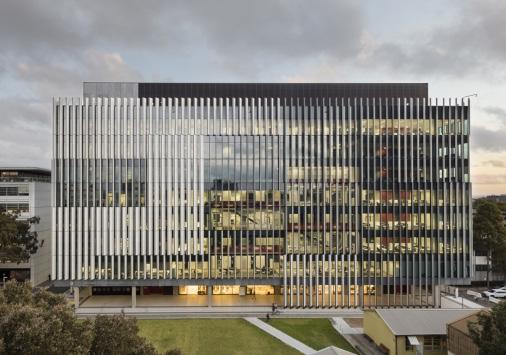
18 minute read
UNSW Sydney
Source: Sally Wood
Out With the Old: UNSW’s Research Delivers for the Future
For 70 years, UNSW has delivered on its promise of teaching programs with a strong emphasis on societal impact.
UNSW is at the forefront of materials science and engineering, which is critical as the world turns towards sustainable practices, fuelled by our desire to protect the planet and future generations.
The School of Materials Science and Engineering is one piece of the puzzle seeking to fill knowledge gaps and sustain Australia’s pipeline of science practitioners.
The School was founded in 1952, in the same year the atom bomb was developed by Britain, and the world witnessed the first successful use of a mechanical heart in the United States.
It was also the dawn of the technology and information age, where silicon microchips changed the way in which people connect, conduct business, and access data.
These microchips, made from semiconductors, brought forward a new era of speed and accuracy. It also transformed computers from room- sized giants to the devices we have become familiar with.
Today, the School of Materials Science and Engineering offers research programs for postgraduate students based on four societal themes of impact:
1. Energy and Environment: covering structural and functional materials that help produce, store and covert energy, as well as sustainable materials and modern recycling technologies.
2. Biomedical and Health: covering materials designed to assist biological systems for therapeutic and diagnostic medical purposes.
3. Transport and Infrastructure: focussing on structural materials designed with the future of transportation and large-scale infrastructure projects in mind.
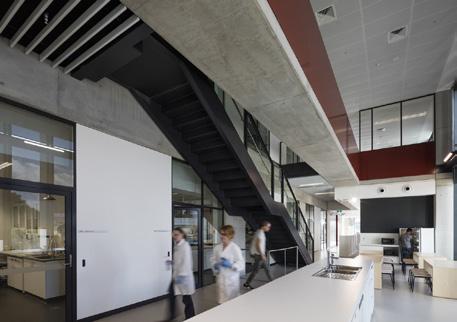
4. Electronics and Communications: developing materials for electrical, electronic and microelectronic applications.
Associate Professor Judy Hart is the Deputy Director of Education, who said the School of Materials Science and Engineering provides students with clear pathways to success.
“We try to make sure that we’re giving students the skills they will need to be able to make their own contributions to the materials field and to society in general.”
It is a formula that seems to be working. Last year, UNSW was recognised as the university with the most employable students by the Australian Financial Review. A UNSW education means graduates are prepared to continue their work at organisations like CSIRO, ANSTO, and Cochlear.
Bracing For Impact
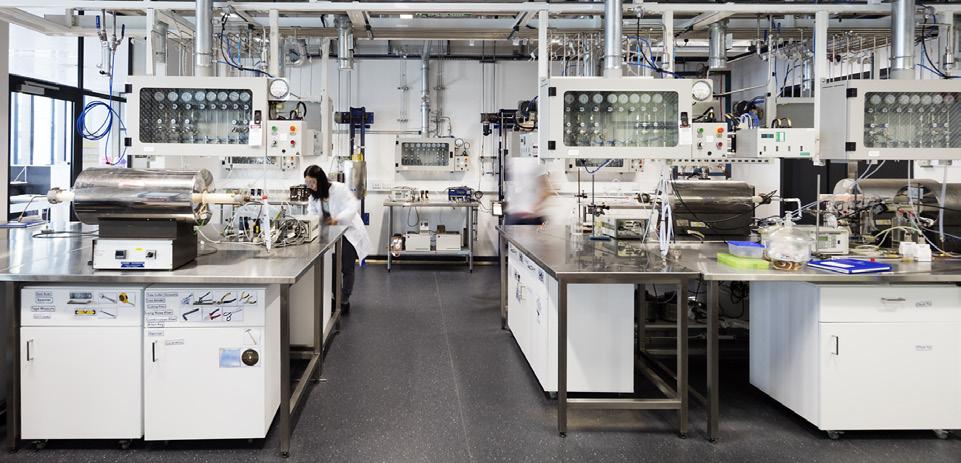
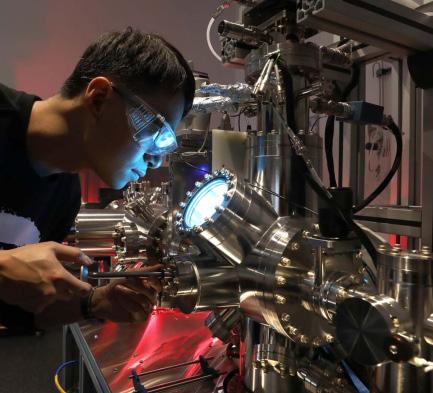
UNSW is not complacent when it comes to delivering impact. Scientists remain focussed in their quest of attracting the brightest minds, and research utilisation across the four societal themes.
