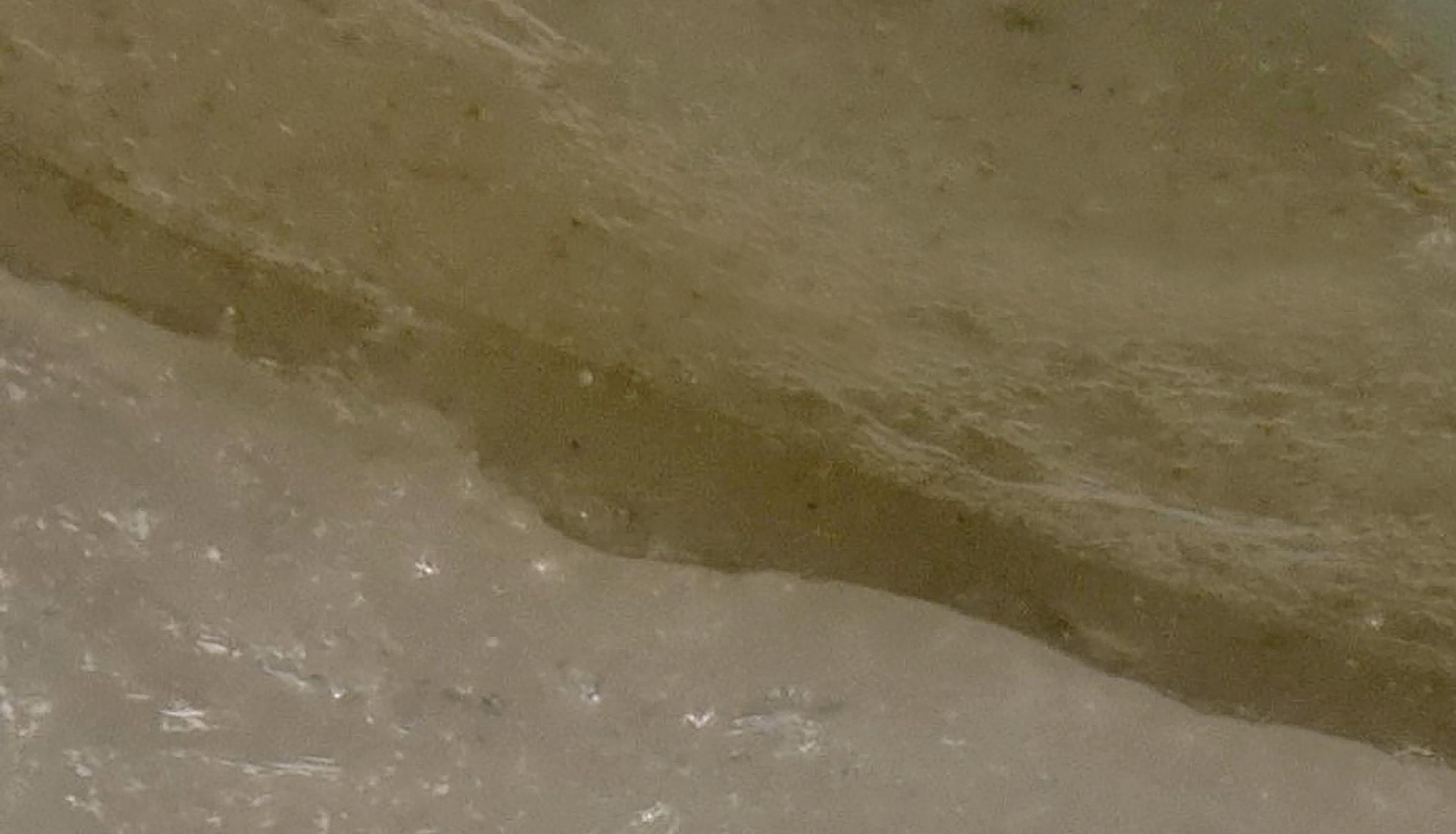
10 minute read
Feature
State of Matters: Fertilizer Coating Technology
By Max Schlossberg, Ph.D.
No more than zero artificial intelligence resources participated in the composition of the following chronicle.
