
8 minute read
Spikes of Death
Can Turfgrass Cultivation Effectively Control White Grubs?
By Ben McGraw, Ph.D. and Kevin Trotta
Advertisement
White grubs, or scarab beetle larvae, may be the one common pest that plague all turfgrass systems around the globe. Larvae feed directly on roots, which impedes water and nutrient uptake. If left unchecked, grub feeding may cause a separation of root tissue from aboveground plant material (Figure 1). This is particularly problematic on athletic fields where large populations of actively feeding grubs can create footing and other safety issues. Additionally, the mere presence of the larvae can attract predators (e.g. skunks and raccoons), which in turn may cause even more severe damage.
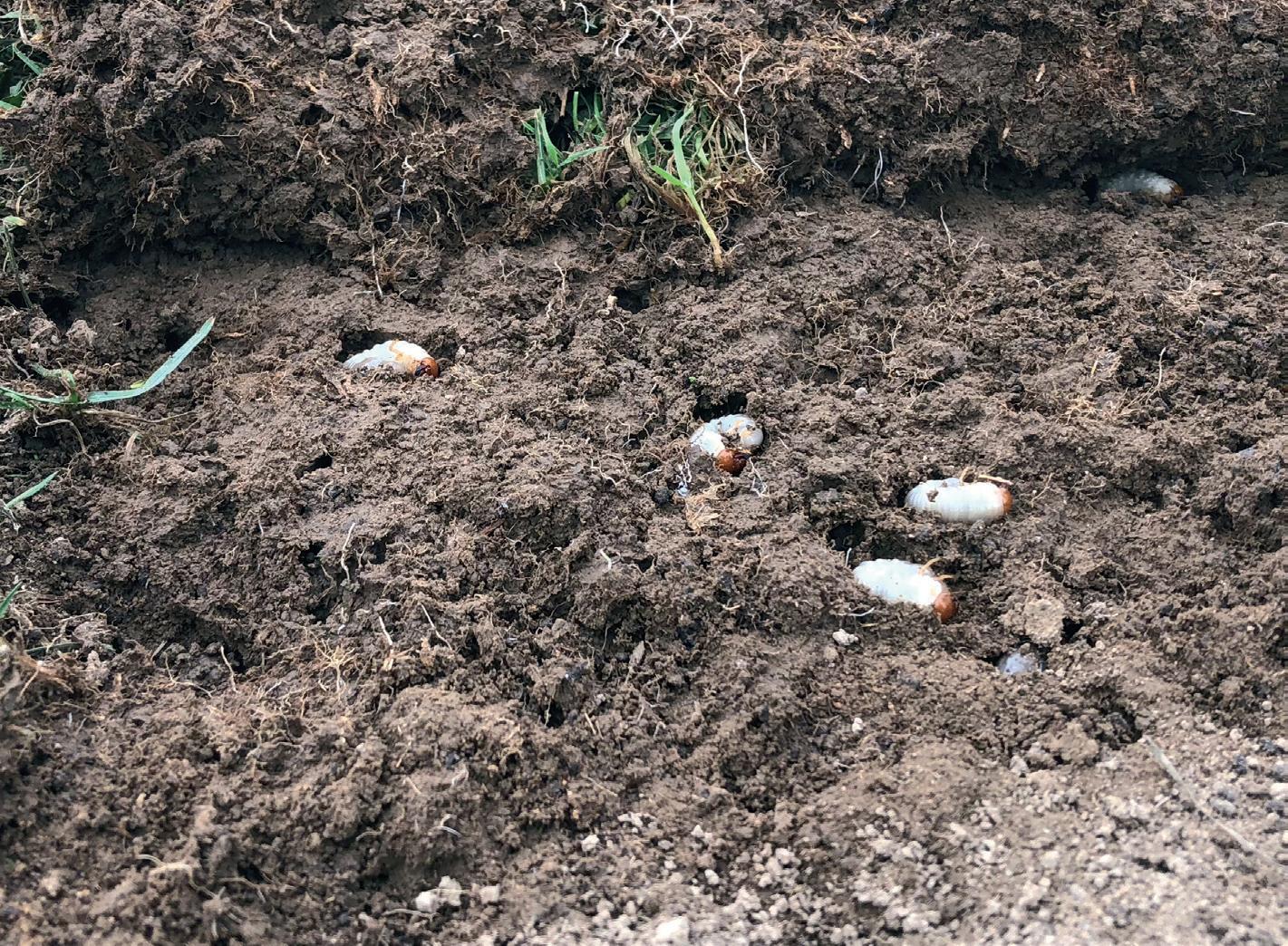
Figure 1: White grub larvae feeding near the soil surface
To avoid these issues, many turfgrass managers choose to control white grubs with preventive (prior to egg laying) or curative insecticides. However, over the last decade many municipalities, several states and Canadian provinces have limited, if not banned the use of synthetic insecticides for “cosmetic” purposes on public or school grounds. Given the increasing public demand for less-toxic alternatives to chemical controls and the tighter governmental scrutiny placed on pesticides used in turf, the Turfgrass Entomology Laboratory at Penn State has conducted studies over the last decade to determine whether turfgrass cultivation is a viable alternative to controlling white grubs with insecticides. Grubs are present near the soil for much of the year and likely to be affected by cultivation practices. Here we report findings from multiple field and simulation studies aimed at determining the expected levels of control provided by cultivation.