In the energy and environment stream, researchers are developing novel hydrogen storage and battery technologies. Hydrogen is a fuel that will play a central role in our future, but challenges remain bringing it to life.
Scientists are pushing through these barriers by working on ways to improve the cost and efficiency of hydrogen fuel cells like platinum. In one study, researchers developed a technique to test the durability and stability of platinum alternatives.
Biomedical and health researchers have their sights set on the development of organic bioelectronic devices. This will create flexible devices with tuneable performances for vulnerable people.
Similarly, engineers in the transport and infrastructure program have worked on the development of high temperature materials that are resistant to corrosion. This will protect the longevity and security of materials across the aerospace, environmental and manufacturing industries. These scientists are the only group conducting this vital research In Australia.
Meanwhile, electronics and communications researchers are learning about nanomaterials, thin films and hybrid heterostructures to power the next chapter in technology innovation.
Listening to Atoms Moving at the Nanoscale
International collaboration is key to the work conducted at UNSW. As researchers seek to solve common problems, it makes sense to join forces with likeminded peers.
Scientists from UNSW Sydney recently partnered with the University of Cambridge to listen to the sounds of atoms moving under pressure.
This phenomenon, known as ‘crackling noise,’ occurs in avalanches. This noise can be observed every day, from crumpling paper and candy wrapping, to the crackling of cereal, as well as in natural occurrences, such as earthquakes.
It was discovered more than 100 years ago by listening to the change of magnetisation in magnets.
However, Professor Jan Seidel and his lab from the School of Material Science and Engineering have taken this further—recording the crackling noise of just a few hundred atoms.
“It is the movement of atoms in materials under external force that generates noise, like a creaking door, as a macroscopic example,” Professor Seidel said. The experiments, which were conducted overnight and lasted over eight hours, provide valuable insights for a range of different fields, from mining to medicine.
“This means that studying atoms in the lab this way could lead to new information about crackling noise in different contexts.”
Professor Seidel took on the challenge of developing a new method to observe crackling noise at the smallest scale.
“We wanted to record the crackling noise of the selected materials, not the crackling of other external elements.”
“We tested this on lots of materials and under slightly different conditions,” he said.
Together, the team were able to record the crackling noise of approximately 30 nanometre deep areas.
Professor Seidel said the noise can be measured at small length scales, which may lead to potential new applications.
“We can now look at different types of nanoscale features in materials and study how they crack or how they deform and that might enable the development of completely new technology. “This is the exciting bit about
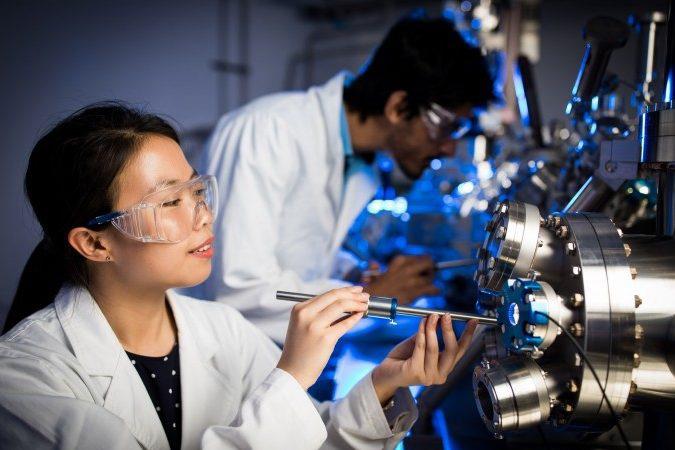
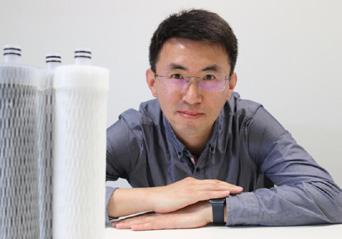
This Device Could Help Clean the World’s Drinking Water, One Filter Cartridge at a Time
A newly-developed water filtration device is rolling off the production line thanks to Australian research.