In the beginning…
By the time NASA successfully landed their unmanned ‘Surveyor 1’ spacecraft on the moon (1965), numerous slow-release, granular N fertilizers were available in the US. Natural organic fertilizers derived from sewage sludge or livestock manure/litter were the original slow-release N carriers. Next came the urea-formaldehyde reaction products; e.g., methylene urea, isobutylidene diurea, and the eponymous ureaform, all characterized as synthetic organic carriers. However, neither these natural nor synthetic organic N sources completely satisfied the Green Industry’s need for a dependably long-lasting, controlled-release, N fertilizer.
But by the time Jacobsen released the original ‘Greens King’ triplex putting green mower (1968), agricultural and material scientists had developed coated fertilizers. Their purpose was to improve the utilization efficiency of nitrogen fertilizer, most notably urea (46-0-0). This effort improved the granular fertilizer at its core by adding one (or more) layer(s) of a persistent coating that limited nutrient diffusion from inside to outside the coating/membrane.
Alkyd resins, synthetic polymers derived by reacting polyhydric alcohols with polybasic acids or anhydrides, were the original fertilizer coatings. Yet the high cost of this added value precluded widescale adoption of the urea fertilizer featuring it. Subsequently, scientists of the Tennessee Valley Authority patented a more economical process of coating urea with elemental sulfur. In 1970, sulfur-coated urea (SCU) became the first, mass-produced, controlled-release fertilizer by which plant-availability of urea-N was initiated by physical rupture or ‘failure’ of the sulfur coating.
The prolific marriage of urea and sulfur was hardly a proverbial crapshoot, given sulfur’s already established role as a plant essential macronutrient, fungicide, and additive mitigating the caking tendency of many fertilizers. Moreover, elemental sulfur comprises a biodegradable and inexpensive acidulent that facilitates plant recovery of urea-N in neutral to alkaline soil. Relative to granular urea and confirming all coatings remained intact through application, numerous University studies showed SCU to significantly improve fertilizer N recovery by treated turfgrass.
This is likely why the next generation of coated fertilizer built upon, rather than replaced, the sulfur component. While the controlled release of urea N from SCU is governed by the sulfur-coat thickness, the addition of a thin thermoplastic or resin envelope around the SCU prill improved the coating integrity without further N dilution. These polymer-sulfur-coated urea (PSCU) or polymer-coated/ sulfur-coated urea (PC/SCU) hybrids featured a more linear release rate than SCU and were widely adopted in the late 1980’s.
Yet the 21st century ushered in a new era of reduced polyurethane/polyolefin cost and a specialty fertilizer marketplace heralding granules coated by a thin layer of polymer as the preferred technology (Figure 1). Owing to their thinner coat, synthetic polymer-coated fertilizers possess a higher grade than their sulfur coated counterparts. Polymer-coated urea (PCU) is minimally affected by microbial activity and remains a highly popular blending source within the turfgrass, landscaping, and horticultural production industries.
While polymers proved aptly supportive of the original, impermeable coating mechanism; newer synthetic polymers marked the advent of semi-permeable coatings. In contrast to the physical rupture or coating ‘failure’ release mechanism exhibited by firstand second-generation coated fertilizers, semi-permeable coatings facilitate a multi-stage diffusion release mechanism governed by an osmotic nutrient transfer from the fertilizer–polymer interface to the polymer–soil interface. Progression through the following sequence; (i) diffusion/swelling; (ii) degradation of the polymer coating, and (iii) fracture or dissolution, is governed by coating thickness, soil temperature, soil moisture, and soil N concentration gradient; all ideally falling in synchrony with vegetative growth and nutrient requirements.
Over the last twenty years…
I have had the honor of evaluating numerous slow- and controlled-release N fertilizers, at the very facility where the first experimentally-coated fertilizers were evaluated by Drs. Duich and Waddington almost sixty years ago. These recent results from the Joseph Valentine Turfgrass Research Center confirm improved plant recovery of urea-N to be a benefit of ongoing technological refinement by polymer-coated granular fertilizer manufacturers. While different granular fertilizers may share a common 100% polymer-coated urea (PCU) attribute and support equivalent cumulative recovery by Kentucky bluegrass or similar turfgrass species, significantly varied patterns of N release have been shown to manifest over 17-week experimental periods (Figure 2).
