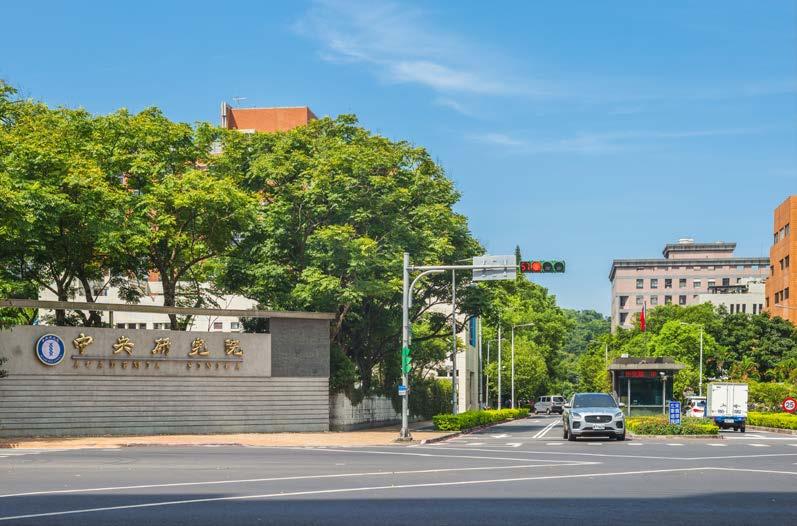
64 minute read
› Interview: Yuan-Tseh Lee: Brain Recycling
Meeting global challenges to secure our global village
by Ehud Keinan
Advertisement
https://doi.org/10.51167/acm00012
Interview with Prof. Yuan Tseh Lee, Academia Sinica It was a sunny day in the early afternoon of September 22, 2014. I visited Prof. Lee at his office in the modern, high-rise building of the Genomic Research Center. Through the large windows of the spacious office, sitting over high-quality Oolong tea, I could enjoy the magnificent view of the Academia Sinica campus.
Ehud Keinan
Professor Keinan of the Technion is President of the Israel Chemical Society, Editorin-Chief of AsiaChem and the Israel Journal of Chemistry, Council Member of the Wolf Foundation, IUPAC Bureau Member, and past Board Member of EuChemS. He was Dean of Chemistry at the Technion, Head of the Institute of Catalysis, and Adjunct Professor at The Scripps Research Institute in California. His research program includes biocatalysis, organic synthesis, molecular computing, supramolecular chemistry, and drug discovery. He received the New England Award, the Herschel-Rich Award, the Henri Taub Prize, the Schulich Prize, the Asia-Pacific Triple E Award, AAAS Fellowship, and the EuChemS Award of Service. PROFESSOR LEE, THE world knows much about your scientific achievements, particularly after winning the 1986 Nobel Prize in Chemistry with Dudley Herschbach and John Polanyi. Most chemists know about your work on the use of advanced chemical kinetics and crossed molecular beams to investigate and manipulate the behavior of chemical reactions. Since many youngsters, particularly in Asia, take you as a role model, I wish to focus on the very beginning of your life journey and talk about your childhood. If these Asian kids could understand what has attracted you to science, we may gain new generations of excellent scientists. My first question is, how early in your life has science triggered your curiosity?
I was born in Hsinchu in 1936 and started my elementary school during WWII before the American Airforce started bombing Taiwan daily. That experience contributed much to my resilience. To avoid the American bombing of Hsinchu, my mother, myself, younger brother, and sister ran away to the mountains and stayed there as refugees for two years. That period in the mountains was the happiest time of my life. I learned how to live with the farmers, working very hard to survive without electricity and running water.
I didn’t go to school in those two years. I am number 3 of nine siblings, five brothers and four sisters. My older brothers and my father remained in the city because the Japanese authorities did not allow them to go to the mountains. They had to help as firefighters when needed and do other services. Much of the time, they lived in a shelter. Life was not easy in the mountains. I was seven years old at that time, and my elder sister, who was nine, had to carry the water from the bottom of the hill up several trips every day and helped the farmers planting, fishing, etc. It was a wonderful time for me, and I remember the change of seasons with birds nesting and us catching eggs, and I was puzzled by the wonders of nature, things that young children these days don’t have a chance to experience.
When and why did you consider becoming a scientist?
When I lived in the mountains during the extensive US bombing, the US Airforce didn’t know that the Japanese didn’t have radar systems, so they always distributed tin foil
flakes, and we, as kids, used to collect boxes of tin foil. I was asking myself when it was safe for me to go out and collect the tin foil. I learned that because of the principle of conservation of momentum, when the plane is already above you, it is safe, and the bombs cannot reach you. Also, the bombs technology attracted me to learn chemistry. I can say that war technology stirred in me much interest, and already in Junior school, I decided to become a scientist. My science teacher was impressed by me and told me, “you are hopeful.” Already in 5th grade, I was reading many things. I remember one cartoon that impressed me very much: a little sheep goes to a chemistry lab, talking to a chemist wearing a lab coat, asking, “can you change my wool into nylon?” I was impressed because it demonstrated that scientists could make artificial things better than those existing in nature. After WWII, nylon stockings became so popular among women, symbolizing the triumph of science. When I finished 5th grade, I read a book on how the Soviet Union, under a 5-year plan, changed a very backward agricultural society into an advanced industrial society, highlighting the benefits of science and technology. When I was in 6th grade, my mother gave me a red envelope with some money on the Chinese Lunar new year, as most other Chinese kids received. My brother went to the bookstore to buy books with this money, and I went with him. There I found magazines for elementary school, published in Shanghai. I read an article named “blue carpet,” describing Russia’s social revolution, describing how a slave on the farm became a master of his land. I was so excited that people could change society if they worked hard, which gave me much hope that our society could be improved. In high school, when I read the biography of Marie Curie, written by her daughter, I realized that a scientist’s life could be beautiful and exciting and full of discoveries, so I wanted to become a scientist.
Your childhood experience gives me the impression that you grew up as a lonely boy with books and thoughts. What about family and friends?
Oh, I had many friends, brothers and sisters, and cousins, all living in the same block. I must say that my mother had a significant influence on my development as a scientist. In those days, only highly talented people could compete in the exams for high school, and my mother did. After graduating from high school, she stayed one more year to become an elementary school teacher. I remember that she always challenged me with many philosophical questions, and often with mechanical problems. For example, when the iron broke, she asked me if I could fix it. I took it apart very carefully, learned how electricity worked, and where did the shortage occur. I learned which line was the high voltage and where to connect the cables, and I finally fixed it. I think I was that time in 9th grade in junior high school.
I remember another case with a Singer sewing machine with a foot pedal that was very noisy, and my mother used to work on it at night. I complained about the noise, and my mother responded: why are you complaining? Fix it and make it quieter. I took the machine apart, oiled it, replaced and tightened some bolts and nuts until it became very quiet. But my mom said then the belt is too tense at one side and too loose on the other, so I took the head apart and fixed it again and adjusted it properly.
Where did you pick up the electrical and mechanical education needed for such tasks?
The mechanical information I learned just from taking things apart and observing how they work together. After the war, my cousin came back from Japan, where he attended a technical school, and he taught me not to be afraid of high voltage cord as long as I insulate my body from the ground. I could even touch the high voltage cord if sitting on a couch.
As an amateur technician, did you seek any help from others?
No, I did it all by myself. My father, who was an artist, a very handy man, influenced me much. You can see some of his framed paintings decorating the walls of my office. He started his career as an art teacher in elementary school, climbed up rapidly to Junior high, then to high school, and finally became a university lecturer. He died at age 73, one year after I received the Nobel Prize, and I was so happy that he managed to witness the ceremony in Stockholm. He was overwhelmed by so many people coming to greet him. That time I was still in California. When I came back to Taiwan, my mother was still here. She died at age 87.
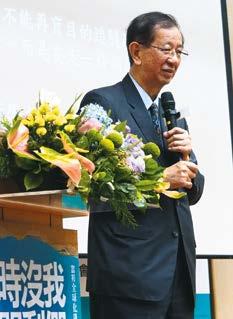
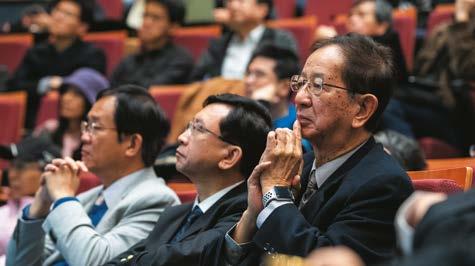
Did you receive a good formal education to become a scientist?
In general, the answer is yes; my formal education was right. My school gave me enough space and time to grow without putting too much pressure on me. The worst type of education is a school that takes up
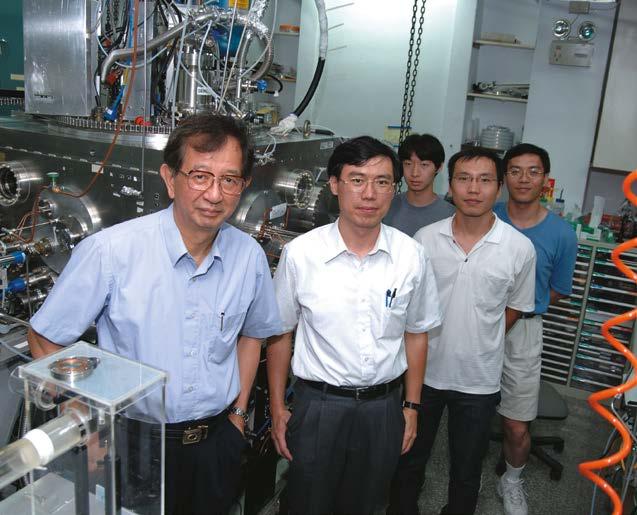
Rather than using the term brain drain, I prefer brain circulation. Young people need to go to other countries to complement their education and then come back.
all your time and does not leave you space to develop. By the time I became a 10th-grade pupil at junior high school, I was already very independent. After 10th grade, I studied almost everything by myself. I read many books on science and social science and learned enough math and physics to match the 12th-grade level.