Deakin University scientists developed the cost-effective and sustainable water filter cartridge by using a cutting-edge composite membrane material.
The device can be integrated into water bottles, water purifiers, filters and coffee machines.
Manufacturers are looking for ways to improve filtration of chemicals and bacteria, while reducing their environmental footprint.
Water can contain dirt, minerals, chemicals, bacteria and mould, impacting smell, taste and potentially endanger health, especially when microscopic organisms and bacteria grow and cause serious illness.
Consequently, the need to improve water quality and ensure the safety of drinking water is becoming increasingly urgent. While most water filters on the market remove bacteria, viruses, spores, and cysts through nanofiltration or reverse osmosis membrane technology; those methods require high water pressure and result in a low flow rate.
However, lead researcher Dr Quanxiang Li said the new device efficiently intercepts bacteria and viruses in drinking water using the principle of charge adsorption.
“The current production process for positively charged filters is complex and expensive, and their performance is limited.”
“That’s why I’ve conducted a great deal of research on the preparation of new and more effective material for water filtration and signed a long-term cooperative project with industry partner, the Runner Group’s Xiamen Filtertech Industrial Corporation, to bring it to life,” he said.
The device has begun rolling off the production line in Xiamen, China, and maintains high performance without
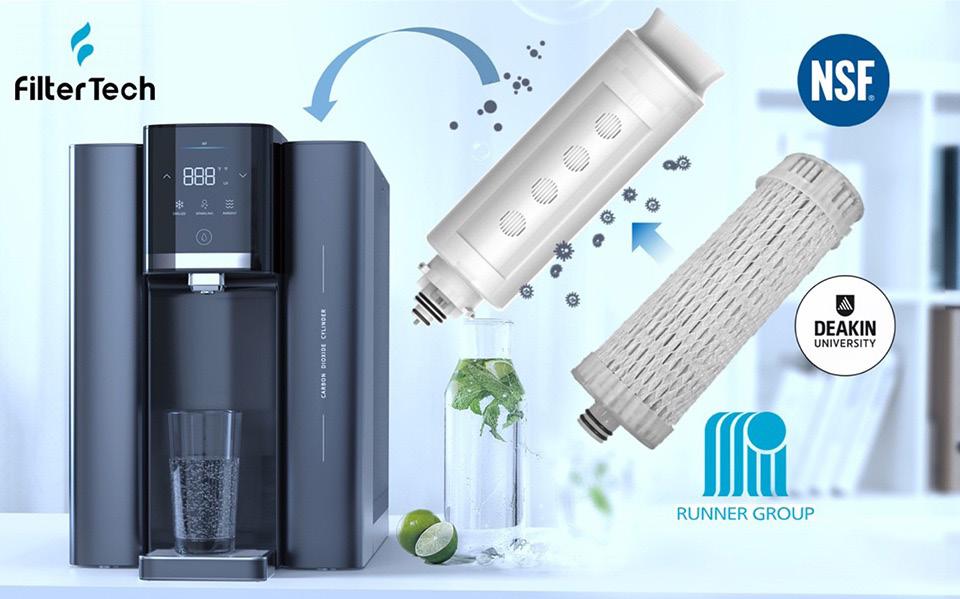

Schematic of the proposed mechanism of the ML-niosome treatment. 1) ML-niosomes bind to the surface polysaccharides removing some of the outer layers, 2) Polymyxin B can more freely reach the surface of the outer membrane. 3) Polymyxin and ML-niosomes then breakdown both the outer and inner membranes. Reproduced under Creative commons license CC BY 4.0
Using Antibacterial Lipids in Nanoparticles
Combined with Established Treatment Shows Promise Against Antibiotic
Resistant Bacteria
A large Australian team led by Monash University has devised an approach to killing antibiotic-resistant bacteria. Researchers used lipid nanoparticles that target specific layers on the surface of the bacterial cell.
It shows antibacterial lipids can be successfully used in combination with nanocarrier lipids to form nanoparticles that kill gram-negative bacteria.
