationship of numbers to potential damage. In Florida, a large stubby root nematode, Trichodorus obtusus, has a lower threshold number than does the smaller and less pathogenic Nanidorus on particular grasses (Crow and Welch, 2004). Trichodorus obtusus has been found in NC and SC at this time, so these states should include this more damaging stubby root nematode (Shaver, et al. 2015) and provide a damage threshold.
For nematode damage thresholds to have their best value, some thought into sampling methods is needed for sampling of soil and root systems. One obvious factor is that nematodes are going to be most abundant where roots are present. Turfgrass roots generally extend to about 4 inches but may be shallower for several reasons and particularly if a nematode problem is present. This is typically the depth of sampling generally recommended, but some nematodes like root-knot seem to be concentrated much more densely in the upper 1–2 inches, so stratified sampling may be appropriate. Also, it is known that sting nematodes migrate to lower soil depths in the heat of summer, so sampling that relies on the upper couple of inches in hot summer months may not reflect the true infestation of the turf site. If samples are taken during the Alabama summer months, then deeper soil profiles (4–5 inches deep) may be needed to detect sting if it is present. However, this also dilutes the sample size and may not accurately reflect root knot populations which remain in the upper inch at highest levels.
Also, because root knot nematodes occur in highest population densities inside the roots, they may be under-estimated using typical sugar flotation/centrifugation extraction methods that most nematode assay service labs currently use. Rather, specialized techniques such as mist extraction (and direct microscopic observation of roots) can be used to better estimate a potential root knot problem. Mist extraction takes typically 3–4 days to extract and count the nematodes, while sugar flotation/centrifugation is quick, less than an hour for extraction. Another technique, the Baermann funnel, can be used for both ecto and endo parasites but also takes more time than sugar flotation/centrifugation.
Nematodes typically are clustered in ‘hot spots’ which may correspond to more favorable soil texture (e.g. sandy areas) for the nematodes. This means that sampling for diagnostic purposes (to answer the question: are nematodes responsible for the symptoms expressed) is different than more routine or systematic sampling that might be done to determine an average population in an area, such as a research plot. Diagnostic samples are recommended to be taken at the margin of visibly damaged patches or areas of turf. The objective is to find damaging species at high levels if they exist. Do not sample dead or extremely thin turf, as nematodes are more likely to be at low populations where their food base is scarce. Rather, sample the margins, where there is still turf but where the turf is showing symptoms (Figure 5). Most labs prefer multiple cores of 1-inch diameter taken at the recommended depth and bulked to provide about 500 cc of soil. The lab will mix and subsample from the composite sample and provide counts of nematode species encountered. If the purpose is to monitor nematode populations in a turf stand, then systematic sampling is typically recommended: areas may be divided into plots (e.g. treated with a nematicide vs. not treated) and multiple cores taken from each area in a zig-zag pattern to provide a good average count. No attention is paid to symptomatic turf in this method, so hot spots as well as healthier turf can be sampled.

FIGURE 5: Localized sting nematode damage; soil samples should be taken at the margins where turf is showing symptoms
Finally, nematode populations also change over time. Generally, populations are going to be highest when plant growth, especially root growth, is favored and may be lower when plant growth is slower or dormant. For bermudagrass in the southern transition zone for instance, root growth is abundant just as bermudagrass is greening up in spring (Figure 6). Soil temperatures are likely still very cool, 50–60° F, and top growth is very slow or almost imperceptible. Nematodes, if present, may already be feeding on these young roots. The same phenomenon occurs in the fall season when soil temperatures dip again as bermudagrass approaches dormancy: new roots appear in September and October but may also continue to be formed in November and early December when frosts have induced semi-dormancy and top growth is suppressed. Typically, a good time to sample to find high levels of nematodes in this region would be April/May or Sept/October months. Sampling for sting nematode in mid or late summer in Alabama, especially the lower half of the state and even in Florida may show lowered populations as soil temperatures are very high, and sting may move to deeper soil profiles and beyond the typical sampling depths. In winter months, counts may also be lower due to nematode mortality.

FIGURE 6: Sting nematode damage on white roots
Nematode Management
Like most pests, options for management include cultural, biological and chemical methods with IPM strategies typically providing the most practical and consistent results. In truth, even in Southern regions where nematode induced stress is high, such as Alabama, most practical management is cultural and is provided by the knowledge and skill of superintendents and other turfgrass managers. These practices include ‘spoon feeding’ of nutrients, hand-watering of symptomatic greens, avoiding stressful verticutting/aerification practices when the root system is so damaged that turfgrass can heave, and other practices. These practices are labor and knowledge intensive! In some cases, even where nematode damage is severe, a turf surface can be maintained to an acceptable quality for short periods of time. The problem is that the risk is high for damage to exceed acceptable levels if the environmental stress becomes too high.
Biological controls include natural products that may suppress nematodes, such as certain extracts of sesame, neem or mustard-based products that release glucosinolate metabolites which are nematicidal. Some biological products are based on microorganisms that are antagonistic to nematodes and perhaps exclude them from their feeding sites or produce metabolites which harm the nematodes in some way. One such product is the bacterium Bacillus firmus strain I-1582, the active ingredient in Nortica (currently unavailable). It is thought that this bacterium is antagonistic to certain nematodes but also may promote plant growth, thereby effectively avoiding some of the stress induced by nematode compromised root systems. Other bacteria can be actual parasites of nematodes, such as species of Pasteuria. These are naturally occurring parasites, with strains that are more or less specific to their particular hosts (e.g. Pasteuria usgae parasitizes sting nematode but not root-knot or lance). As of this writing, these bacteria are not available in a commercial product.