At that time, I had too many activities and exhausted myself to such a degree that I had to stay home for one month to recover. I was a tennis player, table-tennis player, baseball player, and I played trombone in a brass band. I was active in the boy scouts and went to camps until I fell sick of exhaustion. The doctor forced me to stay at home for one month, which allowed me to think of the meaning of life. I was a quite rebellious youngster, didn’t want to be controlled by either school or society, always wanted to be the master of myself. I often annoyed my teachers by asking for lots of difficult questions.
After that month of thinking and reflection, I remember that I went back to school as a different person. I learned how to use time productively, a skill that was very beneficial to my entire career. When I went to university, I had two goals in mind. My primary dream was to become a scientist to contribute to society. Not less important, I wanted to work with idealistic people to advance society in general. I have realized that any person with exceptional skills in any given field can benefit society and push it forward.
Brain drain has been a notoriously known problem in Taiwan. Are you trying to remedy this problem?
It has not only been a Taiwanese issue but an Asian problem. In the 1960s and 70s, we suffered a significant brain drain. Many young people, including myself, went to America and stayed there. But now, during the last two decades, many established scientists, including members of the National Academy of Sciences, have returned to Taiwan. I prefer not to use the term brain drain because scientists need to go to other countries to complement their education. A more appropriate term would be brain circulation. Young people go out to learn something and get new experience and then come back.
As Taiwan’s situation keeps improving, more and more students like to stay at home rather than abroad. We still support them with scholarships and send them out to foreign countries because they need to see the world before developing their independent career. We can see many Asian scholars returning to their native countries and make a real difference by boosting their development. It is no longer a one-way brain drain, but rather brain circulation.
Did you ever fulfill your desire to make the world a better place?
I think I was lucky to be able to do some good things, at least for my own country. In 1994 I received an invitation to become President of Academia Sinica. I thought that at age 57, it might be a good time to return to my home country to contribute to the place I grew up in, and I wanted to do it before I became an old man. After spending 32 years in the USA, I left Berkeley, gave up my US citizenship, and returned to Taiwan with my wife, whereas our three grownup children preferred to stay in the USA.
Academia Sinica is Taiwan’s top research body. It has over 30 research institutes, covering the humanities, social sciences, the physical and biological sciences. During my tenure as President of Academia Sinica, from 1994 to 2006, I worked hard to improve research quality to make it a world-class institution.
Winning the Nobel Prize allowed me to serve various segments of society. As President of Academia Sinica, I could play a significant role in shaping educational and scientific policy in Taiwan. Since I reported directly to the President of Taiwan and acted as his senior science adviser, I could talk to him anytime I wanted. As the Chair of Taiwan’s Council of Educational Reform, I tried to advocate democratization, professionalism, and university autonomy. I have also led a national organization for community empowerment in Taiwan. I chaired a nonpartisan group that advised the President on matters concerning the relations between Taiwan and
China. I have established several new organizations that support education and research activities, etc. In general, I have done everything I could to revamp the Taiwanese education system and to enhance creativity and innovation.
Did you manage to influence other parts of the world as well?
My goal of working with idealistic people to make the world a better place stayed unchanged over the years. I have always been interested in higher education’s direction and responsibilities, the development of creative scientists in Asia, the future of humankind, the futility of war, and environmental challenges, such as global warming. We all live in a global village, and we need to join forces to meet global challenges. To advance these ideas, I served on the International Council for Science (ICS), first as President-elect and (2008-2011) and then as President (2011-2014). I also served as President of the Tan Kah Kee International Society, a significant foundation based in Singapore, dedicated to promoting education as a means of advancing democracy and development. In many of my public lectures, I try to raise public awareness of environmental issues. I think that the greatest danger to humanity is climate change, which is even more alarming than a nuclear war. For the first time, human civilization can change the environment to the point where it can no longer support life. Since we are facing a global problem, neither a single country nor scientists can solve it alone. Suppose we learn to connect knowledge to action, establish better international institutions, and coordinate all global efforts. In that case, we may save the world from global warming before the middle of this century.
I am happy to realize that both of us share optimistic views concerning the future of science and development in Asia. Thank you very much for sharing your experience and opinions with the readers of AsiaChem.
EPILOGUE: THIS INTERVIEW took place in September 2014. Now, six years later, I reminded Prof. Lee of that interview. After looking at it, he responded, “Although almost six years passed, the content is still fresh in my mind. I would not answer any of your questions differently. I will be delighted to see this article published in AsiaChem and would like to express my admiration for taking up such a major responsibility. I do believe that your efforts will strongly impact students in Asia.” ◆
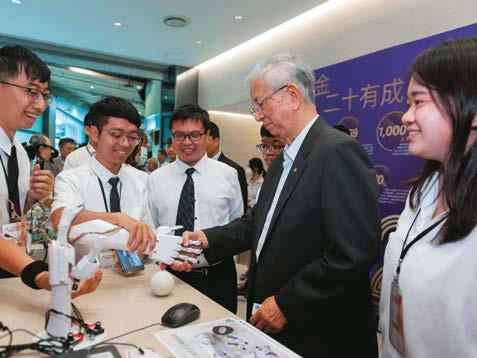
I have always been interested in higher education in Asia, the future of humankind, the futility of war, and our environmental challenges.
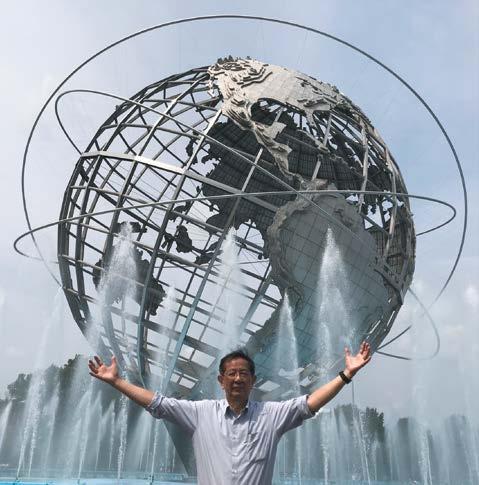
Goverdhan Mehta
Prof. Goverdhan Mehta is an organic chemist who has worked for many years at the University of Hyderabad, where he was a Professor, Founder Dean and Vice-Chancellor. He was also Director of the Indian Institute of Science in Bangalore and has held chairs, visiting and honorary positions in India, Belgium, France, Germany and USA. He is a Fellow of the Indian National Science Academy, serving as President in 1999 – 2001, and a Fellow of the Royal Society, London and of numerous other societies. He is distinguished for his work in organic synthesis, for which he has received many awards and prizes. He is currently University Distinguished Professor and Dr. Kallam Anji Reddy Chair in the School of Chemistry at the University of Hyderabad.
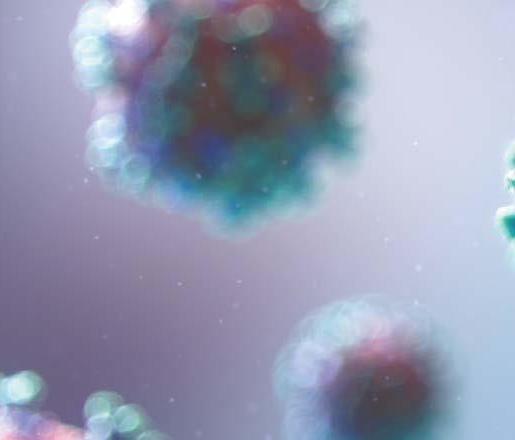
Henning Hopf
Prof. Henning Hopf is an organic chemist who studied in Germany and USA. He became professor in Würzburg and was then appointed to the Chair of Organic Chemistry of the Technical University of Braunschweig (TUB), where he was Managing Director of the Institute of Organic Chemistry until his retirement. He was President of the German Chemical Society and a member of the Göttingen, North Rhine-Westphalian and Norwegian Academies of Sciences. His work has been concerned with cyclophanes, highly unsaturated hydrocarbons and aromatic compounds. He has been awarded many prizes and medals, including the Chemistry Prize of the Göttingen Academy of Sciences and the Max Planck Research Award. He is currently an Emeritus Professor in the TUB. Alain Krief
Prof Alain Krief is widely known for his contributions to organic synthesis, including work on organo-selenium chemistry. He is currently also pursuing work in chemical informatics. For many years he was Director of the Laboratory of Organic Chemistry at the University Notre Dame de la Paix in Namur, Belgium and subsequently an Emeritus Professor there. A French citizen born in Tunisia, he studied in France, the UK and USA and has been a visiting professor at more than 15 universities worldwide. He has been the recipient of many prizes and medals, including the Prize of the French Academy of Sciences. In 2009- 2020 he has held the position of Executive Director of the International Organization for Chemical Sciences in Development.
Stephen A. Matlin
Prof. Stephen Matlin is an organic chemist who served as Professor in Biological Chemistry in City University, London and Warwick University. He then worked in international development, appointmented as Director of the health and education division of the Commonwealth Secretariat, Chief Education Adviser in the UK Department for International Development and Executive Director of the Global Forum for Health Research in Geneva. His awards include the Edmund White Prize at Imperial College London and Kelvin Lectureship of the British Association for the Advancement of Science. He is currently a Visiting Professor in the Institute of Global Health Innovation at Imperial College London and Senior Fellow in the Global Health Centre at the Graduate Institute, Geneva.
Chemistry in a post-Covid-19 world
The long-term impacts of global upheaval unleashed by Covid-19 on economic, political, social configurations, trade, everyday life in general and broader planetary sustainability issues are still unfolding and a full assessment will take some time. However, in the short term, the disruptive effects of the pandemic on health, education and behaviours and on science and education have already manifested themselves profoundly – and the chemistry arena is also deeply affected. There will be ramifications for many facets of chemistry’s ambit, including how it repositions itself and how it is taught, researched, practiced and resourced within the rapidly shifting post-Covid-19 contexts. The implications for chemistry are discussed here under three broad headings, relating to trends (a) within the field of knowledge transfer; (b) in knowledge application and translational research; and (c) affecting academic/professional life.
Historically, pandemics have forced humans to break with the past and imagine their world anew. This one is no different. It is a portal, a gateway between one world and the next.”