Instrument scientist and co-author Dr Anton Le Brun contributed to the research with measurements on the neutron reflectometer Platypus and analysis of the data.
“Neutron reflectometry is a useful tool for understanding the structure of cell membranes at the nanometre length scale.”
The Platypus instrument at the Australian Centre for Neutron Scattering was used to elucidate the mechanism at work in a combined ML-niosome/polymyxin B treatment at the molecular level.
Niosomes are vesicles with special properties that are used to deliver drugs.
Meanwhile, polymyxin B is an antibiotic of last resort for treating infections from gram-negative bacteria. Some bacteria have started to show signs of resistance even to this antibiotic.
By making artificial membranes that mimic the properties of the gram-negative bacterial cell surface, the team discovered the ML-niosomes target the outer layer of the outer membrane, which is mainly composed of polysaccharides. The binding of the ML-niosomes to the surface of the outer membrane exposes the membrane.
This grants polymyxin B better access to attack and breakdown the protective outer membrane and then the inner membrane, which ultimately kills the bacterial cell.
Future work will investigate how this is achieved in detail at the molecular level and why the combination with polymyxin B is more effective.
Researchers “Bioprint” Living Brain Cell Networks in the Lab
Monash University researchers have successfully used ‘bioinks’ containing living nerve cells to print 3D nerve networks.
These networks can grow in the laboratory and transmit and respond to nerve signals.
The research team used a tissue engineering approach, and bioprinting with two bioinks containing living cells and noncell materials respectively.
Through this, they were able to mimic the arrangement of grey matter and white matter seen in the brain.
Professor John Forsythe is from the Department of Materials Science and Engineering, who is leading the research.
He said while two-dimensional nerve cell cultures have previously been used to study the formation of nerve networks and disease mechanisms, those relatively flat structures do not reflect the way neurons grow and interact with their surroundings.
“The networks grown in this research closely replicated the 3D nature of circuits in a living brain, where nerve cells extend processes called neurites to form connections between different layers of the cortex.”
“We found that the projections growing from neurons in the printed ‘grey matter’ or cellular layer readily grew through the ‘white matter’ layer and used it as a ‘highway’ to communicate with neurons in other layers,” Professor Forsythe said.
Bioprinted 3D neural networks are likely to be a promising platform for studying how nerves and their networks form and grow, investigating how some diseases affect neurotransmission, and screening drugs for their effects on nerve cells and the nervous system.
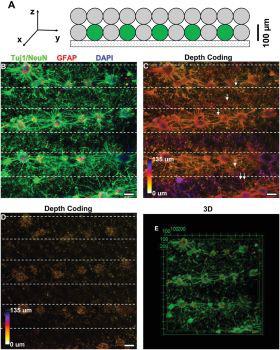
The study, entitled ‘3D Functional Neuronal Networks in Free-Standing Bioprinted Hydrogel Constructs’, was recently published in Advanced Healthcare Materials.
Bioprinted cortical neurons and astrocytes after in vitro culture at 7DIV. A) Cross-section view of bioprinted structure consisting of cellular (green) and acellular (grey) strands. B) Maximum intensity projection of patterned structure: cellular–acellular–cellular. Neurons (NeuN, green), astrocytes (GFAP, red), and nuclei (DAPI, blue) were colocalized and developed complex structures. Axons (Tuj1, green) originating from the proximal cellular strands projected across the distal cellular strand.
C) Depth-coding of Tuj1/NeuN showed the development of axonal projections across strands. White arrows indicate neurites that belong to different focal planes. D) Depth-coding of DAPI shows localization of cell nuclei. E) Confocal image in 3D. Depth-coding was represented using a color bar: red, closest to the glass substrate, and blue, 135 µm away from the substrate. Scale bar, 100 µm.
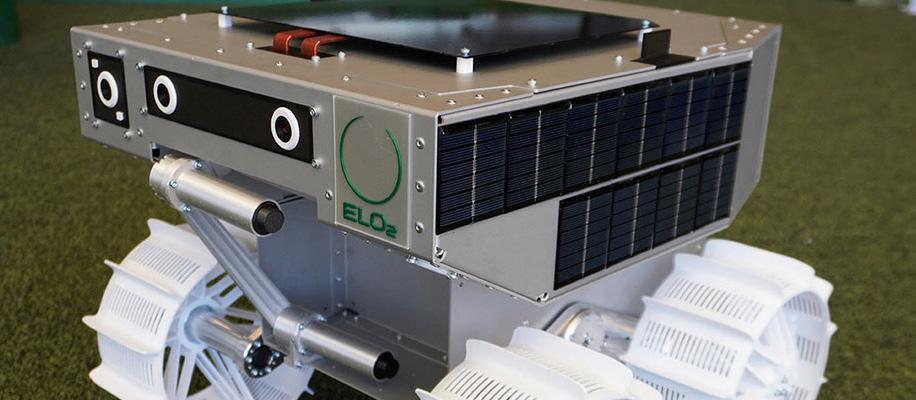
Australia’s first lunar rover. Image credit: RMIT University.