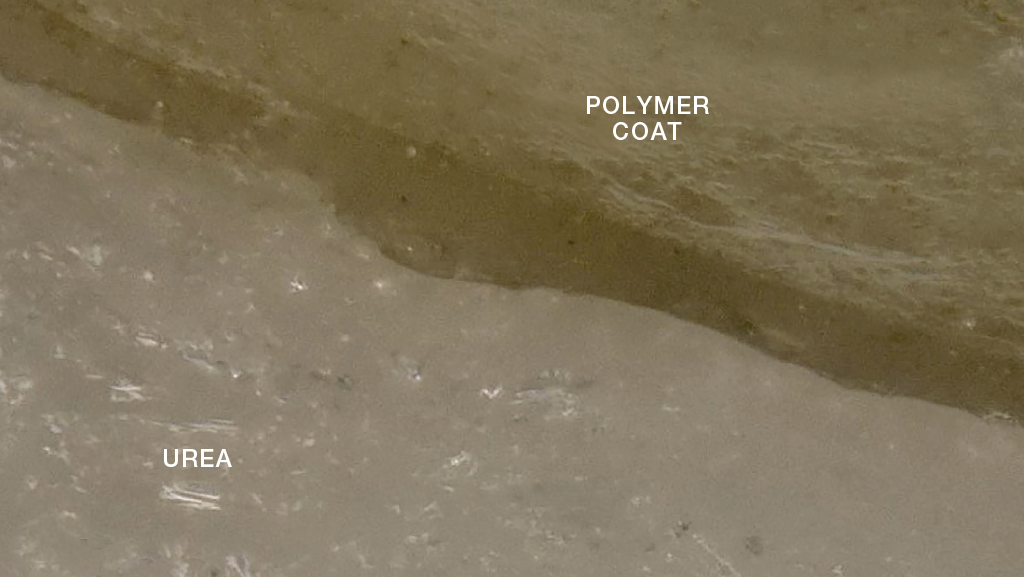
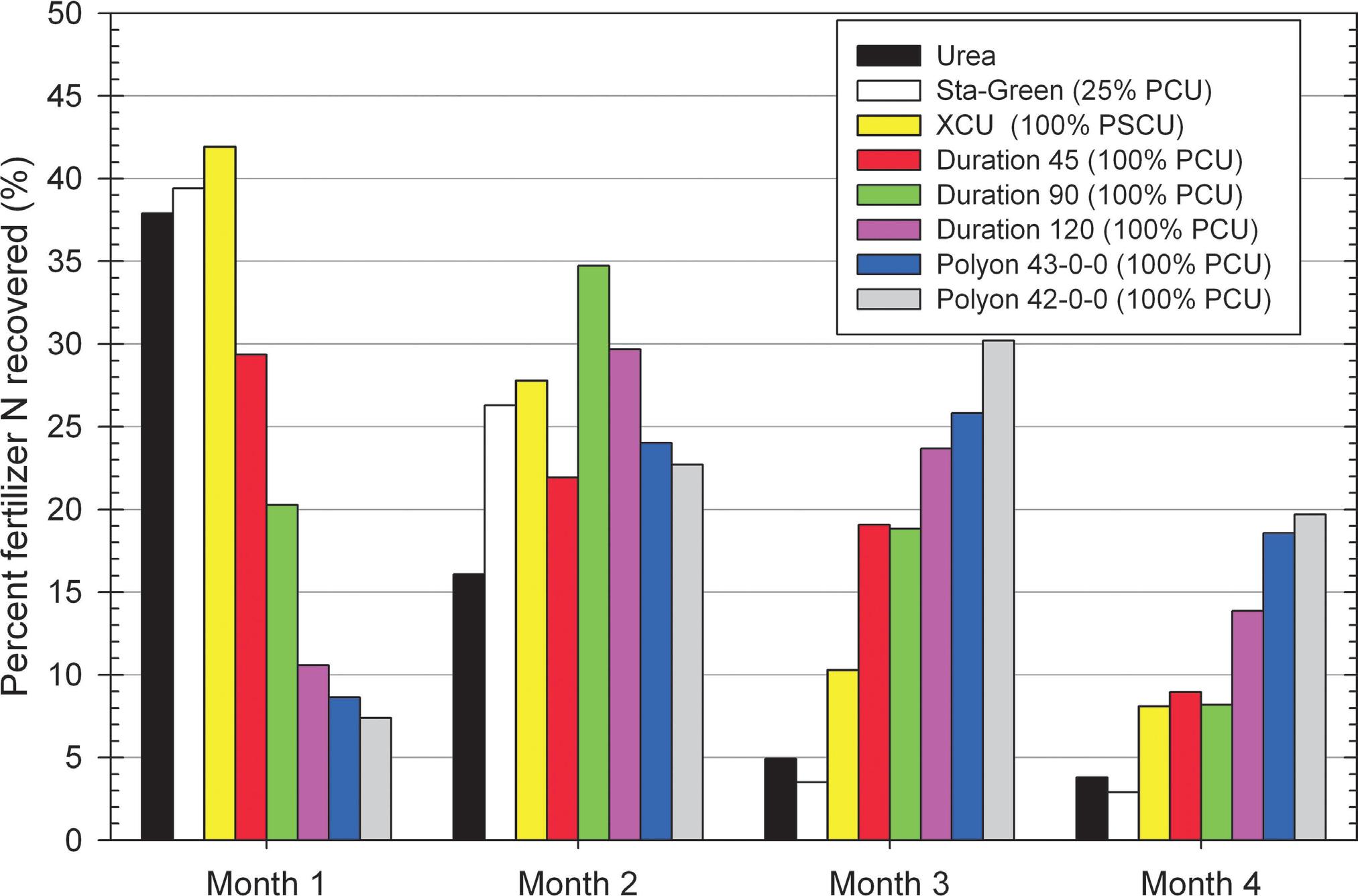
The duration of polymer-coated urea (PCU) fertilizer N release is controlled by impermeable coating thickness and its mass ratio to semi-permeably-coated prills (specific to the blended product). Figure 3 depicts data collected the last 10 years in numerous PSU field trials illustrating the relation of fertilizer N content (%N in grade) to release duration (days until assimilation of 50% of fertilizer N; higher number=slower release). The scatter plot shows little relation between these two parameters when all coated, natural organic, and synthetic urea formaldehyde reaction product fertilizers are considered. Yet regarding PCUs (blue circles), containing only urea and whose N content is diluted by polymer coating thickness, the inverse relationship between PCU fertilizer N content and duration of N release becomes apparent (Figure 3).
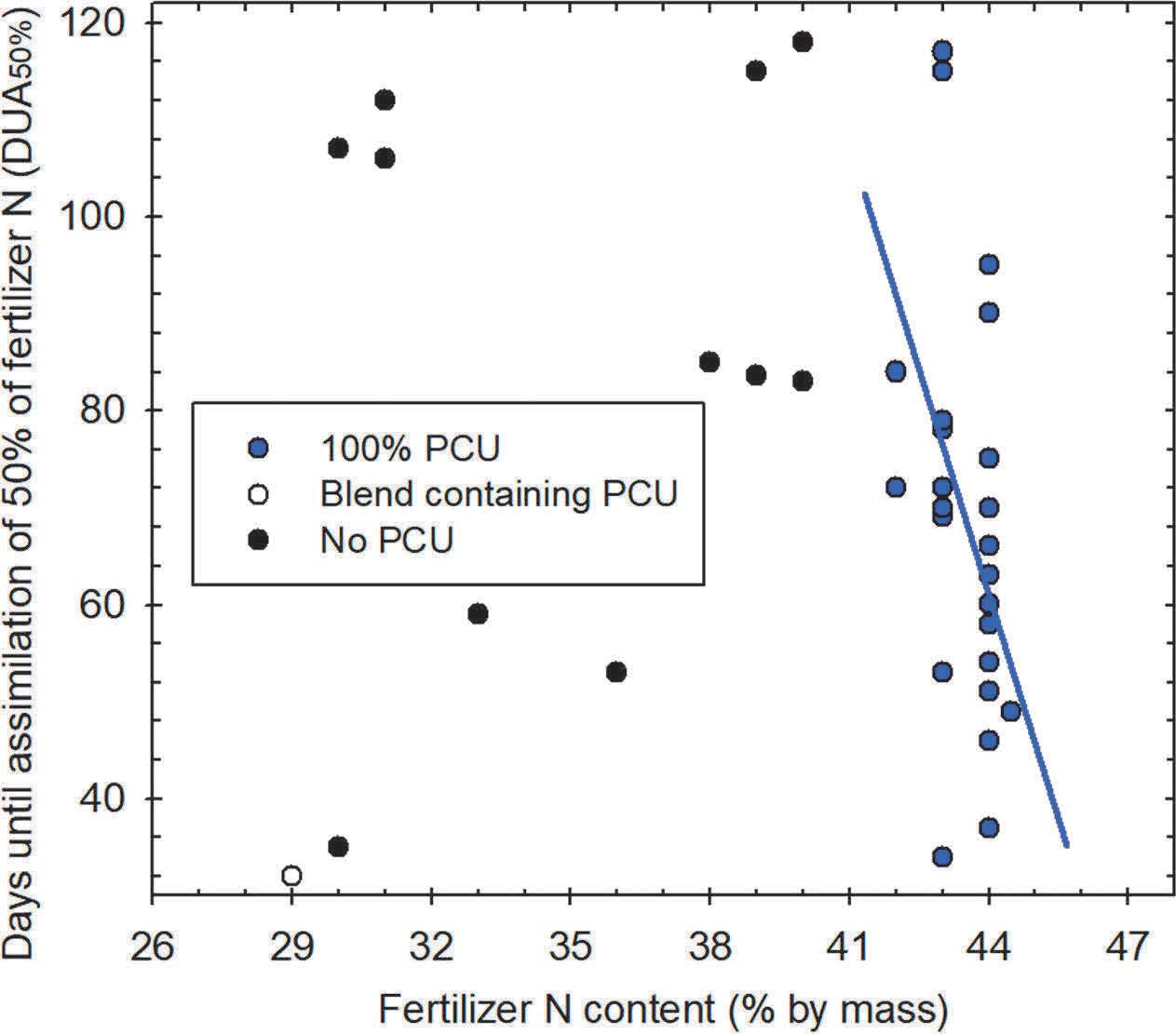