— Arundhati Roy1
THE WORLD IN general will not be same after Covid-192. The new disease, first recognised in late 2019, was the direct cause of more than 45 million recorded cases and 1.1 million deaths by November 20203 and many more could be expected before the pandemic abated. In addition, there is ongoing debate4 about the possible and probable magnitude of the impacts of the pandemic on economics (including shortterm dramatic declines in GDP and increases in poverty), employment, the environment, international development and cooperation, politics and society. The whole of science5 and the domains of universities6,7, and industry8 in which it operates are being greatly affected. Numerous areas of human affairs, including the world of science, are likely to become differentiated as ‘before’ and ‘after’ Covid-19. Chemistry is no exception – and is of particular importance as its capacities are central to combatting such global threats as Covid-19 and protecting lives and the planetary environment.
by Goverdhan Meht, Alain Krief, Henning Hopf and Stephen A. Matlin
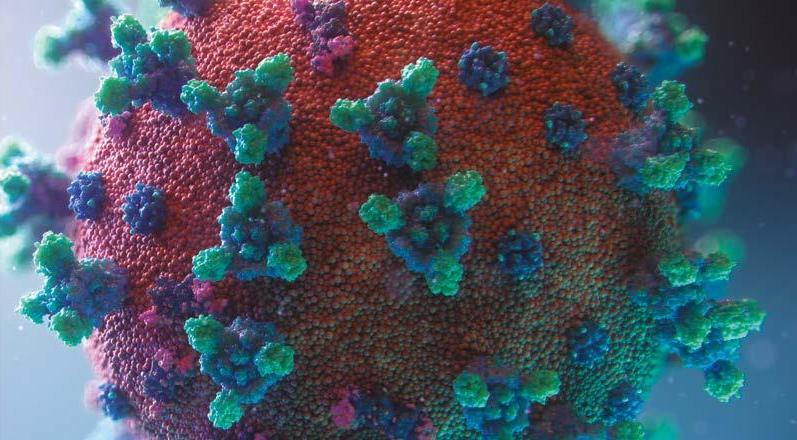
https://doi.org/10.51167/acm00013
The emerging landscape of chemistry
This article attempts to capture some of the main trends and directions for the field of chemistry in this pivotal period – to signpost possibilities, opportunities and challenges in a rapidly evolving landscape. Kekulé (the 19th century chemistry icon and discoverer of the structure of benzene) is often quoted9 for his articulation of the character of chemistry as “the science of the metamorphosis of matter. Its real subject is not the existing matter, but its past and its future. The relationship of a compound to its past and what can become of it, is the true essence of chemistry.” Complementing this insight, it must also be recognised that the subject and practice of chemistry itself changes over time – and that it is presently passing through a period of considerable and diverse metamorphoses. Many of the changes currently underway are being accelerated or reoriented by the global upheavals resulting from the Covid-19 pandemic, so that even though this event may not be the original cause of most of the changes in chemistry it can be seen as an exceptionally powerful accelerator.
Within the continuously evolving landscape of chemistry, while fundamental breakthroughs in this mature discipline are now quite sporadic, major advances that go beyond incremental accretion of knowledge in this central science are primarily driven by contemporaneous developments in adjacent fields and its role as ‘quality of life’ science. This is an inevitable consequence of chemistry’s dual character as a science that, in dealing with the material basis of the world, both pursues knowledge and seeks applications. Three particular sources of stimulus for developments in chemistry as a discipline are notable: (a) Advances in theory or practice in other disciplines (e.g. physics breakthroughs in quantum mechanics and various spectroscopic techniques) and availability of advanced computing and visualization tools for simulation and modelling, which chemists have been very successful in adopting and applying to their own challenges, have provided fresh insights into atomic and molecular structure and properties; (b) New approaches to analytical tools and structural analysis, including advanced separation protocols (from fractional distillation and chromatography to use of membranes, supercritical extraction and centrifugal/cyclonic methods) and tools for structure determination (from early UV, IR, NMR and MS to advanced methods like electron microscopy, atomic force microscopy and hyphenated techniques such as LC-NMR, LC-MS, LC-QTOF
MS, synchrotron-microcrystallography) have enabled chemists to analyse complex materials and isolate and identify novel structures with increasing rapidity.
Concurrently capacities have been developed to operate at smaller scales (milli- to pico-molar) and faster time scales (milli- to femto-second), enabling real-time observations at atomic and molecular levels. (c) In cross-disciplinary work, cutting edge breakthroughs at the interfaces with other disciplines (e.g. biological and solid-state sciences) have synergistically advanced understanding and driven practical applications of chemistry in areas like engineering and medicine that aim to satisfy present and potential future societal needs or desires.
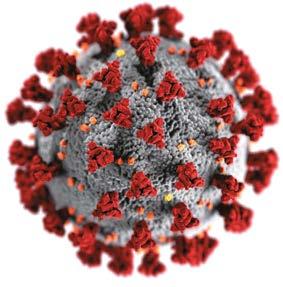
Integrating knowledge and the quest for 21st Century dimensionalities in chemistry
A notable feature in chemistry in recent years has been a desire to advance beyond reductionist approaches, both to within the subject itself and across its interfaces with other disciplines. One important facet of this move towards integrationism and the exploration of new dimensionalities in chemistry has been the effort to enhance directionality, purpose or societal goals, for example to strengthen the contribution of chemistry to achieving the UN Sustainable Development Goals (SDGs) or tackle environmental challenges,
emphasising chemistry’s potential as a core sustainability science.
The changes that are being observed can be classified and analysed in a number of different ways. Here, they have been grouped into three areas – reflecting trends (a) within the field of knowledge transfer; (b) in knowledge application and translational research; and (c) affecting academic/professional life. Of course, all of these are highly interactive and inter-dependent areas and all are situated within the rapidly shifting contexts of 21st Century global affairs including the post Covid -19 scenario.
Chemistry knowledge transfer
Changing a curriculum is like moving a graveyard - you never know how many friends the dead have until you try to move them.”
— Adage ascribed to Woodrow Wilson or Calvin Coolidge
The approach to teaching chemistry that evolved in the 19th-20th Centuries predominantly focused on explaining the fundamental pillars of the subject through lectures, textbooks and laboratory exercises, with some limited attention given to practical applications such as the sources, processes and uses of some selected products. However, the teaching of chemistry has been on an evolutionary trajectory, undergoing major changes involving the content of courses, the nature of knowledge and skills required and how they are assessed, and the process of delivery. Transformations in these areas are being
driven by two cross-cutting, interactive factors, discussed below.
Chemistry’s content, context and interconnections
Chemistry was traditionally taught by a reductionist approach in which, for presumed clarity and ease of learning, topics were broken down into the most basic, ‘fundamental’ elements possible, to be studied one-at-atime and with ‘context’ such as applications or implications for more complex settings dealt with, if at all, as a brief post-script. There have been increasing moves to reorient the teaching of chemistry towards more integrated approaches.10 • Alternative approaches, such as problem-based/inquiry-based learning and context-based learning, became more popular11 in the late 20th and early 21st Centuries, encouraged in part by research-led advances in understanding of learning and pedagogy and of the need to make better connections between facts, concepts and symbols, as well as recognition of the need to reinvigorate chemistry education and make it more relevant to everyday living.12 • The traditional approach of learning ‘fundamentals’ has tended to lead to severe fragmentation in which topics are sub-divided and taught separately and learners find it difficult to make connections between isolated pieces of knowledge, perceive the discipline in an integrated way and apply the understanding gained across sub-areas within chemistry and other disciplines. The evolving emphasis on cross-disciplinary approaches, imparting ability to tackle complex, system-wide problems – such as climate change, waste, clean water and planetary sustainability – has led to a growing desire for a more integrative approach to learning chemistry, that will build competence in such
crucial skills as systems thinking.13 Many universities have already moved to offering courses and research programmes that traverse disciplinary boundaries, These include ones that bridge interfaces between chemistry and other disciplines (such as biology, engineering and medicine), as well as dealing with challenges (e.g. involving systems science, engineering and social and policy connections) such as waste management, water processing and recycling. • A group of chemists committed to promoting sustainability has presented the concept of ‘one-world chemistry’, an approach that incorporates cross-disciplinary working and systems thinking.14 Systems thinking has been identified as one of five key competencies that are essential for a sustainable future15 and consequently a vital skill to be acquired by chemists. Through participation in a project of the International Union of Pure and Applied Chemistry (IUPAC), the roles of system thinking in enhancing the understanding and integration of chemistry and of developing its role in sustainability have been elaborated.13 Furthermore, the systems-oriented concept map extension (SOCME) has been developed as a tool that aids the understanding and visualization of interactions within and between systems.16 • Concerns about global challenges, UN
SDGs, etc, and the overall sustainability of the planetary environment require more than adding information/footnotes to existing chemistry texts. Typical school and undergraduate textbooks carry a historical legacy which is rooted in the idea that the planet has an effectively inexhaustible supply of natural resources. This has been reflected in the way that sources, transformations and applications of inorganic compounds derived from minerals and organic compounds derived from carbon-based fossil deposits have been presented. Chemistry is central to achieving the SDGs and now needs to be repositioned for a sustainable future.17 To take organic chemistry as an example, the ready availability of unfunctionalized aliphatic and aromatic hydrocarbons, downstream from fossil fuels, has conditioned many generations of chemists to emphasize the importance of functionalising C-H bonds and oxidative transformations. A shift to sustainable use of renewable feedstocks, predominantly oxidised and highly functionalised materials, e.g. based on cellulose and lignin, should be mirrored by a re-orientation in the teaching of functional group chemistry and a much greater emphasis on C-O bond manipulations and reductive transformations.18 Overall, there is a distinct trend towards teaching chemistry within a sustainability framework, positioning chemistry as a core sustainability science.19,20
Cross-disciplinary lecture courses have emerged that aim not only to interface or
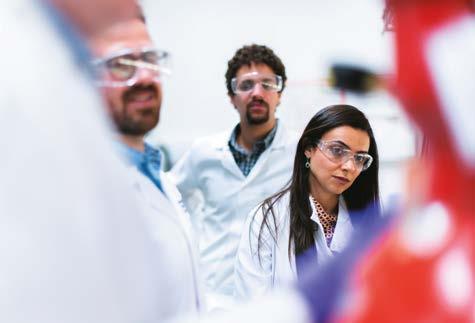
integrate chemistry with physical and biological sciences, but also to broaden the horizon and usher-in societal connections with arts and humanities, including philosophy, psychology and sociology. • Chemistry learning is important for citizens in general, who need to have sufficient knowledge about the nature of the materials they handle in every-day life to make informed judgements about claims and about risks and benefits of particular products and processes. The threats to health and life that Covid-19 has brought have vividly highlighted the need for every citizen to be able to navigate a safe course and to protect themselves and their families and communities by understanding, evaluating, prioritising and applying information with a science/ chemistry angle.
