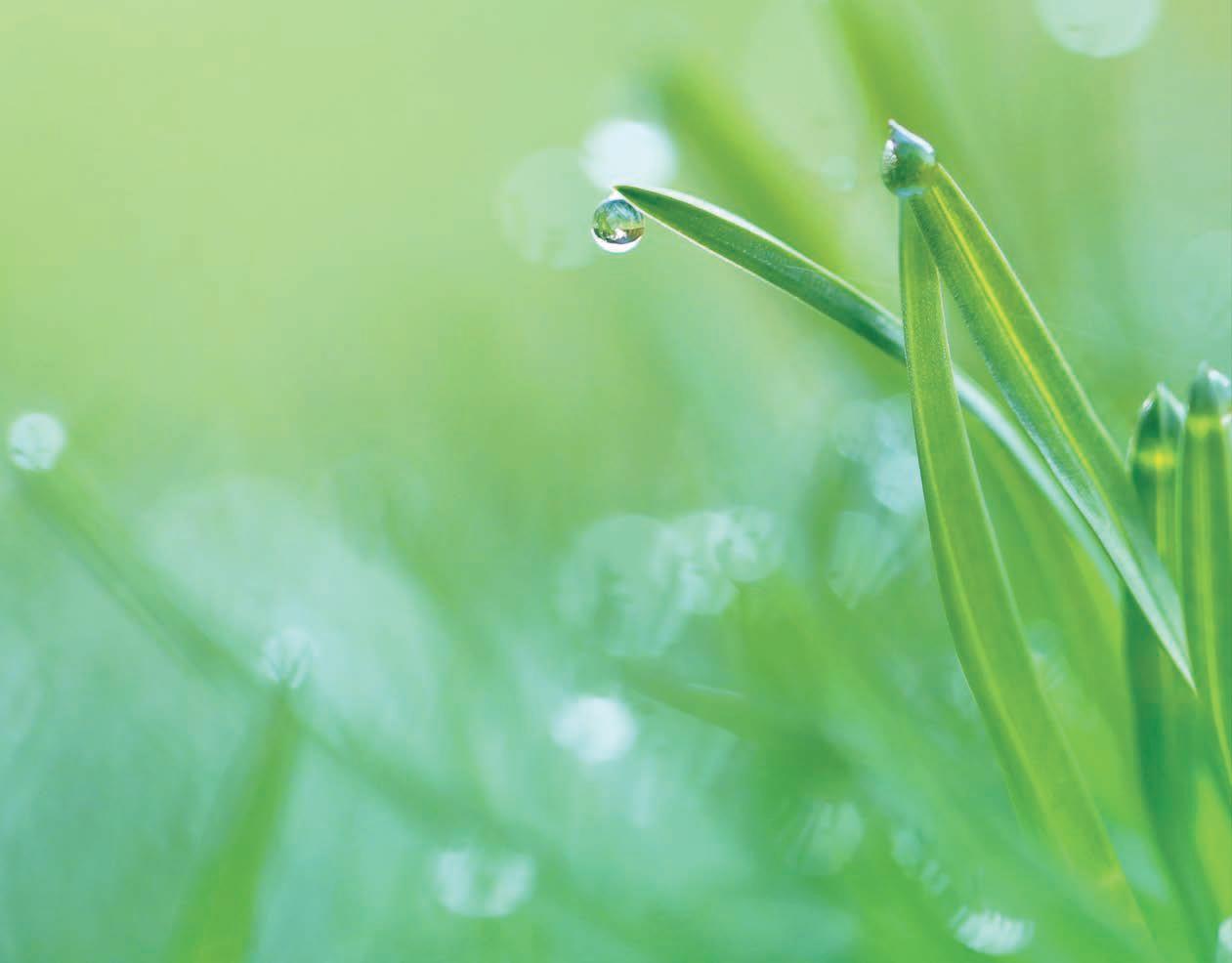
10 minute read
Feature
Coming Soon to Penn State University Park: Class ‘A’ Reclaimed H²O
By Max Schlossberg
Advertisement
Comprising 60 to 75% of green vegetation by mass, there is no more essential a component of plants than water. Accordingly, the quality and resilience of local ecosystems rely on its availability. Penn State’s leadership has long recognized the importance of water quality. Likewise, WE ARE wholly committed to local resource recovery and protection that supports base flow to Spring Creek and its delicate tributaries. A full summary of past and present initiatives supporting these objectives is available here: opp.psu.edu/sustainability/water.