Equipment evaluation:
Preliminary field trials were initiated to determine which cultivation equipment/practices were most effective in controlling white grubs. Treatments consisted of plots cultivated with a hollow-tine aerifier (Toro ProCore ® 648; 0.5" tine, 2" forward spacing; Figure 2a), a solid-tine, vibratory cultivator (First Products UA-60 Aera-vator ® ; Figure 2b), and a below-ground injection system (Cambridge ® Liquid/Air Injection System; Figure 2c). Plots were roughmown (> 1.5 inches) Kentucky bluegrass/perennial ryegrass/fine fescue mixes and arranged in a randomized complete block design. Hollow- and solid, vibratory-tine treatments were applied as either one or two passes over the replicate plot. In treatments with two passes, the second pass was conducted perpendicular to the first pass, immediately following the initial application. Cambridge injection treatments consisted of six injections per plot of either pressurized air (125–150 PSI) or air plus a solid material (Turface ® ). Each treatment was replicated eight times. Grub control was assessed by removing eight golf course cup cutter samples from plots and manually examining the soil for larvae. Control was determined by comparing grub densities in treatments with those in the untreated controls.
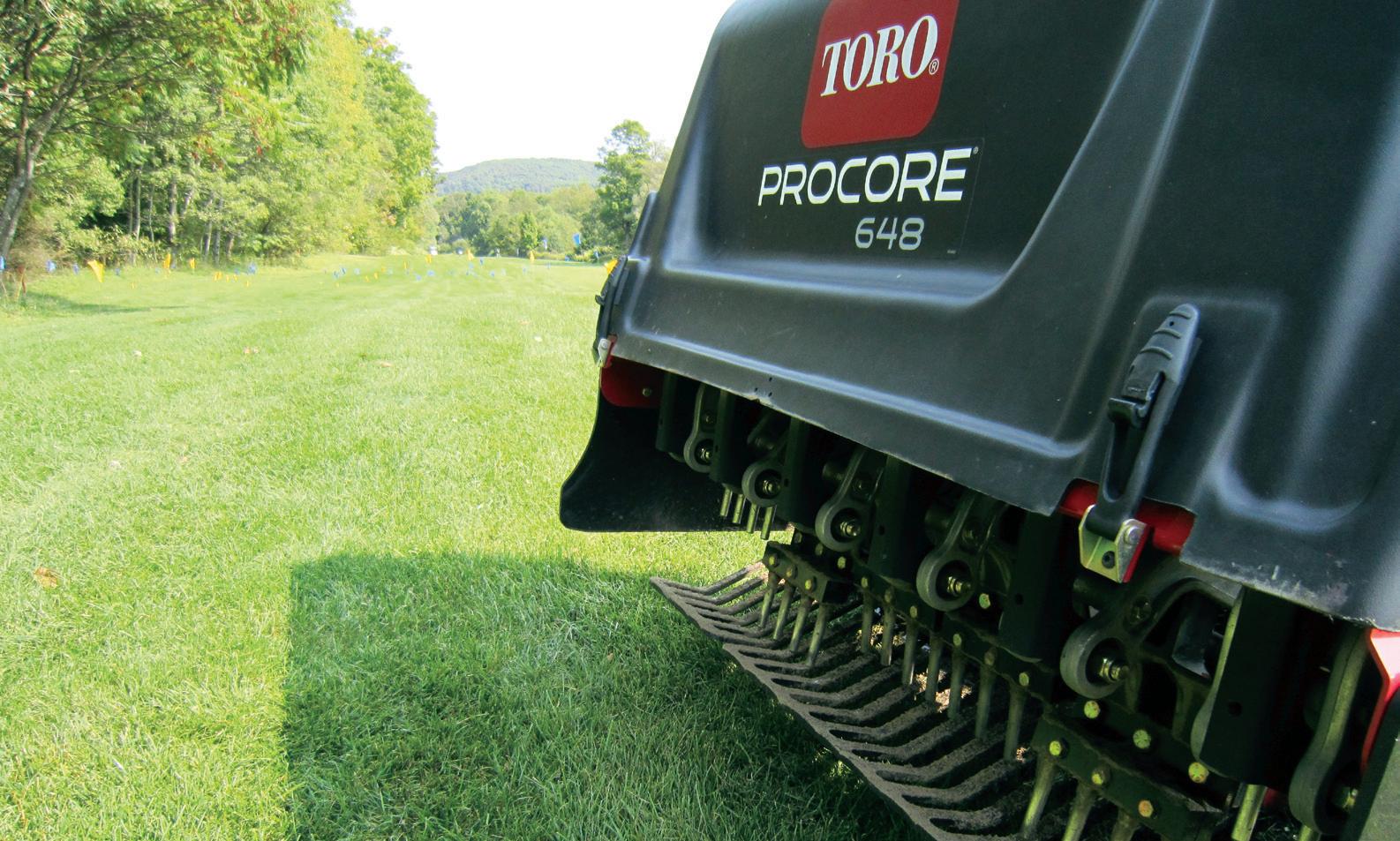