Access and delivery
The approach to transfer of chemistry knowledge that evolved in the 19th-20th Centuries predominantly focused on explaining the fundamental pillars of the subject through face-to-face lectures, tutorials and seminars, complemented by textbooks and laboratory exercises. Transitions towards online/distance learning have been assisted by advances in information and communications technologies (ICTs) and now greatly accelerated by the lock-downs imposed during the Covid-19 pandemic, with major implications for chemistry education. • In recent years new platforms and pedagogies exploring the potential of ‘edutech’ and online teaching have surfaced. Expanding
ICT capacities have encouraged many universities to begin developing on-line elements to their courses and to incorporate augmented/virtual reality21 and visualization elements to assist learners to increase their conceptual understanding, including in areas such as structure, bonding and reactivity. • A prominent impact of Covid-19 in many places has been to accelerate or precipitate a move from face-to-face to virtual ways of engaging and induction of many more people into the work-from-home (WFH) or work from anywhere (WFA) and learn-fromhome (LFH) era. This move has necessitated overcoming resistance to change and acquisition of new skills by both teachers and learners. Post-Covid-19, university education is likely to operate increasingly on a hybrid model, with both face-to-face and
WFH/WFA/LFH virtual modalities operating in parallel or ‘blended’ modes as the future normal. The hybrid system will need to be contextually and geographically specific, sensitive to the economic and social circumstances and living conditions of teachers and students while ensuring high-quality educational outcomes.
Chemistry and health
An example of a field of chemistry teaching and research where revival and reimagination in content, focus and integration are now all timely is that which has been branded for decades as ‘medicinal chemistry’. Traditionally, degree programmes in ‘medicinal chemistry’ have centred on the preparation of sets of compounds (ranging from a handful of examples derived from individual syntheses to extensive libraries generated by combinatorial methods) and on developing an understanding of how structure and physico-chemical characteristics relate to performance at stages such as formulation, absorption, distribution, active-site binding, metabolism and clearance.
It is important that ‘medicinal chemistry’ embraces related areas like pharmacology and biology, as well as the emerging capacities of AI and in silico methods, to help steer drug discovery efforts and avoid being regarded as a service tool; but also that there is recognition of the potential for contributions of chemistry to health to go very much wider than these medicinal/pharmaceutical/drug-discovery areas. The birth of the rapidly advancing arena of ‘molecular medicine’ owes its origin substantially to progress in opportunities opened by medicinal chemistry. The understanding of health itself has broadened from a restricted biomedical view of disease to a much more open model in which other determinants such as environmental, economic, political and social factors also play significant roles.30
A second example that illustrates the importance and value of a unified approach that integrates knowledge and practice within and beyond chemistry is provided by the critical current challenge of sustainability (see below). As a leading sustainability science, chemistry education and research needs to help introduce, build competencies and spearhead innovations in a range of techniques, skills and approaches including life cycle assessment, circularity of materials and systems thinking.
Chemistry and sustainability – working towards material circularity
The evolution from ‘environmental’ to ‘green’ to ‘sustainable’ chemistry31 has reflected the development of an increasingly broad perspective on the nature of the challenges to the planet and of the deep-seated roles that the chemical sciences must play in responding.
The International Year of the Periodic Table in 2019 provided an opportunity for reflection on the material sustainability,32 and brought into clear focus the recognition that dealing with waste by radical improvements in material circularity represents the underlying challenge for sustainability. The need has been emphasised for a comprehensive approach in which chemistry is at the core efforts to clean up, catch up and smarten up in minimising waste in all its forms, as a key enabler of a sustainable post-trash age.33
As an example, case studies have focused on aluminium, plastics and textiles.34 In each case, the challenges for chemistry were highlighted in relation to all stages from the sourcing of feedstocks to the disposal or return to some form of use of the primary material – but also in relation to the comprehensive consideration of all reagents and solid, liquid and gaseous by-products and waste products. The case study on aluminium illustrates the magnitude of many of the challenges that need to be tackled by chemists: while aluminium is one of the most extensively recycled materials on the planet and is often quoted as a model of sustainability and circularity, the large carbon footprint associated with its extraction, refining, applications and recovery, together with the production of massive quantities of by-products during processing (especially Red Mud from the refining of bauxite ore) have major impacts on the environment.
What links these two examples from very different fields is recognition that (a) chemistry itself must be understood as taking a comprehensive and unified view of matter, in which everything is accounted for, rather than focusing exclusively on the desired ‘product’ (as an entity whose use is someone else’s concern) and ignoring the ‘by-products’ (which are viewed as worthless waste); (b) chemistry can and must be central to understanding and helping determine the subsequent fate of all materials involved in the processes adopted, whether it is the creation of a substance for use in a pharmaceutical or medical device or in the delivery of a functional product for any other application; and (c) chemistry’s central contributions are increasingly at and across its interfaces with a host of other disciplines and chemists need to nurture and expand their proficiency in conducting collaborative research in these complex domains.
Healthy people
Healthy animals
Healthy environment
Sustainable development
Education, research & practice in the chemical sciences
Chemical & materials industries, agriculture food & fisheries Environmental monitoring, protection & preservation, cleaning
The chemical sciences
Figure 1 The chemical sciences support sustainable development
The precipitate shifts by many institutions into the new modes of delivery, occasioned by Covid-19, should not be allowed to overshadow the need for fundamental revisions to the content of chemistry education. As pointed out by Talanquer et al22 in drawing lessons from the pandemic, “As chemistry educators, we cannot stand idly by and simply translate what we have done for more than 50 years into a virtual format to ensure its existence for 50 years more.”
Knowledge application and translational research
Nature also has her laboratory, which is the universe, and there she is incessantly employed in chemical operations.”
— Jane Marcet23 (1858)
Trends in chemistry research
Both curiosity-driven scientific research and utility-driven endeavours are sometimes profoundly influenced by the trends/fashions of the day, which in turn are determined by the major breakthroughs of the time such as discovery of C60, the birth of nanoscience and mapping of the human genome. Chemistry is no exception and the formulation of the Woodward-Hoffmann rules, advent of supramolecular chemistry, recognition of super-conducting perovskites and solar cell materials, metal organic frameworks (MOFs: as sensors and energy storage materials), asymmetric catalysis, enzyme engineering for directed evolution, insights into electronic structures through ab-initio and density-functional theory (DFT) methods and single-molecule-spectroscopy are some examples of contemporary trends. There is also a profound influence of funding priorities in areas believed to be of strategic or economic importance (e.g. CO2 fixation, energy storage systems, conducting polymers, photo-electrolysis and H2 production to name a few). Emergence of new processes, equipment and techniques also define new trends e.g. metal-mediated coupling reactions, flow chemistry, digitisation, robotics, 3D printed chemistry vessels, reactions in or on water and ‘no work-up’ regimes for chemical transformations. Such fashions may appear in academia and/or industry (the latter being heavily influenced by potentials for value-creating applications in areas such as new materials related to health, energy or ICT). In addition, broader trends running across the areas of focus are sometimes evident – such as increasing attention to positioning chemistry research ‘closer to nature’ in an effort to reduce the energy and material requirements needed to make products.
Such concentration of effort in a trending field can boost its advancement and may lead to important breakthroughs and valuable applications, and this tendency will undoubtedly continue. However, there are also down-sides. For example, disproportionate fascination with the fashions of the day can distort funding trends, while other areas that might have made advances of fundamental and practical importance may be starved of resources and freedom to innovate constrained. The evolution of more balanced and open processes in resource allocations is now urgently needed to ensure that disciplines like chemistry remain agile, flexible and open to innovation, while directing appropriate levels and proportions of resources to the most pressing priorities.
Refreshing chemistry: a unifying perspective
Strands of chemistry research engagement have always included studies of atomic and molecular structure, properties and dynamics of processes, synthesis and transformation of matter. Increasingly, as chemistry entities have found applications in newer smart materials and products across extremely diverse biological and everyday lifestyle materials, the focus of attention in chemistry research is transitioning towards utilitarian aspects and synthesis of properties rather than of structure per se. 24 In the emergent scenario, the traditional cubicalization of chemistry into components such as organic, inorganic, physical, analytical, etc appears increasingly redundant and must fade away.
Moreover, throughout its history, chemistry has engaged in both blue-sky research for knowledge advancement and research focused on useful applications. Divisions into ‘pure’ and ‘applied’ research are unhelpful, since the former inevitably leads (sooner or later) to the latter and many fundamental breakthroughs have emerged during the course of seeking solutions to specific problems. Nevertheless, funders (whether allocating government grants or making industrial investments) have placed increasing demands on the applicant to predict ‘impact’ as a key requirement, with direction of available resources towards strategic goals.
The time is now ripe for a more unified view of chemistry to taken in the way the discipline is organized, practiced and resourced. An added important factor in support of this is the growing recognition and centre-staging of global 21st Century challenges to human security25,26 and the planetary environment.27,28 Finding credible, sustainable solutions requires concerted effort of the entire dimensionality of the discipline of chemistry, in concert with its sibling and overlapping disciplines.
This concept of a unified approach has found expression in the formulation of the mutually reinforcing SDGs, in the ‘one health’ movement which recognises the inter-dependence of the health/wellbeing of human beings, animals and the environment and in ‘one-world chemistry’ approach that was referred to in earlier. The chemical sciences underpin finding solutions to all these interconnected sustainable development challenges (Figure 1).