Regarding effective wastewater treatment, the less impact on our ecosystems…the better. While the end of an engineered system’s functional life ideally yields replacement for some, Penn State’s Office of Physical Plant recently seized an opportunity to make an inestimably effective upgrade. This, combined with highly efficient bio-engineering and membrane filtration technology advancements, now comprises new infrastructure that will facilitate the recovery, treatment, and delivery of ‘Class A’ reclaimed water across the University Park campus!
What does this mean?
Those of us managing plant systems in support of Penn State’s mission should embrace and commit to effective resource adoption when afforded the opportunity. The extent to which we utilize reclaimed water protects valuable ground water resources! Doing so essentially ‘skips the middleman’ and avoids risk and expense incurred through land application of ‘Class C’ reclaimed water 365 days a year. Only ‘Class A’ reclaimed water is permitted for irrigation of unrestricted public areas and edible crop systems of the Commonwealth. Resultingly, the PA Dept. of Environmental Protection (DEP) mandates more intense water quality testing protocols for ‘Class A’ than all lesser reclaimed water designations. Furthermore, every gallon of safe and convenient Class A effluent used to foster plant growth, function, and health, precludes the energy-intense extraction and treatment of one-gallon potable water from the ground. It’s not unlike what Charlie Sheen calls winning, but in a wholesome way that the Lorax approves.
How is irrigating with reclaimed water different from potable water?
Good question. It is different in that it contains a greater number of soluble, or dissolved, solutes. The degree to which certain concentrations have changed in recent period varies by solute. Irrigation water quality parameters most influencing plant nutrition and physicochemical properties of receiving soils include total solute, alkalinity, sodium, and chloride concentration. While the primary goal of irrigation is supplementing natural precipitation to satisfy plant water requirement in support of optimal growth and yield, delivery of plant essential nutrients often comprises a benefit. Penn State’s reclaimed water at University Park contains dependable levels of Ca, K, Mg, NH4, NO3, P, and S; all essential macronutrients in plant available forms (Table 1). Furthermore, the reclaimed water contains trace to measurable concentrations of the following plant essential micronutrients: B, Cl, Cu, Fe, Mn, Mo, and Zn. Nutrient and liming agent delivery loads are shown in lbs per foot•acre (325,851 US gallons) irrigation applied.
Salt affected soil and climatic conditions exacerbating risk
Salt affected soils continue to form and persist worldwide. The most common salt affected soil conditions are sodicity and salinization. Either can be caused by sodium, but only sodicity is for certain. Potassium, bi/carbonate, sulfate, and/or chloride solutes can be as significant an influence on soil salinization as sodium. Drought and/or saltwater intrusion are common causes of salt affected soil, yet irrigation with low quality water also contributes. Particularly in highly populated and arid, semi-arid, or Mediterranean climatic regions, where irrigation water constitutes the sole provision for meeting crop water requirements. The extent to which growers rely on irrigation water to satisfy crop/plant needs is a heavy, if not overriding, influence on the risk of a soil becoming salt affected.
Figure 1 shows the 30-year average monthly precipitation totals (blue columns) in University Park, USA or Marrakech, Morocco. Clearly, University Park receives more total precipitation annually than Marrakech, and that precipitation is distributed more evenly throughout the year. The other data displayed in each half of Figure 1 is the estimated 30-year average monthly evapotranspiration (ET) rate (black line). The magnitude and monthly distribution of soil water extraction, or reference ET rate, is influenced by mean air temperature, atmospheric vapor pressure, solar radiation intensity, photoperiod length, and windspeed. The two locations have a similar annual distribution of reference ET rate. Due to its higher elevation and arid climate however, soil water in the Marrakech landscape experiences greater atmospheric tension, i.e., extraction, than its University Park counterpart April to September.