Figure 2a: Hollow-tine aerifier (Toro ProCore 648)
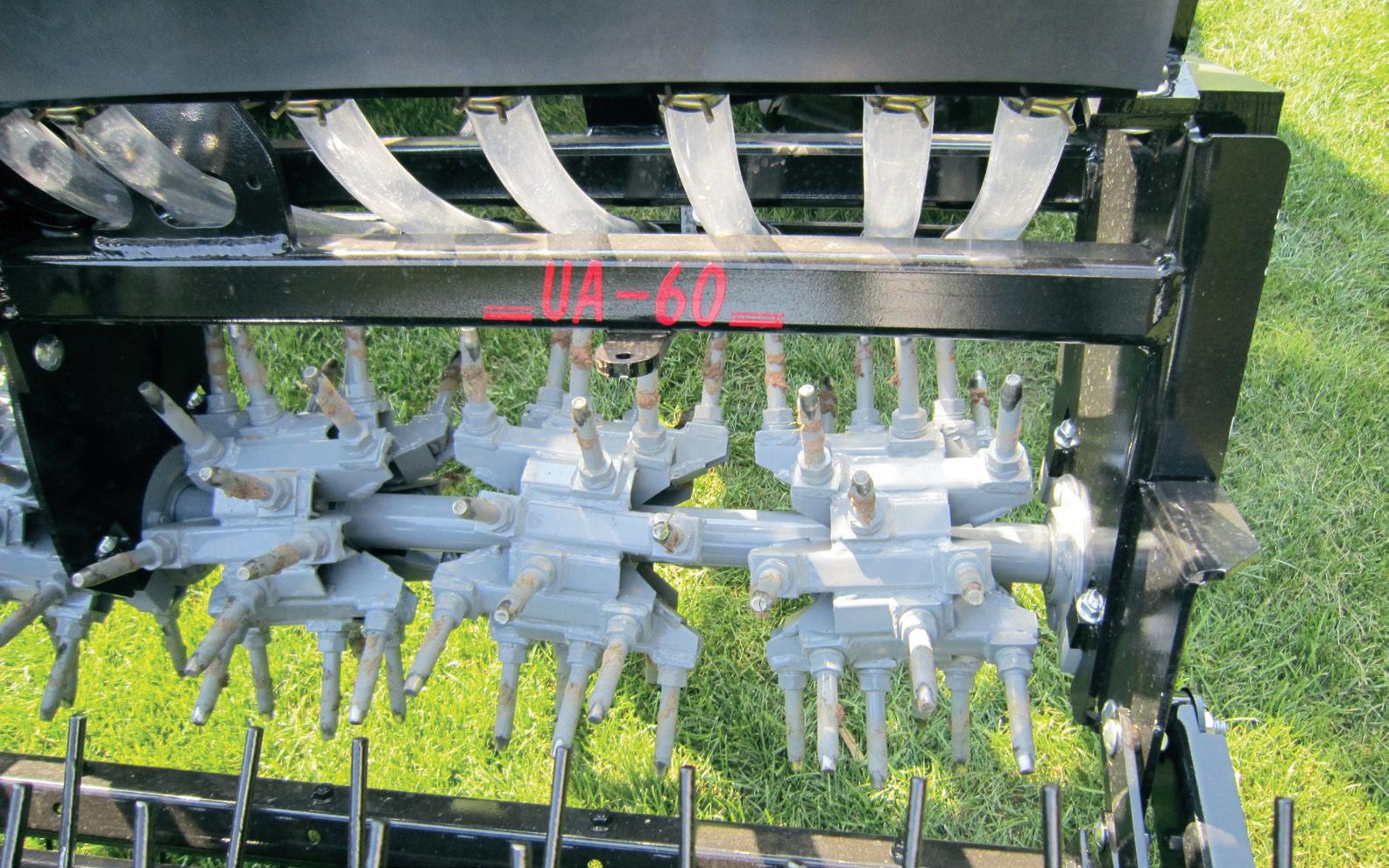
Figure 2B: Solid/vibratory-tine aerifier (First Products UA 60)
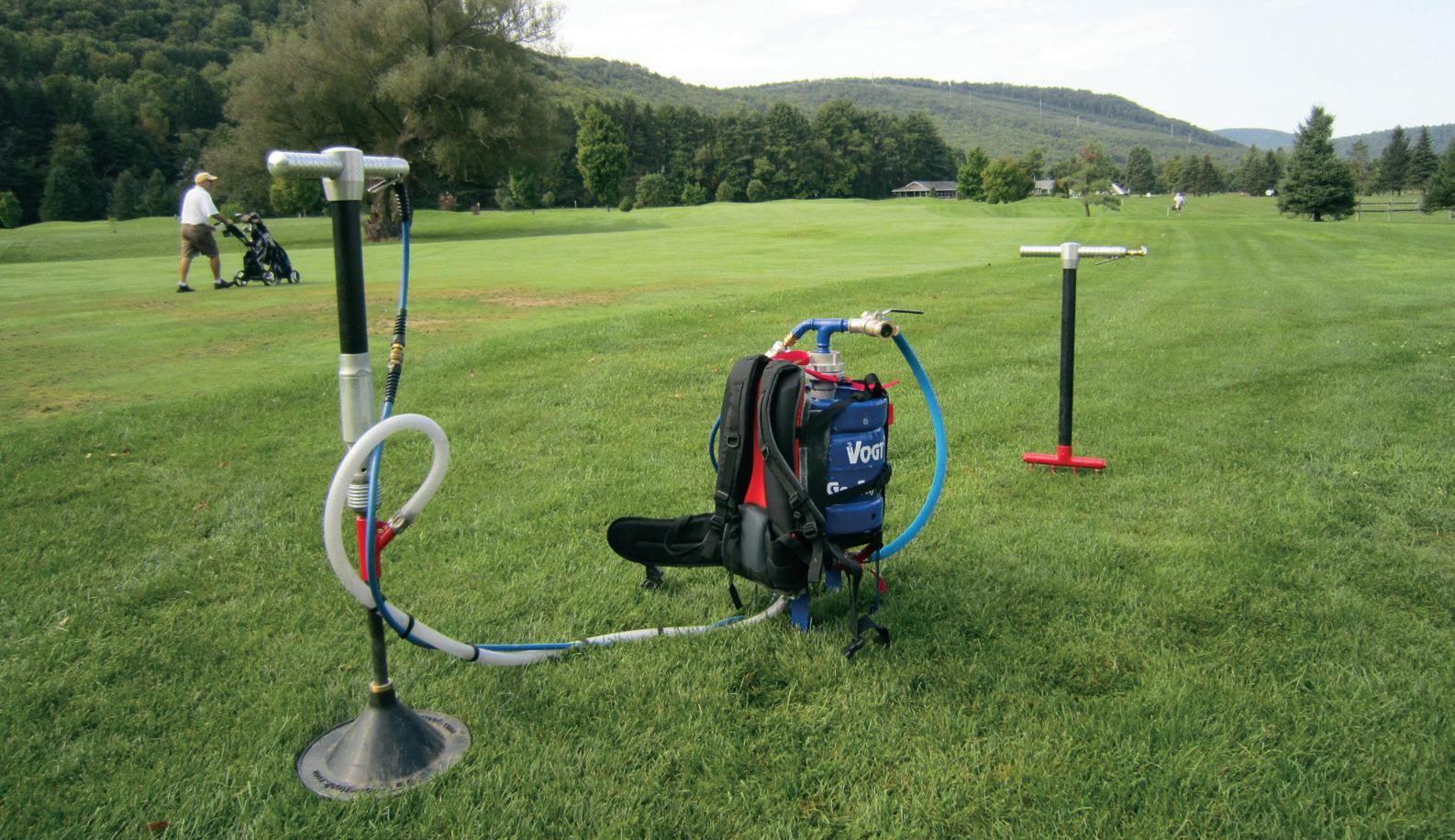
Figure 2C: Cambridge ® Liquid/Air Injection System
All treatments caused grub mortality (as determined by visual examination following treatment prior to the trial), yet control in the trial ranged between 0 to 30% with the greatest reductions observed in hollow-tine treatments with two passes. Multiple passes with both hollow- and solid, vibratory-tine aerators resulted in slightly greater, but nonsignificant reductions compared to one pass. Both Aera-vator and injection treatments were incapable of reducing white grub densities compared to the untreated checks. Therefore, future studies were conducted solely with the Toro ProCore (solid and hollow tines).
Cultivator variables
Tine diameter, spacing, and type
The effects of tine diameter, forward spacing of tines, and tine type on white grub control was examined over a twoyear period (2009–2010). In 2009, 10 treatments, consisting of two tine types (solid, hollow), two tine diameters (3/8", 1/2"), three forward tine spacings (1.5, 2, and 3"), and their interactions were examined. In 2010, 13 treatments were examined to include larger diameter tines (1/2" solid tines, and 1/2, 5/8 and 3/4" hollow tines) in an effort to improve control.
Grub reductions in aerified plots ranged from 6 to 53% in 2009, with the greatest reductions observed in the largest diameter tine treatment (53% with a 1/2" tine). When all tine spacing treatments were pooled by tine diameter, the larger tines resulted in the greatest reductions (21 – 53%). Though control was higher with the closer forward spacing of tines (28% – 1.5", 30% – 2", and 13% – 3"), no significant differences were found between treatments. Additionally, no significant interactions were found between tine spacing and diameter, despite the numerical trends for improved control with larger diameter tines and closer forward spacing. In the following trial, reductions ranged between 0 and 43%, though given the low grub densities in controls (2.2 larvae per ft 2 ) statistical differences were not found. The combined 3/4" tine treatments resulted in the greatest reductions (19%), followed by 5/8" (6%) and 1/2" (0%). Similarly, tine spacing trended towards greater control with more surface area disruption (0% and 8% reduction, for pooled 3" and 1.5" forward spacings respectively). No significant differences were detected between hollow- and solid-tine treatments in any of the trials.