Changes in priorities for science and in the types and contents of research will necessarily have implications for research funding, which may need to be reoriented or repurposed. Inevitably, there will be a rebalancing of the roles of scientists and policy-makers in determining the priorities, with the influence of policy-makers likely to grow – and with it the
need for scientists to engage effectively in the debate. This rebalancing may be framed as a ‘loss of freedom’ for science or, more productively, as an opportunity for science to gain importance and influence in national policy-making. Chemists should ensure that they are participants in the dialogue.
An illustration of how a unified approach, where revival and reimagination in content, focus and integration in a field of chemistry are now all timely, is that area which has been branded for decades as ‘medicinal chemistry’. This could be transformed into a broader, coherent field of ‘chemistry and health’ (see page XX). The Covid-19 pandemic has highlighted the centrality of chemistry to health. Its disruptive effects may now help to provide the activation energy necessary to achieve deep-seated reforms to define ’chemistry and health’ as a distinct field in both teaching and research.29
The digital transition and automation in chemistry
The impact of ICTs and the digital transition has increasingly been seen in many areas of chemistry. These include the screening and optimization of reaction conditions, selection of efficient synthetic pathways, automation of synthetic processes and identification of potential new drug molecules. The age of the ‘robochemist’ has been heralded35 and process chemistry and chemical manufacturing are poised to go fully digital.36 These rapidly-advancing developments necessitate a fundamental rethink about what chemistry researchers should do37 and consequently what they must learn, including what kinds of experimental skills they need to acquire and how ingenuity and innovative talents in molecular level manipulations will be nurtured and assessed. Chemistry research supervisors and managers need to reflect rapidly on these questions and develop practical responses.
Reconceptualising research collaborations
It has been increasingly recognised that no single discipline, sector or country will solve the challenges of sustainable development alone and that collaboration is the key.38 Stern39 has contended that internationalism is a critical force for driving sustainable development in the 21st century. In science generally, a trend towards multi-centre, often international research collaborations has been underway for many years and, at least in the short term, has been accentuated in the response to Covid-19. Since the mid-20th Century, multilateral scientific collaborations led, among many positive fruits for humanity, to the global eradication or control of diseases, prevention of destruction of the stratospheric ozone layer and improved agricultural production that helped to feed the world’s rapidly burgeoning population, as well as advances in many fundamental science areas. While chemistry played a major role in all of these, in the past, chemistry engaged in large-scale, multi-centre collaborations to a much smaller extent than some other disciplines, such as physics and biology.40 In the new post-Covid-19 times, there are substantial opportunities for chemistry to strengthen its position as a responsive solution provider for societal wellbeing, with increased emphasis on problem-oriented research.
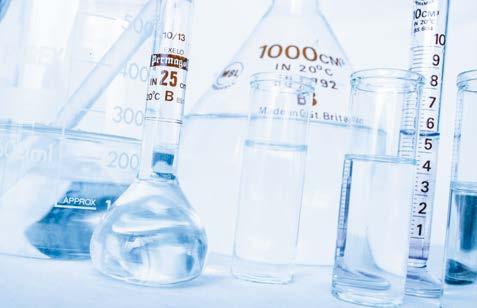
Research, development, innovations – Liebig’s legacy and the industry connect
Among major trends that have been under way in chemical industries during the last few decades, increasing automation, greater attention to the ‘greening’ of chemicals production and heightened concerns about the management of effluents have been impacting on approaches within the factory gates. At a broader level, the nature of the field has been transformed by condensation of the entire industry through mergers and acquisitions since the 1990s, but also increased focusing on ‘core business’ by the new actors. There has been a shift to outsourcing of many components/feedstocks of supply chains and adoption of ‘just in time’ stock approaches; and the overall dis-integration of some major areas. This has been particularly visible in the pharmaceutical industry, with research, development and production being outsourced and new spaces created for the entry of research start-ups, contract research and development companies and clinical trials businesses and organizations.
Whether these developments have all been good for science, health and national economies has been the subject of much debate. What is evident is that Covid-19 has had a dramatic short-term impact on both the focus and functioning of chemical industries. Renewed interest in vaccine development and in the repurposing of drugs41 have been prominent features of the immediate industry response – along with heightened efforts to collaborate. For example, two front-runner drugs for Covid-19 management, favipiravir and remdesivir, have been out-licensed by the innovator companies to manufactures in different parts of the world. AstraZeneca has demonstrated commitment to broad and equitable global access to the University of Oxford’s potential Covid-19 vaccine, AZD1222, through agreements with the Coalition for Epidemic Preparedness Innovations (CEPI), Gavi the Vaccine Alliance, and the Serum Institute of India (SII). A number of supply chains are being built in parallel across the world to support global access at no profit during the pandemic.42 As a harbinger of what may be anticipated in future disruptions caused by other pandemics or other major global events, Covid-19 is likely to cause a serious rethink about the robustness of industry structures, the advantages of shortening supply chains and sourcing closer to home to increase resilience to future uncertainties. 43
Academic/professional life
Every aspect of the world today – even politics and international relations – is affected by chemistry.”
Linus Pauling44 (1984)
To Pauling’s statement of the ubiquitous effects of chemistry on the world needs to be added the mirror image: everything in the world also impacts on chemistry. The practice of chemistry and the life of the professional chemist – whether in teaching, research or applied areas – take place within and are influenced by
global, national and local contexts that include competitiveness for position, resources, recognition and rewards, influenced by factors ranging from globalization to local politics and social attitudes.
Values in chemistry
The sciences share a fundamental set of values resting on the foundation that honesty is paramount. In recent years, evidence of falsifications within science and the dissemination of fake science, exacerbated by misinformation about Covid-19 during the pandemic, have caused growing concern. In the age of the internet and social media, there has also growing publicity concerning cases of bias and poor professional conduct. Examples have been seen across science, including in the field of chemistry, involving negative attitudes of individuals, arrogance, aggressiveness, bullying, discrimination, unrealistic expectations and failure to allocate due credit to collaborators. Moreover, there have been systemic failures by institutions in dealing effectively, objectively and transparently with complaints.
It is important that institutions (including academia, industry, funders and publishers) now take a firmer, proactive lead in developing systems that can help to create a safe, congenial, fair and open environment in which scientists can work. At the same time, it is important to ensure that there are spaces for a diversity of opinions to be aired, debated and considered and for everyone to become better educated in recognising the roots of inappropriate attitudes and actions and taking steps to correct them. As a leading discipline in science education, research and publishing, chemistry can play a major role in both improving its own performance and moving institutions in this direction.
Integrity, the scope of which encompasses personal attitudes and behaviour, ethical practices and research, is a key attribute that needs to be instilled in every scientist and its development should not be left to chance but achieved through training and incentives. Integrity in research must include not only the prevention of publication of fake science, but also earlier steps along the pathway in which hype and hypocrisy are increasingly prevalent and encouraged.45 Integrity is not just a principle, but a practice which requires skill development and support by systematic approaches. Much can be learned from the systems of Good Laboratory Practice (GLP) and Good Manufacturing Practice (GMP) that have become norms in industry, are prerequisites for the registration of pharmaceuticals and are already familiar to many chemists. Such systems can be adapted and incorporated, discipline-wide, as a general basis for all scientific research.
A major example of an area which illustrates the need for reform of inappropriate attitudes and behaviour and where the discipline of chemistry can make a difference concerns equity, diversity and inclusion (EDI). In all areas of life, including the world of science, the impact of Covid-19 has been especially felt by groups that have long been subjected to discriminatory practices and barriers to diversity and inclusion. In addressing the challenge of EDI, experience shows that it is important to go beyond efforts that rely on brief exposure to ‘sensitisation’ or ‘awareness raising’ or formulaic processes that count numbers of individuals in a particular group as evidence of ‘diversity’. Fundamental change requires training and the acquisition of skills and proficiency in ‘cultural competence’ to deal effectively with diversity and inclusion.46
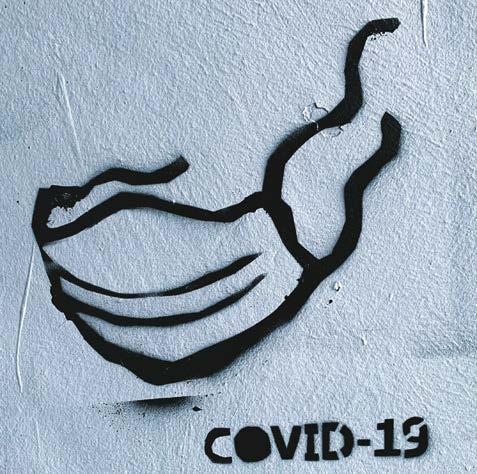
Conclusions
There can be no return to normal because normal was the problem in the first place”
— Graffiti in Hong Kong47
The extraordinary world-wide disruptions caused by the Covid-19 pandemic have caused a temporary halt or redesign in many professional activities, including in science and especially in experimental fields like chemistry where access to laboratories is required. There has been displacement of activities like teaching and conferencing into online, virtual channels. The pandemic has accelerated many movements and trends that were already in progress, forcing rapid decisions to be made and breaking down barriers and resistances to change.
As the central science that studies the metamorphosis of matter, chemistry offers a creative, exciting and rewarding career to professionals in academia and industry. And, as a science that also undergoes continuous change itself, chemistry is situated in the whirlwind of forces that is re-shaping the contemporary world. The discipline will undoubtedly be affected in major ways, both in the short and long terms, by Covid-19. Chemists should see this not just as a threat, but as an opportunity to seize the initiative, to take advantage of the increased fluidity of the times to drive the ongoing changes in the discipline towards better outcomes for science, for society and for the planet. ◆
Acknowledgments
We acknowledge support from the International Organization for Chemical Sciences in Development, which received funding during 2020 from the Gesellschaft Deutscher Chemiker, the Royal Society of Chemistry and Syngenta India. IOCD is hosted at the University of Namur, Belgium.