Shooting For the Moon with Australia’s First Rover
Australia’s first lunar rover is on the horizon, as RMIT University researchers unveil their first prototype.
RMIT is playing a key role in the Australian Space Agency’s Moon to Mars Trailblazer program, which aims to land the rover on the Moon as part of a future NASA Artemis mission later this decade.
If chosen, the rover will be tasked with transporting lunar regolith, also known as Moon soil, to a NASA-run facility for the extraction of oxygen.
It will autonomously navigate the lunar environment, and locate and collect regolith. It is an essential element to enable NASA’s in-situ resource utilisation facility to operate.
Once complete, oxygen will be extracted from the regolith, which can be used for Artemis astronauts to breathe and for spacecraft fuel.
Engineering A Rover Designed for Space
RMIT University’s Space Industry Hub is supporting the technical design, optimisation and precision manufacturing of the rover.
Professor Ray Kirby, Dean of RMIT’s School of Engineering, said technology will be crucial to launching Australia’s first lunar mission.
“We are excited to help drive technology to support sustainable human life on the Moon and, in doing so, to be part of an accelerating Australian aerospace industry.”
“The ELO2 consortium have unveiled a rover prototype that demonstrates our world-leading skills in engineering and manufacturing robotic technologies, remote operations and autonomous decision-making systems,” he said.
RMIT University is one of 16 partners in ELO2, an experienced consortium that brings Australia’s top talent and expertise across space research and industry.
Dancing Delicacies: Combining Food and Tech for Interactive Dining
Food is able ‘dance’ across platters because of a recent study from Monash University.
Researchers looked at the design of food as a material in which computer programs can be enacted.
The program has offered playful and interactive culinary opportunities for diners and chefs.
Food interaction design researcher and lead author Jialin Deng created a system encompassing a plate fitted with electrodes, which can be programmed to move different food elements like sauces and condiments around on their own.
Ms Deng said the project was about exploring the integration of food’s material properties and ‘computational’ capabilities, and achieving different dining journeys.
“For example, a chef can predefine the locations where they want to put the food droplets and ingredients, and they can programme the dish frame by frame, like you do in animation.”
“We can put solid items and watery items together, we can merge two different flavours, we can transport various things towards the plate, we can play with chemical or physical reactions like in molecular gastronomy,” Ms Deng said.
Interaction, game and play design expert from the Faculty of IT’s Creative Technologies discipline group and co-author of the research, Professor Florian ‘Floyd’ Mueller, said the research is a glimpse into the future of food and computing.
“This will not only change the hospitality industry, who can create much more engaging experiences by being able to tell new and different stories through interactive food, but also computer science education, where students learn about computing by eating food.”

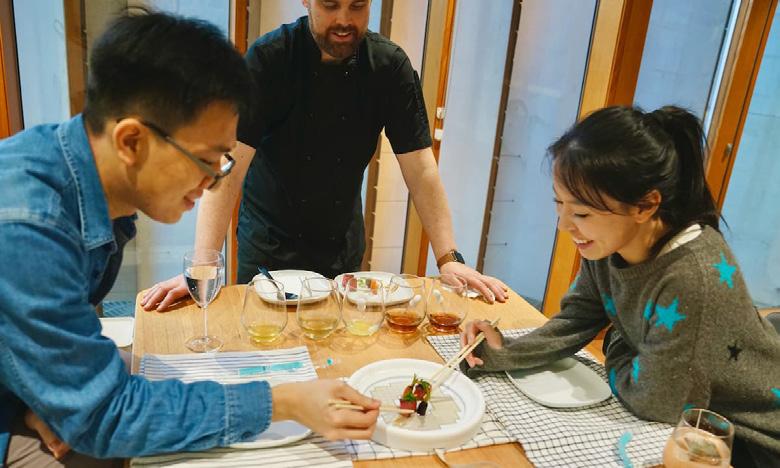
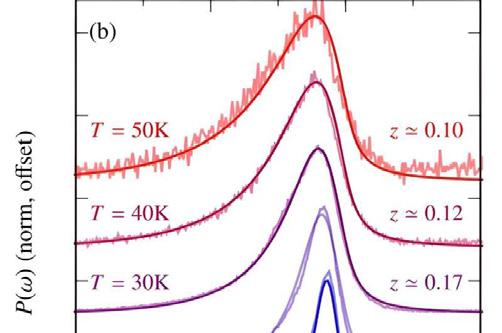
The new technique shows remarkably good agreement with experimental results, essentially perfect at high temperature, with small discrepancies at lower temperatures. Comparison of theoretical (solid dark) and experimental (solid light) photoluminescence spectra at different lattice temperatures. Credit: FLEET
Solving Quantum Mysteries: New Insights into 2D Semiconductor Physics
Researchers from Monash University have unlocked fresh insights into the behaviour of quantum impurities within materials.