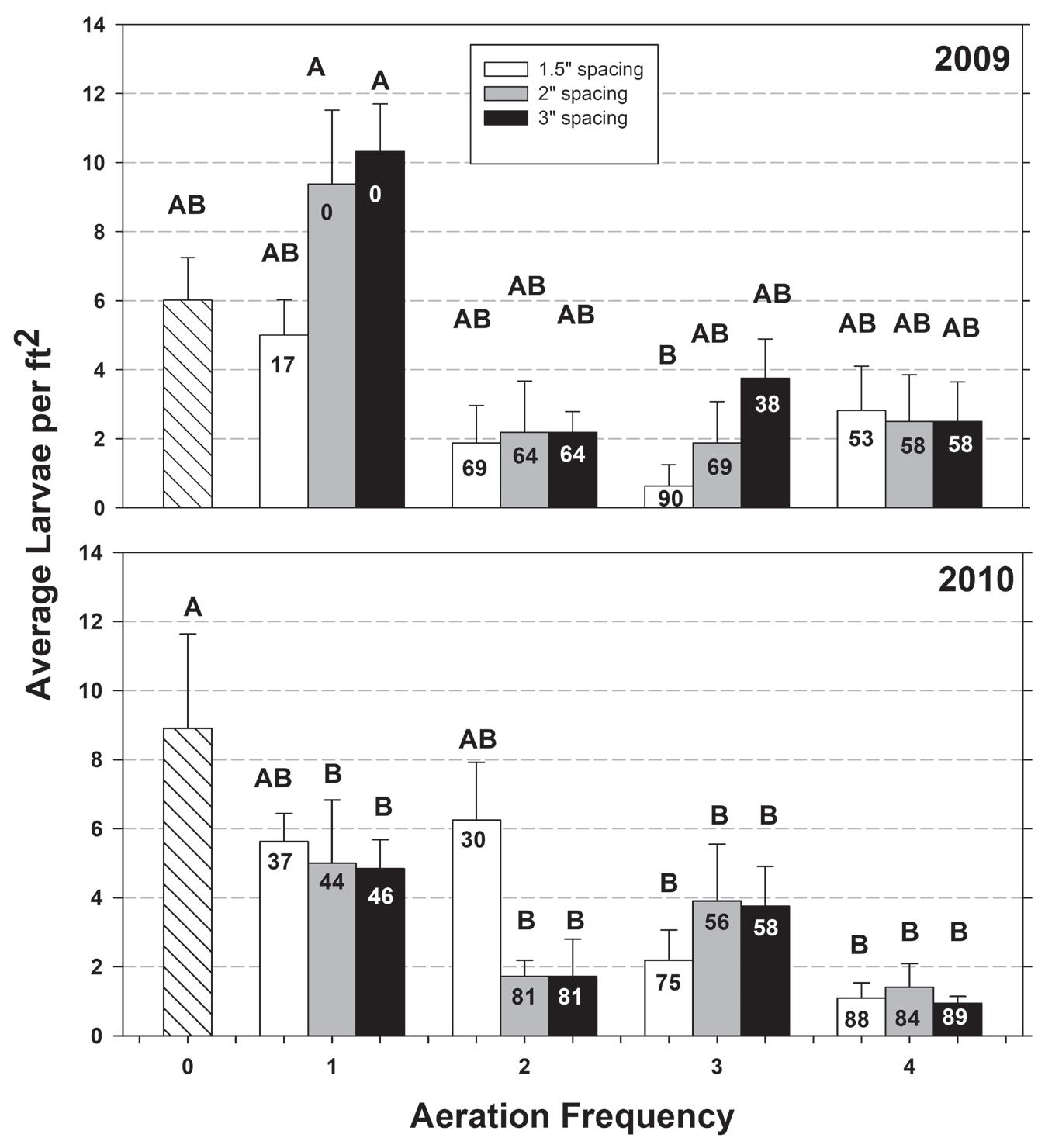
Figure 3: Effect of multiple passes with hollow-tine (0.5") aerifier on averagewhite grub density (± SE). Numbers inside bars represent percent controlrelative to the untreated checks. Treatments with same letters overbars are not significantly different from one another
Effects of aerification frequency
Fourteen treatments were examined, consisting of three forward tine spacings (1.5, 2, and 3") at four frequencies (1, 2, 3, or 4 passes) using a 1/2" diameter hollow tine. Significant reductions were observed in both years of the study in plots that were aerified more than once (Figure 3). Low mortality was observed in 2009 trials with only one pass in (0 – 17%), yet eight of nine multiple pass treatments provided between 53 and 90% control. Two (pooled average = 65%), three (65%), or four passes (56%) significantly improved control compared to one pass and the untreated check. Spacing did not have a statistically significant effect on grub reductions, though numerical reductions were greatest with closer forward tine spacing (1.5" = 57% control, 2" = 34%, 3" = 22%).
In 2010, similar results were observed in grub reductions with two or more passes. Moderate, yet more consistent reductions were observed with one pass (37 – 46% control) when compared to the same treatments in the 2009 trial. Four passes over the same plot significantly reduced grub densities (87% control, all forward tine spacing combined) when compared to one pass and the untreated control. However, four was not significantly different from two or three passes (64 and 63%, respectively). Over the two-year observation period, reductions ranged between 0 – 46% for one pass, 64 – 81% for two passes, 56 – 90% for three passes and 53 – 89% control for four passes.
••••••
Table 1: Results from in-field simulation modelling of white grub control with hollow-tine cultivation machinery (0.5" tine) with different forwardspacing patterns
••••••
Tine Spacing – in (cm): 1.5" × 2" (3.81 × 5.08)
Surface Area Affected (%): 6.54 %
Holes ft -1: 48
Simulated Control (% ± SE) 1: 7.31 ± 1.38
Actual Control (% ± SE) 2: 18.51 ± 3.09
••••••
Tine Spacing – in (cm): 2" × 2" (5.08 × 5.08)
Surface Area Affected (%): 4.91 %
Holes ft -1: 36
Simulated Control (% ± SE) 1: 5.34 ± 1.27
Actual Control (% ± SE) 2: 13.53 ± 2.99
••••••
Tine Spacing – in (cm): 3" × 2" (7.62 × 5.08)
Surface Area Affected (%): 3.27 %
Holes ft -1: 24
Simulated Control (% ± SE) 1: 4.6 ± 1.22
Actual Control (% ± SE) 2: 11.66 ± 2.93
••••••
1 Control estimated in the 200 plots/simulations. 2 Control estimated in only the plots that contain grubs (79 of the 200 simulations).