References
1. A. Roy. The Pandemic Is a Portal, In: A. Roy, Azadi: Freedom. Fascism. Fiction.
Haymarket Books, Chicago, 2020, ISBN: 9781642592603. https://www.haymarketbooks.org/books/1547-azadi 2. E. Svoboda. How the COVID-19 pandemic will change the way we live. Discover 17 April 2020. https://www.discovermagazine.com/health/how-the-covid-19-pandemicwill-change-the-way-we-live 3. COVID-19 coronavirus pandemic. 2020 Worldometer. https://www.worldometers.info/coronavirus/ 4. A. Guterres. The world of work cannot and should not look the same after this crisis. Launch of policy brief on COVID-19 and the world of work. United Nations,
New York, 18 June 2020. https://www.un.org/en/coronavirus/world-work-cannotand-should-not-look-same-after-crisis 5. M. Holford, R. Morgan. 4 ways science should transform after COVID-19, World
Economic Forum, 17 June 2020. https://www.weforum.org/agenda/2020/06/4-waysscience-needs-to-change-after-covid-19-coronavirus/ 6. COVID-19 Impact on international higher education: Studies and forecasts.
Deutscher Akademischer Austauschdienst, 22 July 2020. https://www.daad.de/ en/information-services-for-higher-education-institutions/centre-of-competence/ covid-19-impact-on-international-higher-education-studies-and-forecasts/ 7. A. Witze. Universities will never be the same after the coronavirus crisis. Nature, 2020, 582, 162-164,doi: 10.1038/d41586-020-01518-y. https://www.nature.com/articles/d41586-020-01518-y 8. M. Joshi. Who will be the winners in a post-pandemic economy? World Economic
Forum, 20 April 2020. https://www.weforum.org/agenda/2020/04/post-pandemiceconomy-favour-fastest-movers/ 9. A. Kekulé, Lehrbuch der Organischen Chemie oder der Chemie der
Kohlenstoffverbindungen , Band 1 und Band 2, Verlag Ferdinand Enke,
Erlangen 1859–1866. (Quotation translated from German by H. Hopf.) http:// www.worldcat.org/title/lehrbuch-der-organischen-chemie-oder-der-chemie-derkohlenstoffverbindungen/oclc/20926002 10. H. Sevian, V.A. Talanquer. Rethinking chemistry: a learning progression on chemical thinking. Chem Ed Res Practice 2014, 15, 10-23, doi: 10.1039/ c3rp00111c. 11. Mahaffy, P., Bucat, B., Tasker, R., Kotz, J., Treichel, P., Weaver, G., & McMurry, J. (2015) Chemistry: Human Activity – Chemical Reactivity, 2nd Canadian and 2nd
International Editions (Integrated package of introductory university Chemistry textbook and interactive digital learning resources), Nelson/Cengage. 12. Eilks, I., Hofstein, A. (Eds.) Relevant Chemistry Education: From Theory to
Practice. Springer AG, 2015, doi: 10.1007/978-94-6300-175-5. 13. P.G. Mahaffy, S.A. Matlin. Next hundred years: Systems thinking to educate about the molecular basis of sustainability. L’Actualité Chimique, December 2019, 446,47-49. https://www.lactualitechimique.org/Pour-les-cent-ans-a-venir-reflexionssur-l-enseignement-de-la-chimie-et-la-durabilite 14. Matlin, S.A., Mehta, G.,Hopf, H., Krief, A. One-World chemistry and systems thinking. Nature Chemistry 2016, 8, 393−396, doi: 10.1038/nchem.2498. 15. Wiek, A., Withycombe, L., Redman, C.L. Key competencies in sustainability: a reference framework for academic program development. Sustain. Sci. 2011, 6, 203–218, doi: 10.1007/s11625-011-0132-6. 16. K. B. Aubrecht, Y. J. Dori, T. A. Holme, R. Lavi, S.A. Matlin, M. Orgill, H.
Skaza-Acosta. Graphical tools for conceptualizing systems thinking in chemistry education. J. Chem. Educ. 2019, 96, 2888-2900, doi: 10.1021/acs. jchemed.9b00314. 17. S.A. Matlin, H. Hopf, G. Mehta, A. Krief. Repositioning the chemical sciences for a sustainable future. Chimie Nouvelle 2018, 128, 17-27. http://chimienouvelle.be/CN128/Article%20Krief.pdf 18. D.J. C. Constable, C. Jiménez-González, S.A. Matlin. Navigating complexity using systems thinking in chemistry, with implications for chemistry education. J Chem
Educ 2019, 96, 2689-2699, doi: 10.1021/acs.jchemed.9b00368. https://pubs.acs.org/doi/10.1021/acs.jchemed.9b00368 19. Kümmerer K, Clark JH, Zuin VG (2020) Rethinking chemistry for a circular economy. Science 367(6476):369-370, doi: 0.1126/science.aba4979. 20. Professional Master in Sustainable Chemistry (M.Sc.). Leuphana University,
Lüneburg, Germany 2020. https://www.leuphana.de/en/professional-school/mastersstudies/sustainable-chemistry.html 21. M.-H. Chiu, C.-C. Chou, Y.-H. Chen, T. Hung, W.-T. Tang, J.-W. Hsu, H.L. Liaw,
M.-K. Tsai. Model-based learning about structures and properties of chemical elements and compounds via the use of augmented realities. Chem. Teacher
Internat. 2018, 1(1), 1-10, doi: 10.1515/cti-2018-0002. 22. V. Talanquer, R. Bucat, R. Tasker, P.G. Mahaffy. Lessons from a Pandemic:
Educating for Complexity, Change, Uncertainty, Vulnerability, and Resilience. J.
Chem. Educ. 2020, doi: 10.1021/acs.jchemed.0c00627. https://dx.doi.org/10.1021/acs.jchemed.0c00627 23. J. Marcet. Conversations on chemistry: in which the elements of that science are familiarly explained and illustrated by experiments, and 38 engravings on wood.
Longman, London, 1st Edn. 1806. https://libquotes.com/jane-marcet/quote/lbi8o6g 24. National Research Council (US) Committee on Challenges for the Chemical
Sciences in the 21st Century. Beyond the Molecular Frontier: Challenges for
Chemistry and Chemical Engineering. Washington (DC): National Academies
Press (US); 2003, Chapter 3: Synthesis and manufacturing: creating and exploiting new substances and new transformations. https://www.ncbi.nlm.nih.gov/books/NBK207669/ 25. What is human security? 2020 United Nations Trust Fund for Human Security.
United Nations, New York. https://www.un.org/humansecurity/what-is-human-security/ 26. United Nations. 2016 Human Security Handbook 2016 United Nations, New York. https://www.un.org/humansecurity/wp-content/uploads/2017/10/h2.pdf 27. Steffen, W , Richardson, K. Rockström J, Cornell SE, Fetzer I, Bennett EM, Biggs
R, Carpenter SR, de Vries W, de Wit CA, Folke C, Gerten D, Heinke J, Mace GM,
Persson LM, Ramanathan V, Reyers B, Sörlin S. 2015 Planetary boundaries:
Guiding human development on a changing planet. Science 347, 6223, 736−747. (doi: 10.1126/science.1259855) 28. W. Steffen, W. Broadgate, L. Deutsch, O. Gaffney, C. Ludwig, The trajectory of the Anthropocene: The great acceleration. The Anthropocene Review 2015, 2, 81-98, doi: 10.1177/2053019614564785. 29. S.A. Matlin, G. Mehta, A. Krief, H. Hopf. The chemical sciences and health:
Strengthening synergies at a vital interface. ACS Omega 2017, 6819-6821, doi: 10.1021/acsomega.7b01463. 30. S.A. Matlin, T. Evans, J. Hasler, C. IJsselmuiden, O. Pannenborg, O.I. Touré.
Signposts to research for health. The Lancet 2008, 372 (9649), 1521-1522, doi: 10.1016/S0140-6736(08)61630-X. 31. Kümmerer K. Sustainable chemistry: A future guiding principle. Angew Chem
Internat. Edn. 2017, 56, 16420 –16421 doi: 10.1002/anie.20170994. 32. Matlin, S.A. Mehta, G. Hopf, H, Krief. A. The periodic table of the chemical elements and sustainable development. Eur. J. Inorg. Chem. 2019, 39-40, 4170–4173, doi: 10.1002/ejic.201801409. 33. Matlin, S.A. Hopf, H., Krief, A., Mehta, G., 2019. Ending the time of waste: Clean up, catch up, smarten up. Angle Journal, published online 1 November. http://anglejournal.com/article/2019-11-ending-the-time-of-waste-clean-up-catch-upsmarten-up/ 34. S.A. Matlin, G. Mehta, H. Hopf, A. Krief, Lisa Keßler, K. Kümmerer. Material circularity and the role of the chemical sciences as a key enabler of a sustainable post-trash age. Sustainable Chemistry and Pharmacy 2020, in the press. 35. C. Bettenhausen. IBM debuts chemical synthesis robot. Chem. & Eng. News 2020, 98(34), https://cen.acs.org/business/informatics/IBM-debuts-chemical-synthesisrobot/98/i34?utm_source=Newsletter&utm_medium=Newsletter&utm_campaign=CEN 36. P.J. Kitson, G. Marie, J.-P. Francoia, S.S. Zalesskiy, R.C. Sigerson, J.S.