Perhaps of greater interest to our readership is that recent PSU research shows under typical Mid-Atlantic US conditions, a single 1.8 lbs N / 1000 ft2 application of 100% PCU fertilizer to a mature Kentucky bluegrass lawn poses no greater environmental risk than conventional urea applied at a 0.9 lbs N / 1000 ft2 rate.
The current state of affairs…
It wasn’t long before the fertilizer industry branched out from urea to diversify coated fertilizers and appeal to additional commodity markets. Polymer-coated ammonium nitrate and ammonium sulfate are currently available in select regions of the US. If compound fertilizers are more your thing, then you’re encouraged to contact one of the numerous commercial vendors with polymer-coated potassium nitrate or diammonium phosphate available for delivery. There are even polymer-coated complete fertilizers that feature all the primary nutrients in a single bag of controlled-release splendor.
The coating materials currently used in synthesis of PCU, PSCU, or alternative N source fertilizers are polyolefins, polyvinylidene chloride, acrylic resin, and polysulfone synthetic polymers. And despite years of woeful commitment to collecting and sorting used plastic containers in support of recycling, these polymeric materials continue to be sourced from non-renewable resources. Furthermore, the very properties of polymers that make them such effective barriers to nutrient diffusion contribute to their persistence in nature and resulting microplastic pollution.
Which all begs the following question: ‘Do the negative environmental impacts associated with the manufacture and application of PCU fertilizers outweigh their proven agronomic benefits?’ This is sure to spawn an extraordinary complex answer... one I’d certainly open with ‘It depends.’ But that’s as far as I can currently go with this apples to oranges comparison, perhaps better posed as: ‘How many grams of legacy microplastic is offset by a pound of urea not volatilized as NH3 or N2O?’ Once I am notified this article is off to press, perhaps I’ll ask ChatGPT.