The international theoretical study introduces a novel approach known as the ‘quantum virial expansion.’
It offers a powerful tool to uncover the complex quantum interactions in two-dimensional semiconductors.
The breakthrough holds potential to reshape our understanding of complex quantum systems and unlock exciting future applications using novel 2D materials.
The study of quantum impurities has far-reaching applications across physics in systems as diverse as electrons in a crystal lattice to protons in neutron stars.
These impurities can collectively form new quasiparticles with modified properties, but their associated problems are difficult to solve.
"The challenge lies in accurately describing the modified properties of the new quasiparticles," said Dr. Brendan Mulkerin, who led the collaboration with researchers in Spain. However, researchers introduced the ‘quantum virial expansion’ (QVE), a powerful method that has long been indispensable in ultracold quantum gases.
QVE’s integration into the study of quantum impurities meant only the interactions between pairs of quantum particles needed to be considered (rather than large numbers of them).
The innovative approach is remarkably effective at high temperatures, and low doping, where the electrons' thermal wavelength is smaller than their interparticle spacing.
"One of the most intriguing aspects of this research is its potential to unify different theoretical models, with the ongoing debate surrounding the appropriate model for explaining the optical response of 2D semiconductors being resolved through the quantum virial expansion," said corresponding author Jesper Levinsen
The research is published in the journal Physical Review Letters.
2023 Malcolm McIntosh Prize for Physical Scientist of the Year
The Australian National University’s Professor Yuerui (Larry) Lu recently received the 2023 Malcolm McIntosh Prize for Physical Scientist of the Year.
Professor Lu was recognised for discovering interlayer exciton pairs, which can help to unravel the phenomenon of superfluidity.
His research brings to life a passion for understanding and unlocking new areas of science.
“When I was a child, I was an eager explorer, often taking apart toys to unlock their mysteries,” he said.
His recent discovery is paving the way for new electronic devices, which are more energy efficient and faster.
“My team made the first experimental discovery of interlayer exciton pairs.”
“An interlayer exciton is made by a positive charge and a negative charge sitting in two different layers. Two interlayer excitons combine together to form an interlayer excision pair,” he said.
Professor Lu’s research team also made the world’s thinnest micro-lens, only 1/2000th the thickness of a human hair.
This can be used to make lightweight optical systems, opening possibilities for space exploration, medical imaging, environmental monitoring and food safety.
“Teamwork and collaboration are crucial for my research. The diversity of scientists and disciplines in my team leads to more robust, inclusive and impactful advancements for science, Professor Lu said.
He has made a significant contribution to educating and developing the next generation of nanoscience and nanotechnology researchers.
Professor Lu said it was an honour to receive the recognition.
“This prize will continue to inspire me and young scientists to think big and address challenges for the future.”


Novel Approach to Advanced Electronics, Data Storage with Ferroelectricity
Australian researchers recently worked on switchable polarisation in a new class of silicon compatible metal oxides.
The research paves the way for the development of advanced devices including high-density data storage, ultra-low energy electronics, and flexible energy harvesting and wearable devices.
Flinders University and UNSW Sydney led the study, which provides the first observation of nanoscale intrinsic ferroelectricity in magnesium-substituted zinc oxide thin films.
Ferroelectrics are similar to magnets, which exhibit a corresponding electrical property known as permanent electric polarisation.
This phenomenon stems from electric dipoles featuring equal but oppositely charged ends or poles.