••••••
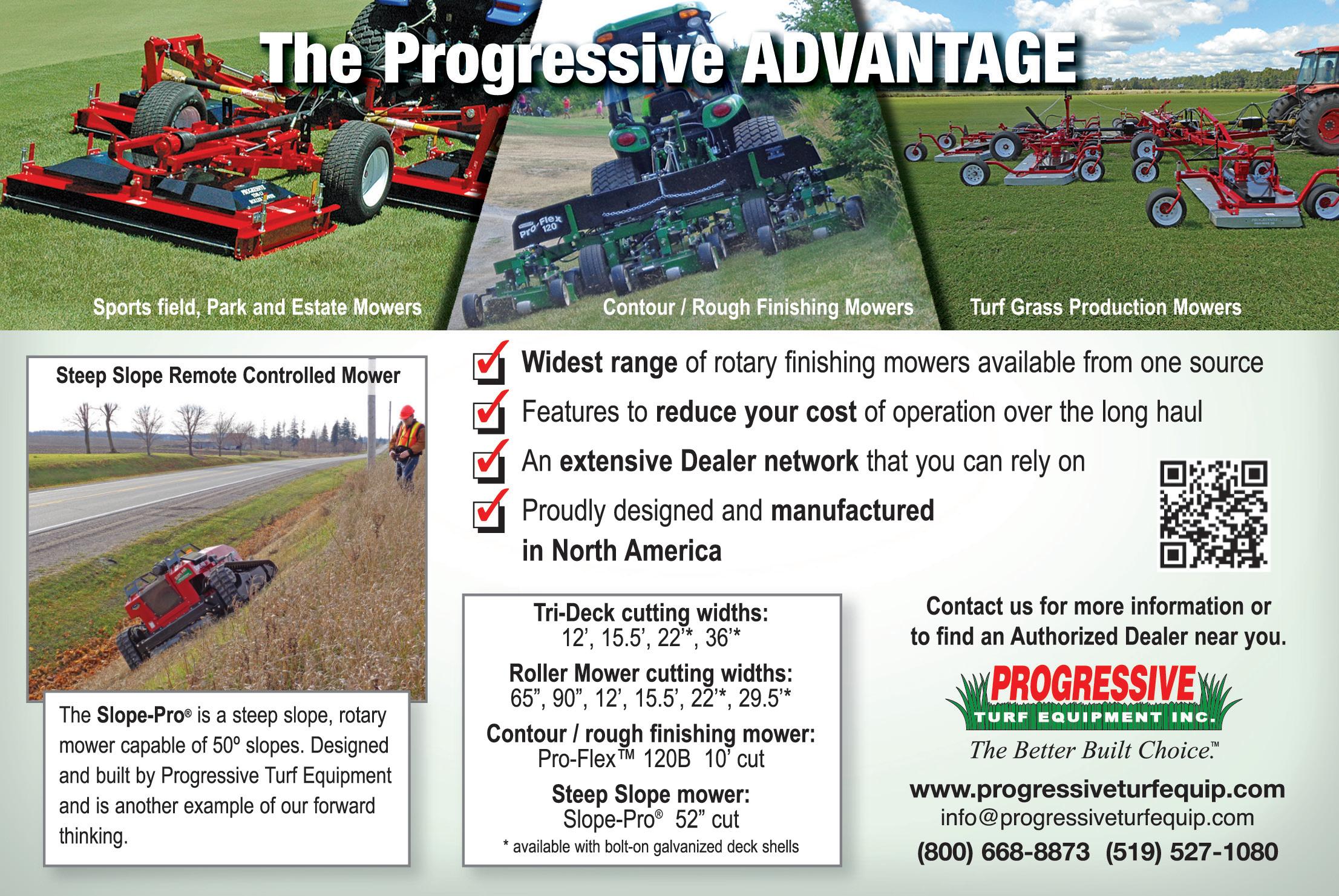
In-field modeling
We sought to model white grub mechanical control through in-field simulations of aerification to overcome variability associated with working with inherently patchy and low-density populations. Simulations were conducted on mixed Japanese beetle-northern masked chafer populations in roughmown turf. A 50 ft transect of turf was sod cut 1 – 2" deep and the turf was rolled back to expose grubs. Each run of the simulation involved placing one of three 1 ft 2 tine templates (1/2" tine at 2" lateral × 1.5, 2 and 3" forward spacings) over the ground. Grubs were recorded as killed if any part of the insect could be seen through the holes from above. After grub mortality was recorded, the template was exchanged, and the process repeated for each 1 ft 2 area in the transect. The experiment was repeated four times for a total of 200 simulations for each tine spacing pattern.
Simulated control was low when averaged across the 200 observations and closely mirrored the amount of surface area impacted by each pattern (4.6, 5.3, and 7.3% control for 3, 2 and 1.5" forward spacings) (Table 1). No significant differences were detected between the three tine patterns. A high percentage of plots did not contain grubs (60%). Removing these plots from the analysis revealed that actual control was three to four times greater (11.7, 13.5, and 18.5% control for 3, 2 and 1.5" forward spacing, respectively) which is closer to the estimated level of control observed in single aerification events in our field trials.