Mathieson, L. Cronin. Digitization of multistep organic synthesis in reactionware for on-demand pharmaceuticals. Science 2018, 359, 314-319, doi: 10.1126/ science.aao3466. 37. A. Milo. The art of organic synthesis in the age of automation. Israel J. Chem. 2018, 52, 131-135, doi: 10.1002/ijch.201700148. 38. World Economic and Social Survey 2013: Sustainable Development
Challenges. UN Department of Economic and Social Affairs, New York, eISBN 978-92-1-056082-5, 2013. https://sustainabledevelopment.un.org/content/ documents/2843WESS2013.pdf 39. Stern N. 2019 Sustainability and Internationalism: Driving Development in the 21st Century. London: Grantham Research Institute on Climate Change and the
Environment and Centre for Climate Change Economics and Policy, London
School of Economics and Political Science. http://www.lse.ac.uk/GranthamInstitute/ publication/sustainability-and-internationalism-driving-development-in-the-21st-century/ 40. Mallapaty S. 2018 Paper authorship goes hype. Nature 30 January 2018. https://www.natureindex.com/news-blog/paper-authorship-goes-hyper 41. C. De Savi, D.L. Hughes, L. Kvaerno. Quest for a COVID-19 cure by repurposing small-molecule drugs: mechanism of action, clinical development, synthesis at scale, and outlook for supply. Org Process Res Dev. 2020 Jun 2:acs. oprd.0c00233, doi: 10.1021/acs.oprd.0c00233. 42. AstraZeneca takes next steps towards broad and equitable access to Oxford
University’s potential COVID-19 vaccine. AstraZeneca, Cambridge, 4 June 2020. https://www.astrazeneca.com/media-centre/articles/2020/astrazeneca-takes-nextsteps-towards-broad-and-equitable-access-to-oxford-universitys-potential-covid-19vaccine.html 43. Consolidation, risk reduction and demand shift: Potential strategic implications of the Corona virus for the global chemical industry. CHEManager 21 April 2020. https://www.chemanager-online.com/en/topics/strategy/consolidation-risk-reductionand-demand-shift 44. L.C. Pauling. Chemistry and the World of Tomorrow. Chem. Eng. News 1984, 62, 16, 54–56. doi: 10.1021/cen-v062n016.p054 https://pubs.acs.org/doi/abs/10.1021/ cen-v062n016.p054 45. H. Hopf, S.A. Matlin, G. Mehta, A. Krief. Blocking the hype-hypocrisy-falsificationfakery pathway is needed to safeguard science. Angewandte Chemie
International Edition 2020, 59, 2150-2154, doi: 10.1002/anie.201911889. 46. S.A. Matlin, V. W. W. Yam, G. Mehta, A. Krief, H. Hopf. The need for cultural competence in science: A practical approach to enhancing equality, diversity and inclusion. Angewandte Chemie International Edition 2019, 58(10), 2912-2913, doi: 10.1002/anie.201900057. 47. Wintour P. 2020 Coronavirus: who will be winners and losers in new world order?
The Guardian 11 April 2020. www.theguardian.com/world/2020/apr/11/coronaviruswho-will-be-winners-and-losers-in-new-world-order
by Thomas H Spurling and John M Webb
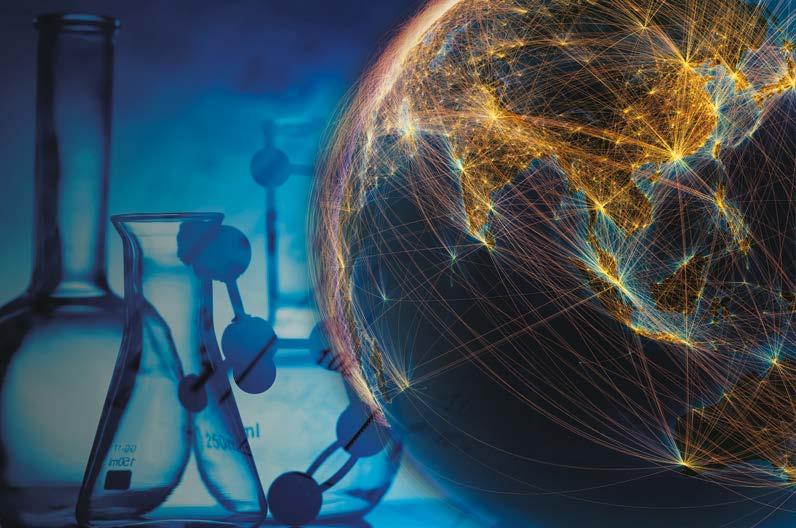
https://doi.org/10.51167/acm00014
Thomas H Spurling
Tom Spurling received his PhD in Physical Chemistry from the University of Western Australia in 1966. He joined CSIRO in 1969 and led its Chemistry research from 1989 to 1998. In 1999 he led the World Bank ‘Management and Systems Strengthening-LIPI’ project in Jakarta. He is now Professor of Innovation Studies at Swinburne University of Technology. Tom was President of the FACS from 1989 to 1991. He was made a Member of the Order of Australia in 2008 ‘For service to chemical science through contributions to national innovation policies, strategies and research, and to the development of professional scientific relationships within the Asian region.’ John M Webb
John Webb is a graduate in chemistry from the University of Sydney (B.Sc.) and from the California Institute of Technology (Ph.D.). He coordinated chemistry networks in Asia for many years for UNESCO, for the FACS and for the Australian government aid program. His subsequent diplomatic roles included UNESCO Paris and as Counsellor (Education, Science and Training) at the Australian High Commission in New Delhi with responsibilities that included Nepal and Pakistan. He now is Adjunct Professor at Swinburne University of Technology. In 1996 he was awarded the Medal of the Order of Australia for establishing collaborative research networks in Asia In the 1970s UNESCO and many national aid agencies understood the important role that the application of chemistry had in developing the social, economic and environmental wellbeing of nations UNESCO also understood the vital role that professional societies play in fostering chemical capability and helped organise the formation of the Federation of Asian Chemical Societies (FACS)
In 1980 our region contributed only 19% of world chemistry papers. The task of the FACS was, through networks, working groups and collaboration, to foster the development of chemistry in the region. This was highly successful. In 2018 our region contributed nearly 60% of all the world’s chemistry papers. We are the epicentre of world chemistry. However, chemical capability is still low in many of our member countries. The FACS now has two tasks: catering for the high performing chemists in the region while still fostering the development of chemistry in those countries still developing.1
Forty years on
We want to take your minds back to the late 1970s and early 1980s and recall the place that Asian chemistry had in comparison with the rest of the world. In those days, despite having more that 50% of the world’s population the region contributed only 19% of the chemistry papers in the Web of Science database. Only three countries, Japan (12.7%), India (4.8%) and Australia (1.7%) contributed more than 1% to the world output (See Table 1).
At that time UNESCO and many national aid agencies were very conscious of the important role that the application of chemistry had in developing the social, economic and environmental wellbeing of nations. As we have noted elsewhere3, in 2015 the United Nations General Assembly Resolution 70/1 set 17 Sustainable Development Goals for 2030 and at least eight of these goals require chemistry capability for their achievement.
It was during the 1970s that the Chemistry section in UNESCO was developing from its school science origins to include higher education and research. It was fortunate for our region that the person in the UNESCO Headquarters in Paris responsible for this development was Dr John Kingston, an Irish-born chemist with degrees from Trinity College, Dublin and the University of New South Wales, Sydney.
Research and training were the central themes of the program with an emphasis on capacity building and the development of endogenous capabilities. UNESCO provided small grants for activities such as workshops and training courses as well as contacts in the region. Projects were to be local and the benefits of that research were to be locally based.
Australia 1.7 Nepal <0.1 Bangladesh <0.1 New Zealand 0.3 China Mainland 0.1 Philippines <0.1 Hong Kong 0.1 Singapore <0.1 India 4.8 South Korea <0.1
Indonesia <0.1 Sri Lanka <0.1
Iraq 0.1 Taiwan 0.13
Japan 12.7 Thailand <0.1
Malaysia 0.1 Table 1. Chemistry research publications in 1980 (as % of global research publications) of FACS member countries; data from the Web of Science database.2
To achieve this, Kingston mobilised chemists in other countries as collaborators.4
His Australian experience led him to readily engage chemists in many countries in establishing and delivering programs through regional networks. The first such network, established in 1974, was the regional network for the chemistry of natural products in South East Asia. This research theme was an inspired choice, since the chemistry of extracts from plant species peculiar to a country’s biodiversity provided unique opportunities for local chemists to select and control their research.
Over time, the regional network approach was extended to other regions such as South Asia and other areas of chemistry such as inorganic and analytical chemistry and, in time, training courses for instrumentation.
The success of the network approach prompted the UNESCO Division of Scientific Education and Research to propose, in 1978, that a Federation of Asian Chemical Societies be established. The model was the already established Federation of European Chemical Societies, widely seen as being valuable in the construction of the post-World War II scientific environment within Europe. UNESCO thought of Asia as all those parts of the world that weren’t Europe, Africa or the Americas! This definition included countries from Iran to New Zealand and from Australia to Japan. An information notice was sent to the national chemical societies of the region in February 1978 asking them to prepare to present their ideas at a meeting to be arranged later in the year. By October 1978 UNESCO had responses from Australia, Hong Kong, Indonesia, India, Iraq, Japan, Korea, New Zealand, Malaysia, Philippines, Singapore, Sri Lanka and Thailand. UNESCO arranged for a working group meeting to be held in Bangkok in December 1978, and this meeting agreed on draft statutes to be presented at an inaugural function in August Number Sustainable Development Goal 2 Zero Hunger
3 Good Health and Well-Being
6 Clean Water and Sanitation
7 Affordable and Clean Energy
9 Industry, Innovation and Infrastructure
13 Climate Action
14 Life Below Water
15 Life on Land
Table 2. Sustainable Development Goals requiring chemical capability
of 1979 at Mahidol University, Bangkok. A glittering ceremony, attended by Her Royal Highness Princess Chulabhorn of Thailand and other dignitaries, opened the inaugural meeting. The following eleven societies agreed to be founding members of the Federation of Asian Chemical Societies: Royal Australian Chemical Institute, Hong Kong Chemical Society, Indian Chemical Society, Iraqi Chemical Society, Korean Chemical Society, Malaysian Chemical Society, Integrated Chemists of the Philippines, Institute of Chemistry Ceylon, Singapore National Institute of Chemistry, Indonesian Chemical Society and the Chemistry Section, Science Society of Thailand (replaced by the Chemical Society of Thailand in 1981). Of the missing two of the original thirteen respondents to the UNESCO invitation, the Chemical Society of Japan and the New Zealand Institute of Chemistry joined the Federation in 1981.