Where nematode damage is chronic and frequently severe, the use of chemical nematicides has provided the best method for suppressing populations and allowing turf to tolerate the stressful months adequately. The old nematicides such as DD (1,3 –dichloropropene and 1,2-dichloropropane), DBCP (1,2-dibromo-3-chloro-propane) and EDB (ethylene dibromide) were used in the late 1950’s, ‘60’s, and ‘70’s to provide cheap, long lasting and effective control of nematodes in turf. It is interesting to read the old literature concerning these and other chemicals. For instance, in one 1978 issue of Golf Business (now Golfdom), the following quote exists: “DBCP ban does not include golf courses”. However, in the 1979 issue, this quote was found: “DBCP turf uses suspended by EPA”. And so it went until in 1984, EDB was canceled and that left only 1,3-dichloropropene (Telone) as the only post plant fumigant nematicide. It was eventually canceled also but found new life as Curfew Soil Fumigant, which is still registered in certain southern states, including Alabama, under 24c (special local need) labels. Also left were certain contact nematicide/insecticides in organophosphate or carbamate chemistry. However, today, there are no more organophosphate nematicides available for turf nematode control.
Obviously the need for effective, safe nematode control products is very high. Since 2016, three nematicides have been marketed for turf: these include fluensulfone, the active ingredient in Nimitz Pro G, marketed by Quali-Pro (currently unavailable). Also, fluopyram, the active ingredient in Indemnify, developed by Bayer was registered and remains available. Finally, abamectin, the active ingredient in DivaNem, developed and marketed by Syngenta is available and replaced the Avid 0.15EC formulation of abamectin which had previously been available under 24c labels in certain states. There are also several other formulations of abamectin available for turf in addition to DivaNem. All of these materials are much safer than the old pesticides used for nematode control. Experiences with these newer products has resulted in successes and disappointment since each has characteristics that limit their broad efficacy. For example, in a practical matter, none of these products has provided consistent good control of lance nematodes. However, generally Indemnify has been very good for sting and root-knot nematodes. Abamectin tends to bind very tightly to soil organic matter, so it is difficult for the pesticide to provide nematode suppression beyond about an inch or so of the soil profile. However, since that is the site where root knot nematodes predominate, abamectin works well for root knot. These setbacks are not unexpected, but they do illustrate the continuing need for newer, safe and effective nematicides. As with any material for control, we continue to learn how best to use these regarding timing, placement, rates and effectiveness for particular nematodes. Strategies that develop over time will likely include more than a single product, as none of these materials seems to be highly effective against all of the 'Big 3' (Sting, Root-Knot and Lance) in the South.
REFERENCES
Anonymous, 1979. DBCP turf uses suspended by EPA. Golf Business (Golfdom). Dec 53(12):5.
Christie, J. R., Good, J. M., and Walter, G. C. 1954. Nematodes associated with injury to turf. Proc. Soil Sci. Soc. Florida 14: 167-169.
Crow, W.T., and Welch, J. K. 2004. Root reductions of St. Augustinegrass (Stenotaphrum secundatum) and hybrid bermudagrass (Cynodon dactylon x transvaalensis) induced by Trichodorus obtusus and Paratrichodorus minor. Nematropica 34:31–37.
Dickerson, O., Blake, J. H., and Lewis, S. A. 2000. Nematode guidelines for South Carolina. Clemson S.C., USA; Clemson Cooperative Extension.
Peacock, Charles H. 1989. Turf nematodes: dealing with an old problem. Conf. Proceedings: 60th International Golf Course Conference and Show, p. 73.
Perry, V.G., and Horn, G.C. 1969. The nematode parasites of warm season turfgrasses. Proc. Int. Turfgrass Res. Conf. 1: 330–336.
Rhoades, H.L. 1962. Effects of sting and stubby root nematodes on St. Augustinegrass. Plant Disease Reporter 46:424–427.
Settle, D. M.; Fry, J. D.; Milliken, G. A.; Tisserat, N. A.; Todd, T. C. 2007. Quantifying the effects of lance nematode parasitism in creeping bentgrass. Plant Disease. September. 91(9): p. 1170–1179.
Shaver, Bradly R., Martin, S. Bruce, Bridges, W.C., and Agudelo, Paula. 2015. Effects of Trichodorus obtusus on zoysiagrass and bermudagrass root weight and turf quality. Nematology 17:671–678.
Ye, Weimin, Zeng, Yongsan, and Kerns, James. 2015. Molecular characterization and diagnosis of root-knot nematodes (Meloidogyne spp.) from turfgrasses in North Carolina, USA. PloS ONE Nov. 24, 10 (11): p.e0143556 (1–16).
Zeng, Y., Ye, Weimin, Martin, S. Bruce, Martin, Matt, and Tredway, Lane. 2012. Diversity and occurrence of plant-parasitic nematodes associated with golf course turfgrasses in North and South Carolina, USA. Journal of Nematology 44:337–347.