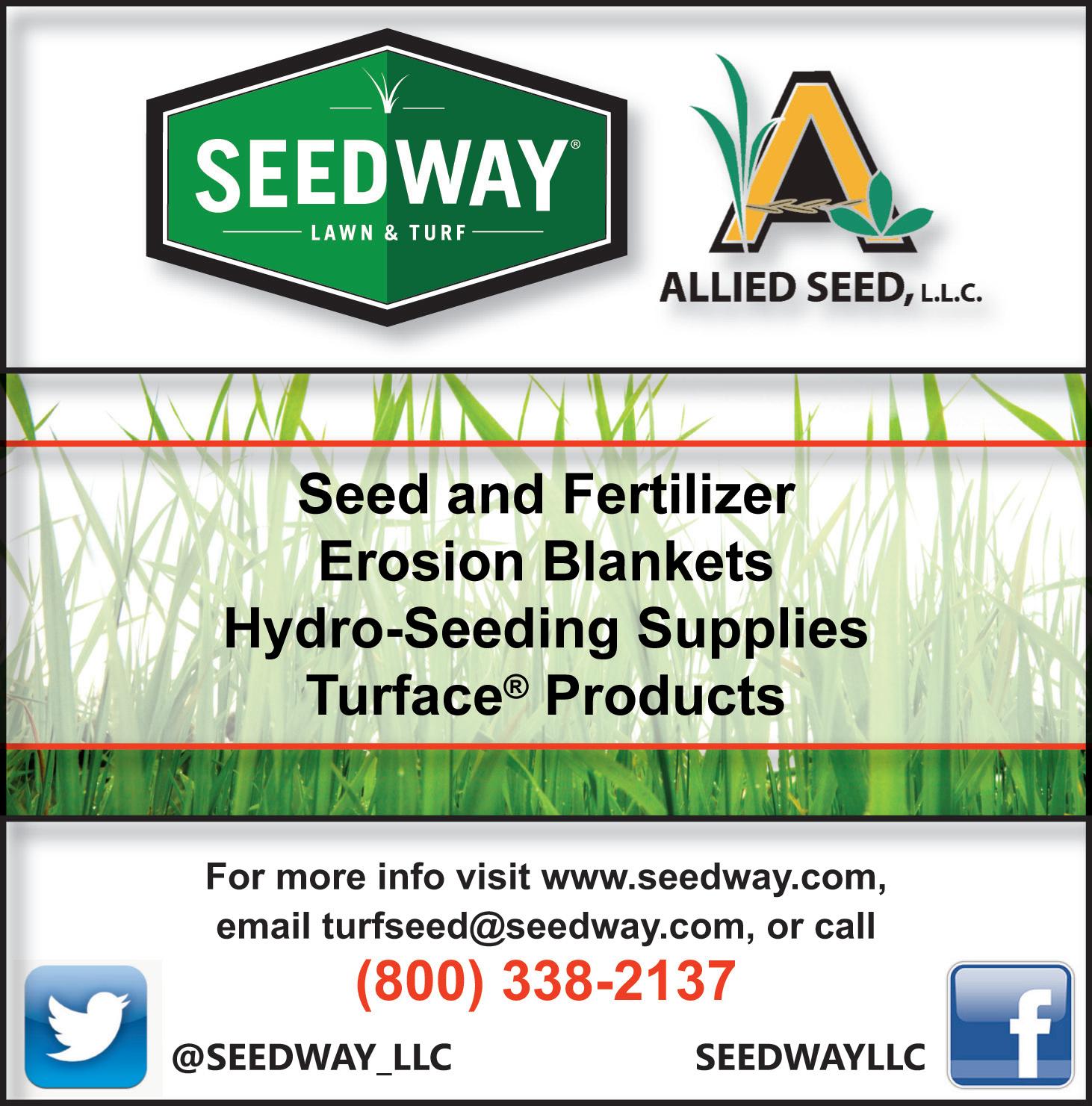
Conclusion
Mechanical controls do not have any of the inherent issues that chemical and biological controls have, including sensitivity to timing, grub species-specific susceptibility, and inconsistency with weather and site conditions. However, controls must be efficacious and act reliably to be a viable replacement for insecticides in an aesthetic cropping system like turfgrass. We were able to demonstrate that several cultivation techniques can cause white grub mortality. However, the impact was often minimal (and variable) with single events. Common sense would dictate that increasing the amount of surface disrupted (e.g. larger tines, closer spacing) would provide greater control. Aerification frequency was the most consistent factor in providing improved control in field trials, though these estimates were based on presence (no control)-absence (control) sampling. Simulated aerification trials demonstrated grubs are patchy or aggregated in natural populations which likely leads to the variability in control between trials and an overestimation of control in low-density populations. Population densities were below thresholds for damage (< 10 larvae/ft 2 ) and therefore more simulations are needed to determine not only the effect of multiple passes, but also increasing grub densities on observed control.