Corresponding author Dr Pankaj Sharma is from Flinders University, who said the research offers fresh insights into this stream of science.
“The research findings offer significant insights into the switchable polarisation in a new class of much simpler siliconcompatible metal oxides with wurtzite crystal structures and lay a foundation for the development of advanced devices.”
The polarisation can be altered between two or more equivalent states or directions when subjected to an external electric field.
As such, the switchable polar materials are under active consideration for numerous technological applications including fast nano-electronic computer memory and lowenergy electronic devices.
Historically, this technologically important property has been found to exist in complex perovskite oxides that incorporate a range of transition metal cations leading to diverse physical phenomena such as multiferroicity, magnetism, or even superconductivity.
However, the research points to a potential solution, which avoids the stringent processing requirements.
The research was recently published in the American Chemical Society ACS Nano journal.
Brothers Produce Excellent Scientific Results with Improvements to Silicon
Two brothers, who work in unrelated disciplines have combined their expertise to tackle a chemical problem.
Dr Tamim Darwish suggested to his brother, Dr Nadim Darwish—a Senior Lecturer with expertise in molecular electronics at Curtin University—that deuterating silicon might improve its properties.
Dr Tamim Darwish is familiar with the unique properties of deuterium, an isotope of hydrogen used to replace hydrogen in molecules.
The pair worked at the National Deuteration Facility (NDF) at ANSTO, which is a world leader in deuteration for research applications.
The pair reported enhancements to the properties of silicon when hydrogen was replaced with deuterium on the surface layer.
In recent years, there has been significant interest in a technology that combines silicon and organic molecules for various applications such as sensors, solar cells, power generation, and molecular electronic devices.
The challenge with this technology has been the surfaces made of silicon and hydrogen (Si H surfaces), which are crucial for building these devices, are prone to oxidation. This oxidation can harm the stability of the devices both mechanically and electronically.
However, the Darwish brothers found if hydrogen is replaced with deuterium, creating Si D surfaces, these surfaces become much more resistant to oxidation when exposed to either positive or negative voltages.
Si D surfaces demonstrated more stability against oxidation, and their electrical characteristics were more consistent compared to Si H surfaces.
The investigators recommended using Si D surfaces instead of Si H surfaces in applications that require non-oxidised silicon surfaces, such as electrochemical biosensors, silicon-based molecular electronic devices, and silicon-based triboelectric generators.

In situ cell construction. Reproduced under creative commons license CC BY 4.0 https://creativecommons.org/licenses/by/4.0/.
Preventing Catastrophic Failure in Lithium Ion Batteries
A team of ANSTO scientists recently used neutron scattering techniques to understand the formation of harmful lithium structures in rechargeable lithium ion batteries (LIBs).
Despite being found in most portable electronics and electric vehicles, the energy capacity of LIBs falls short of what is required by many next-generation technologies. Although replacing the common electrodes in these batteries with pure lithium metal can help the battery store more energy, lithium microstructures forming at the lithium surface can create short-circuits, and lead to catastrophic battery failure.
There are different types of structures in dismantled batteries:
• Whiskers: resembling tiny needles
• Moss: looking like a porous layer
• Dendrites: long, thin structures.
Professor Vanessa Peterson, who led the study, said the pointy nature of dendrites causes the most trouble. “We used small-angle and ultra-small-angle neutron scattering (SANS and USANS) techniques with our Quokka and Kookaburra instruments at the Australian Centre for Neutron Scattering to study these complex lithium structures.”
She explained these methods are insightful because they provide information about the size and shape of the lithium structures inside a battery without taking it apart. The investigators used SANS and USANS to provide a precise and less complicated way to analyse the structure of deposited lithium compared to other methods like X-ray imaging, microscopy, or gas adsorption.
“This research opens the door for future investigations to explore how factors like the amount of electric current, charging time, and the cyclic process of lithium deposition and dissolution impact the surface area and the distances between interfaces within deposited lithium,” Professor Peterson said.
Capturing Greenhouse Gases with the Help of Light
Slowing global warming relies on drastic cuts to greenhouse gas emissions.
However, researchers believe reducing emissions alone will not be enough to meet current climate targets.
In light of this, researchers at ETH Zurich have worked on a new method of carbon capture using light. This ensures energy required for carbon capture will come from the sun in the future.
Led by Professor Maria Lukatskaya, the scientists are exploiting acidic aqueous liquids, where CO2 is present as CO2, but in alkaline aqueous liquids, it reacts to form salts of carbonic acid, known as carbonates.