For any given month, the reference ET rate affords us an estimate of a plant’s average maximum water requirement in head units. The disparity between that line and the blue column, in any month, indicates a plant water surplus or deficit (requirement) in head units. To optimize plant growth and yield, irrigation is needed to rectify water deficit conditions. It is important to realize that the values shown for both the blue columns and the black line are 30-year averages. While the University Park panel in Figure 1 indicates an irrigation requirement over the summer months, Central PA residents undoubtedly recall the extent to which this may vary by year! For example, a 7" water deficit accrued from June to July in 2016, whereas optimal plant growth over the entire summer of 2018 required a mere 2" of supplemental irrigation (Fig. 2).
Soil salinization prevention
Returning briefly to the Marrakech, Morocco water balance diagram (Fig. 1), most salt affected soil degradation worldwide results from growers rectifying water deficits using limited quantities of low-quality irrigation water. Salts applied in irrigation water, if not diluted and mobilized downward by natural precipitation, will accumulate to problematic concentrations at the soil surface. As fortunate benefactors of dependably frequent precipitation, open-air facilities in University Park are unlikely to be affected by salts. Yet under high tunnels or glass, plant-available water is supplied exclusively by irrigation. As a result, the associated soil or potting media is at marginal risk of becoming salt affected by solutes in reclaimed water.
Fortunately, most plants are tolerant of the solute concentration observed of Penn State reclaimed water as defined by its mean electrical conductivity (ECw = 1.5 dS /m). For salt-sensitive plant species, agronomists have refined methods for preventing soil salinity from developing. The common approach is counterintuitive, yet effective, so just trust me . For technicians and managers of greenhouse and high-tunnel systems, the continued health of salt- sensitive plants is sustained by employing the maintenance leaching requirement (MLR). Essentially, this means over-irrigate!
The same advice pertains to managers of open-air facilities experiencing drought conditions (>2" plant water deficits, June through Sept). Presuming moderate to high soil permeability, the MLR strategy pushes salt out the bottom of the root zone to prevent accumulation. Table 2 shows the % MLR requirement and irrigation multiplier based on the maximum observed electrical conductivity of Penn State reclaimed water (ECw =1.74 dS /m) and ECe (electrical conductivity of soil extract) thresholds for very sensitive (0.75 dS /m) to moderately tolerant (5 dS /m) plant species. ECe thresholds for grasses, ornamentals, and trees common to mid-Atlantic landscapes are maintained by the USDA (plants. usda.gov) and Food & Agriculture Organization (fao.org/3/ y4263e/y4263e0e.htm).
The MLR strategy effectively fosters optimal production of even the most salt-sensitive species. However, there are two important governing requirements:
1. Moderately- to highly-permeable root zones free of impeding layer(s), and
2. Reliable and uniform irrigation delivery.
Most Penn State facility managers have already recognized and attained these goals. Otherwise, greenhouse and high-tunnel systems can achieve Req. 1 through installation/maintenance of subsurface drainage and use of coarse-textured potting media (avoid clayey mineral soils) or soil amendments (sand, compost, expanded/ calcined clay), respectively. Agronomic and horticultural managers of rainfed landscapes or production systems can facilitate soil structural percolation (permeability) through organic amendment and mulching. Similarly, turfgrass managers should take opportunities to incorporate compost into seedbeds being prepared for establishment or after physically cultivating (coring, dethatching, etc.) mature turfgrass systems. Req. 2 is achieved by assessing/ auditing overhead irrigation coverage, adjusting pressure/nozzles for uniformity, and scheduling intermittent (pulse) application of overhead irrigation in the early morning hours (2 to 6 am). Increasing drip irrigation flow rate may necessitate replacement and/or more frequent inspection and maintenance of line/tape by landscape, nursery, and high tunnel growers/managers.