Like some scientists, I appreciate objectivity and have been reading up on experimental fertilizer coatings accordingly. Which seems understated considering the last Web of Science search I queried using ‘fert*’ and ‘coat*’ as keywords returned 1,511 papers published since 2018! I’ll be honest, I didn’t read the whole lot. But please... I’m an author on two of them anyhow.
To summarize, the majority of material scientists currently developing and evaluating innovative urea coatings do not believe the agronomic benefits of polymer coatings outweigh the negatives. Rather, they’re hellbent on preserving humanity by replacing synthetic polymer coatings with biodegradable membranes. But in the spirit of objectivity, they may not be hellbent, perhaps just allergic to plastic. Deathly allergic, like the Wicked Witch of the West to water kind of allergic. Ding Dong!
Nevertheless, recent advancements in nanotechnology and material science afford us a wide array of encapsulation technologies and unlimited possibilities. Most research has focused on renewable and biodegradable chemistries, and/or efficient coating processes. Compounds that have recently been investigated as a fertilizer coating and described in a peer-reviewed publication include (among others): alginate, biochar (of course biochar, as if there is anything it can’t improve), cassava (Manihot esculenta) starch composite film, castor oil, cellulose, chitin/chitosan, graphene oxide, humus, lignin, natural rubber, pectin, phase-transitioned whey protein, xanthan gum, and zeolite.
The future of coated fertilizers…
In one recent paper, scientists polymerized castor oil and magnetite (Fe 3O 4) nanoparticles into a cross-linked membrane with high thermal stability. This is an intriguing approach that checks both the ‘recycles waste oil’ and ‘contains nanoparticles’ boxes, while relieving current industry reliance on non-renewable fossil fuels.
Lignin also comprises an organic fiber of great potential, primarily due to its high carbon content, biodegradability, tensile strength, and oxidation resistance. As a component of slow-release fertilizer coatings, lignin is shown to delay the release of fertilizer nutrients and subsequently improve water holding capacity and quality of treated soils.
The above are a few of the more promising results my literature review revealed. It seems the most likely progression in coating technology will be avoiding non-renewable resources and incorporating agronomically advantageous compounds. Such additives may include biological agents; e.g., beneficial microbes, NBPT (urease inhibitor), dicyandimide and nitrapyrin (nitrification inhibitors), biostimulants; and/or surfactants, micronutrients, zeolite, etc.
Further along the innovation pipeline await ‘smart coatings.’ A given name I hope will do as much for coatings as phones. The only thing I love more than my smart phone is keeping it encased in acrylic, charged, dry, financed, and continuously updating its operating software and 37 apps I can’t open. But smart coatings will ultimately have to earn that lofty title by filing tax forms, graphing your caloric intake by week, and releasing nutrients in response to environmental stimuli like temperature, moisture, and/or turfgrass uptake. I hope I am around long enough to evaluate that last responsibility in the field.
Which seems like a decent segue to my take-home point: an important and final aspect of fertilizer innovation is unbiased, thirdparty evaluation in the field. Perhaps the most entertaining parts of those 1,511 papers, or less (that I read), were the agronomic validation efforts directed by the very material scientist(s) who engineered the experimental coatings. Many of which I would characterize as somewhere between marginally critical and straight-up flimsy; i.e., conducted in a glasshouse over short duration, limited to 2 or 3 replications, and offset by peculiar positive controls.
As you assimilate new fertilizer, amendments, and/or pesticide promotions; please remind vendors and technical reps of your insistence on impartial, University-grade field research results when making purchasing decisions. Field trials capture highly-variable growing conditions that may exacerbate limitations, or advantages, of new or experimental products. Likewise, University personnel will include appropriate positive controls; i.e., respected industry bellwethers that support practical new product assessment.
Thank you for your ongoing support and interest and please send comments / questions to me at mjs38@psu.edu!
This article was originally published in Pennsylvania Turfgrass magazine, Fall 2023 and is reprinted with permission.