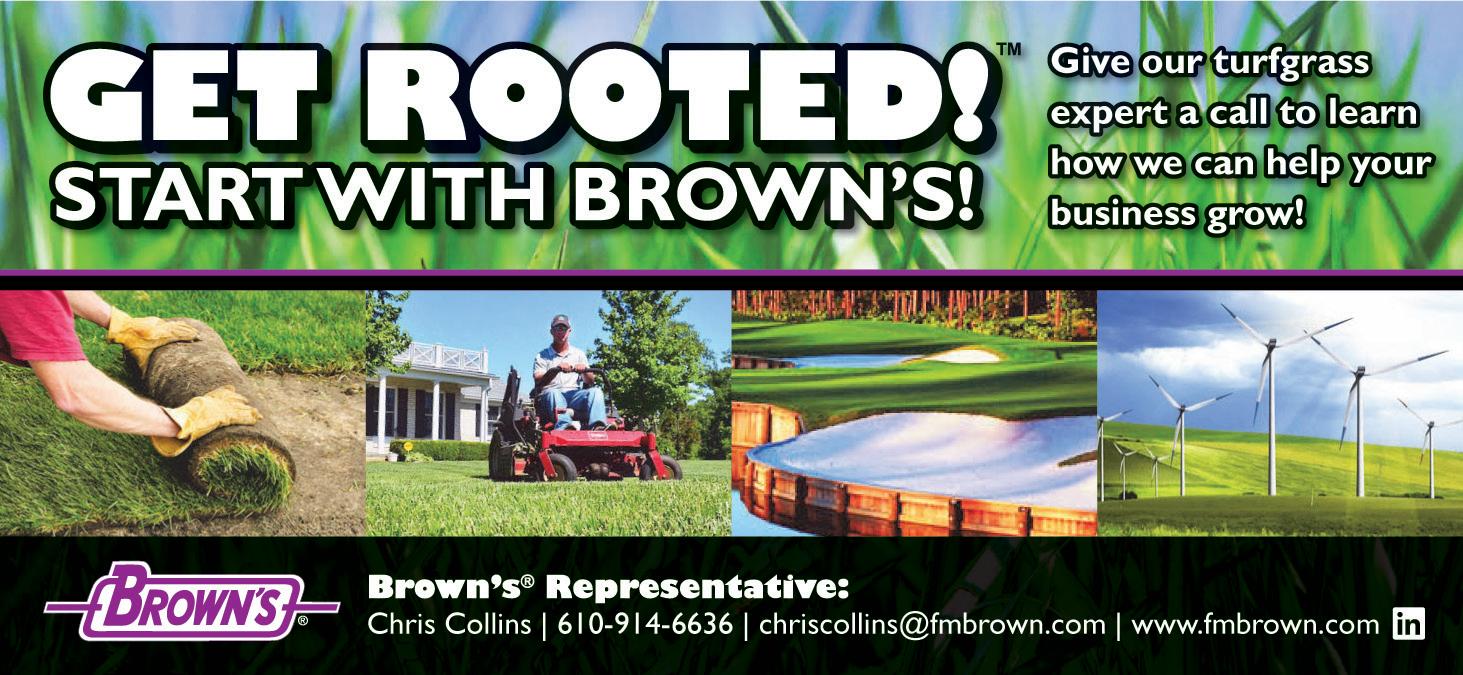
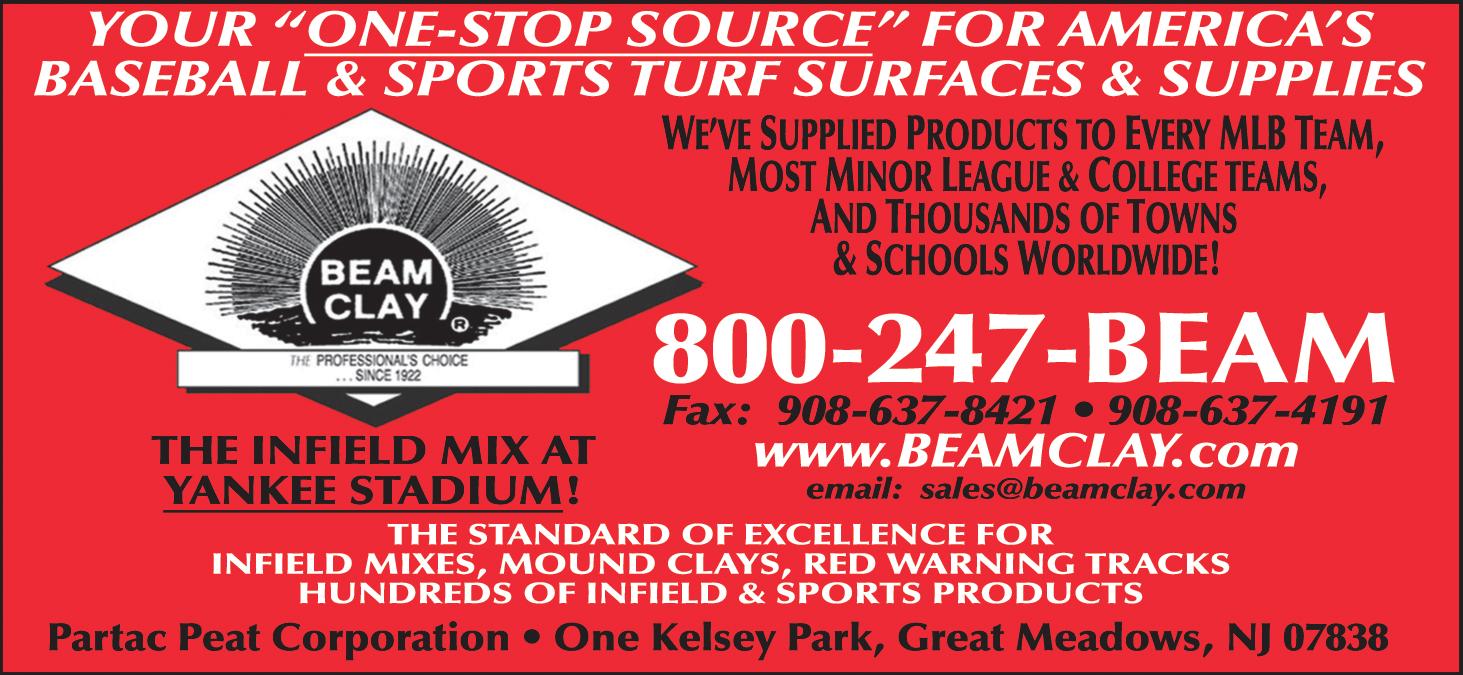