The original statutes included many details and qualifications needed to persuade the foundation members that the new Federation was not going to threaten their autonomy. The objectives were: 1. The Federation is a voluntary association, the object of which is to promote co-operation in Asia, Australia and New
Zealand between non-profit-making learned societies in the field of chemistry whose membership consists of individual qualified chemists. With a view to promote the advancement of chemistry. It seeks to cooperate with regional and international organisations, and to act as a channel of communication for such organisations and to avoid overlap with them on projects initiated by itself or by them. 2. A fundamental principle governing the work of the Federation is that nothing done by or in the name of the Federation shall detract from the autonomy of any of the participating societies.
This was replaced in 1991 by a simpler statement:
The general objective of the Federation is to promote the advancement of chemistry and the interests of professional chemists in the Asia Pacific region in a way which does not detract from the autonomy of any of the member societies.
And in 2019
The general objective of the Federation is to promote the advancement and appreciation of chemistry and the interests of professional chemists in the Asia Pacific.
The FACS web site6 lists 31 member societies in late 2019, with member societies covering most of east and south-east Asia but less across west Asia. The chemical societies of central Asia as well as Iran are not yet members of FACS. Some of the listed member societies are mostly inactive in FACS matters such as those from Iraq and Mongolia. There is clearly some way to go to achieve a fully inclusive FACS that extends across Asia.
An examination of Table 1 of the situation in 1980 indicates that in these early days there was considerable scope for the ‘advancement of chemistry’. Many member society countries accounted for less than 0.1% of the global production of research papers in the chemical sciences. But much was to change.
During the past 40 years, the economies of many, but not all, countries in Asia
GDP since 1980 for FACS countries in the G20
12
10
8
rilli o n s T 6
4
2
0
19 8 0 19 8 3 19 8 6
China India Turkey 19 8 9 19 9 2 19 9 5 19 9 8 20 0 1 20 0 4 20 0 7 20 1 0 20 1 3
20 1 6 Australia Korea, Rep. Indonesia Japan
Figure1. GDP since 1980 for FACS countries in the G20
GDP since 1980 for FACS countries not in the G20
1.40
1.20
1.00
0.80
0.60
0.40
0.20
0.00
1980 1985 1990 1995 2000 2005 2010 2015
Malaysia Bangladesh Hong Kong SAR, China Iraq Nepal Philippines Sri Lanka New Zealand Singapore Thailand
Cambodia Brunei
Pakistan Vietnam Jordan Mongolia Saudi Arabia Fiji Israel Kuwait Papua New Guinea Taiwan
Figure 2. GDP since 1980 for FACS countries not in the G20
GDP of countries in our region with growth potential
0.09
0.08
0.07
0.06
0.05
0.04
0.03
0.02
0.01
0.00
1980 1985 1990 1995 2000 2005 2010 2015
Myanmar Timor-Leste Bhutan Lao PDR Cambodia
Figure 3. GDP of countries in our region with growth potential have grown strongly. When the FACS commenced in 1980 only Japan and Australia were in the top 20 economies of the world. In 2018 China, Japan, India, Korea, Australia, Indonesia and Turkey are all in the top twenty countries by nominal GDP with Taiwan just outside the top 20 economies. We have illustrated this in the following figures7. In these figures we have used data from the World Bank and have expressed the figures as constant 2010 US dollars. We would have preferred to use purchasing power parity to compare national GDPs but that World Bank series for many countries only goes back to 1990.
In Figure 1 we have displayed the GDP of the seven countries of the FACS who are members of the G20 group of nations. Note the growth of China and the emergence of India.
In Figure 2 we have displayed the GDPs of the FACS countries that are not in the G20 group of nations. The GDP data for Taiwan is from a different source from the other countries8. Taiwan has a GDP which is marginally lower than the 20th of the G20 nations. When comparing this Figure 2 with Figure 1, note the different scale on the y-axis.
While we can all marvel at the extraordinary economic growth in our region in the past 40 years, we should not forget that some of the countries represented in our Federation have not yet participated in this growth. This is illustrated in both Figure 2 and in Figure 3. In Figure 3 we have included Myanmar, Lao and Bhutan, countries that are not yet members of the FACS. TimorLeste is our newest member.
As noted earlier, in 1980 the region covered by the Federation contributed only 19% of the world’s output of chemistry Country
China
India
Indonesia
Pakistan
Bangladesh
Japan
Philippines
Vietnam
Turkey
Thailand
South Korea
Iraq
Malaysia
Nepal
Australia
Taiwan
Sri Lanka
Israel
Hong Kong
Singapore
New Zealand
Total
% of world population
18.5
17.7
3.5
2.8
2.1
1.6
1.4
1.25
1.07
0.9
0.66
0.51
0.41
0.36
0.32
0.31
0.27
0.11
0.095
0.075
0.062
54.00
Table 4. Percent of world population of FACS countries.
Australia 2.1 Nepal < 0.1
Bangladesh 0.1 New Zealand 0.2
China Mainland 32.9 Philippines < 0.1
Hong Kong 0.7 Singapore 0.9
India 7.0 South Korea 4.0
Indonesia 0.1 Sri Lanka < 0.1
Iraq 0.1 Taiwan 1.4
Israel 0.6 Thailand 0.5
Japan 5.7 Turkey 1.2
Malaysia 0.7 Vietnam 0.3
Table 3. Chemistry research publications in 2018 (as % of global research publications) of FACS member countries; data from the Web of Science database.10 papers. The situation in 2019 is quite different from that of 40 years ago. Our region now produces about 56% of the world output. (see Table 3) Curiously, this is about the same as the percent of the world who live in this region!9 (see Table 4) The global scientific output in chemistry has continued to grow across this period but the contributing countries have changed appreciably. Some key examples are shown in Figure 4: in 2018 China contributed close to 35% of world chemistry literature in the data base; the USA has dropped down to around 15%. Such data are sometimes contested in terms of the relative importance of these publications. Citation data, however, confirm that much of the chemistry research literature from Asia is well cited. In Table 5 we have listed the Category Normalized Citation Impact (CNCI) for the chemistry papers from selected countries (see Table 5).
40.0%
35.0%
30.0%
25.0%
20.0%
15.0%
10.0%
5.0%
AUSTRALIA
CHINA MAINLAND
GERMANY (FED REP GER) INDIA
ISRAEL
JAPAN
SINGAPORE
SOUTH KOREA
UNITED KINGDOM
USA
0.0%
Figure 4. Global chemistry papers: contribution of selected countries.
Category normalised citation impact 2018 Australia 1.26 China 1.28 Germany 1.1 India .85 Israel 1.01 Japan 0.8 Singapore 1.89 South Korea 0.97 Taiwan 0.87 UK 1.17 USA 1.26
Table 5. Impact measure of chemistry papers from selected countries.
The CNCI of a document is calculated by dividing the actual count of citing items by the expected citation rate for documents with the same document type, year of publication and subject area.
In the 40 years of the Federation’s life our region has become the epicentre of world chemistry! In this new global configuration of chemistry, the Federation has a unique opportunity. This has some implications for the program of the FACS.
In this new global chemical milieu, the Federation has to cater for the needs of the high performing countries. This means: • Conferences such as the ACC • Attracting more IUPAC specialist conferences to our countries • Promoting one or more high impact journals • Consider sponsoring its own specialist conferences
The Federation also needs to foster chemistry in the countries that have not yet reached their potential. Here, we refer to countries in the Federation such as Cambodia, Mongolia, Papua New Guinea and Timor-Leste as well as those not yet in the Federation. We have noted above the skewed geographic distribution of member countries and recognise the need to consider expanding the Federation to include other potential member countries within Asia such as Iran, Uzbekistan, Kazakhstan, Myanmar, Bhutan and Laos. We show in Figure 5 the GDP data for the countries in West Asia who are not members of the FACS. They have a lot to offer!
Finally, we recall the important role of the Federation in stimulating regional cooperation to assist countries to develop their capacity in chemistry and chemical education. The chemical society of the newest country in Asia, Timor-Leste, has applied to be a member of the Federation. The authors of this paper are familiar with the situation regarding chemical education and chemical research in Timor-Leste. The secondary school system has a modern curriculum based on the Portuguese curriculum but the schools, both public and private) lack even the most basic laboratory facilities and equipment. The same is true of the only public university in Timor-Leste, the National University of Timor-Leste (UNTL) in Dili.
We should remember that in the 1970s and 1980s various country’s aid programs contributed significantly to the building of chemical capacity in the region. UNTL urgently needs a general-purpose science laboratory with equipment to enable them to teach chemistry, physics and biology. Can the FACS be an agent to develop an aid program to achieve this?
We would like to thank Adam Finch for assistance with the bibliographic data and Helen Wolff for her help in preparing the GDP figures. ◆
GDP for countries in West Asia
0.6
0.5
0.4
rilli o n s T 0.3
0.2
0.1
0
1980 1987 1994 2001 2008 2015
Afghanistan Iran, Islamic Rep. Uzbekistan Kazakhstan Syrian Arab Republic Turkmenistan Tajikistan Kyrgyz Republic
Figure 5. GDP for countries in West Asia
References
1. A version of this paper was published in Chemistry in Australia. Thomas H Spurling and John M Webb,
Chemistry in Australia, July-August 2020, pp 20-23 2. Clarivate Analytics, Web of Science, https://clarivate.com/products/web-of-science/ 3. Samuel Freitas, John Webb and Thomas Spurling, The Role of Chemistry and Chemical Education in Achieving the Sustainable Development Goals, Understanding Timor-Leste: 2019 TLSA Research
Conference, Dili, June 27, 2019 4. M. Mohinder Singh, The Federation of Asian Chemical Societies; Its formation, administration and activities (A historical review), FACS Newsletter No1, 1988, 3-7. 5. Barry N Noller, T H Spurling and M Mohinder Singh, FACS and its 25th Anniversary, A review of its progress 1987-2004, FACS Newsletter Special 2004, pp24-41 6. http://www.facs-as.org/ accessed 20 November 2019 7. https://data.worldbank.org/indicator/NY.GDP.MKTP.KD accessed 20 November 2019 8. https://en.wikipedia.org/wiki/List_of_countries_by_past_and_projected_GDP_(PPP), accessed 20 November 2019 9. https://www.worldometers.info/world-population/population-by-country/ accessed 22 November 2019 10. Clarivate Analytics, Web of Science, https://clarivate.com/products/web-of-science/