Soil sodicity prevention
While the only kind of salt-affected soil more difficult to reclaim than a sodic soil is a saline-sodic soil, development of either requires specifically unfortuitous circumstances. These include, among others; clayey soil, an arid climate, and dependence on irrigation water having high sodium and bi/carbonate relative to calcium and magnesium levels. While the mean sodium adsorption ratio (SARw= 3.25) of Penn State’s reclaimed water is elevated relative to previous potable water sources, it falls well short of the moderate sodium permeability hazard (SARw= 10) threshold. In layman’s terms, the ratio of sodium to both calcium and magnesium in Penn State reclaimed water comprises a non-issue. Likewise, the negative mean residual sodium carbonate (RSC= –3.1) mitigates sodium activity. Thus, naturally occurring precipitation and/or adherence to the MLR by managers irrigating with the reclaimed water will reliably protect the soil or potting media cation exchange suite from sodium adsorption, a.k.a. sodicity development.
Assessment of salt affected soil or potting media
The best way to assess what you suspect may be salt-affected soil or potting media, is to collect a representative soil sample and submit it for saturated paste extract (SPE) analysis. If this sounds more specialized than a routine soil test, it should…because it is. A partial list of Soil Laboratories offering SPE analysis includes:
• Logan Labs LLC (loganlabs.com) 620 North Main St. Lakeview, OH 43331
• UC Davis Analytical Lab (anlab.ucdavis.edu) Hoagland Annex One Shields Ave. Davis, CA 95616-5270
• Waypoint Analytical (waypointanalytical.com) 2790 Whitten Rd. Memphis, TN 38133
In the meantime, sample the soil to the depth plant roots are capable of exploring. For turfgrass, this is typically a 0 to 4" soil depth. For herbaceous/woody ornamental, native grassland, pasture, or horticultural production systems, an appropriate sampling depth is 0 to 6". For understory or forested landscape systems, a 0 to 8" or 6 to 12" depth should be sampled. An important aspect of soil sampling is obtaining a composite sample representative of the soil depth. There are countless tools available to sample soil, but resilience and repeatability make punch-type soil probes very popular. Using a spade to sample soil typically over-represents the surface two or three inches. The Penn State Cooperative Extension Service features video tutorials on this topic (https://extension.psu.edu/soil-sampling).
Summary
Once available, Penn State’s reclaimed water will support resource conservation, environmental quality, and pursuit of the University’s mission. This ‘Class A’ reclaimed water contains safe, yet supportive, levels of essential minerals and plant nutrients. These and other intensely monitored constituents will supplement liming agent and fertilizer requirements of maintained turfgrass, herbaceous, ornamental, pasture, horticultural, agronomic, and forested systems across our campus facilities and landscape. Relative to recent campus potable water, experienced managers will not detect any differences in the appearance, utility, or presence of the reclaimed water. Only under severe drought conditions should managers of open-air, rainfed facilities invoke a maintenance leaching requirement (MLR) strategy. For managers of greenhouses or other irrigation-reliant production facilities, particularly those managing salt-sensitive plant species; employing an MLR facilitated by under-drained soil or potting media may prove a worthwhile new adoption.
REFERENCES
Carrow, R.N., and R.R. Duncan. 2012. Best Management Practices for Saline and Sodic Turfgrass Soils: Assessment and Reclamation. CRC Press Taylor & Francis Group, Boca Raton, FL.
Li, L., J. Young, and S. Deb. 2019. Effects of Cultivation Practices and Products on Bermudagrass Fairways in a Semiarid Region. Agron. J. 111:2899–2909 doi:10.2134/ agronj2019.04.0262.
Sanchez, E., T. Ford, and R. Berghage. 2019. Irrigation Water Quality for High Tunnels. Pennsylvania State University Cooperative Extension. https://extension. psu.edu/irrigation-water-quality-for-high-tunnels.