It means this chemical reaction is reversible. A liquid's acidity determines whether it contains CO2 or a carbonate. To influence the acidity of their liquid, researchers added molecules, called photoacids, to ensure it reacts to light.
If such liquid is then irradiated with light, the molecules make it acidic. In the dark, they return to the original state that makes the liquid more alkaline.
In practice, however, researchers encountered a problem: the photoacids used are unstable in water. As such, the research team analysed the decay of the molecule. They solved the problem by running their reaction in a mixture of water and an organic solvent.
The scientists were then able to determine the optimum ratio of the two liquids by laboratory experiments. In addition, they could explain their findings thanks to model calculations carried out by researchers from the Sorbonne University in Paris.
Their next step on the way to market maturity will be to further increase the stability of the photoacid molecules.
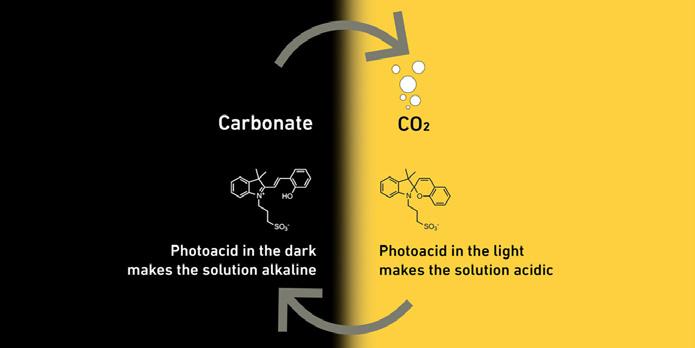
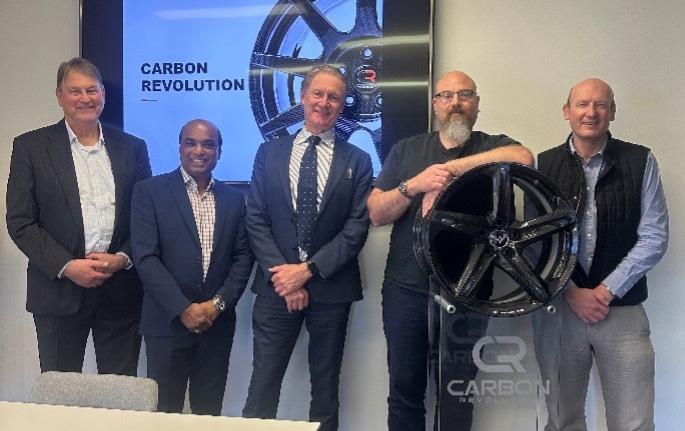
Carbon Revolution has partnered with the Institute for Frontier Materials (IFM) to revolutionise the production of carbon fibre wheels. Image credit: Deakin University.
Carbon Revolution Partnership to Revolutionise Carbon Fibre Wheels Production to Meet Demands
Carbon Revolution, a global technology company and Tier 1 OEM (original equipment manufacturer) supplier, has partnered with Deakin University’s Institute for Frontier Materials (IFM) to revolutionise the production of carbon fibre wheels.
This collaboration is part of the Australian Composites Manufacturing CRC project, which aims to make Australia a leader in composite technologies with an advanced and automated network of designers, manufacturers and service providers.
The CRC’s CEO, Dr Steve Gower praised Carbon Revolution’s capabilities in advanced composites manufacturing.
“Carbon Revolution provides a great example of Australian capabilities in advanced composites manufacturing. ACM CRC is proud to support our partners Carbon Revolution and Deakin University to develop product and process technologies to support the expansion of manufacturing capabilities for Carbon Revolution’s single-piece carbon fibre wheels.”
Carbon Revolution has already established itself as the recognised leader in the production of lightweight carbon fibre wheels for the global automotive industry. The company is a Tier 1 original equipment manufacturer supplier.
Through this research collaboration, Carbon Revolution has progressed from single prototypes to designing and manufacturing wheels at commercial scale for some of the most prestigious brands in the world.
With almost 80,000 Carbon Revolution wheels produced, the company has penetrated the performance and premium end of the automotive market with 18 awarded programs for Ford, Ferrari, General Motors, Jaguar Land Rover and Renault.
The wheels offer unsprung weight savings of up to 50% relative to the comparable aluminium wheels.
The CRC program brings industry and researchers together leveraging resources, expertise and funding to advance Australian business and Industry.
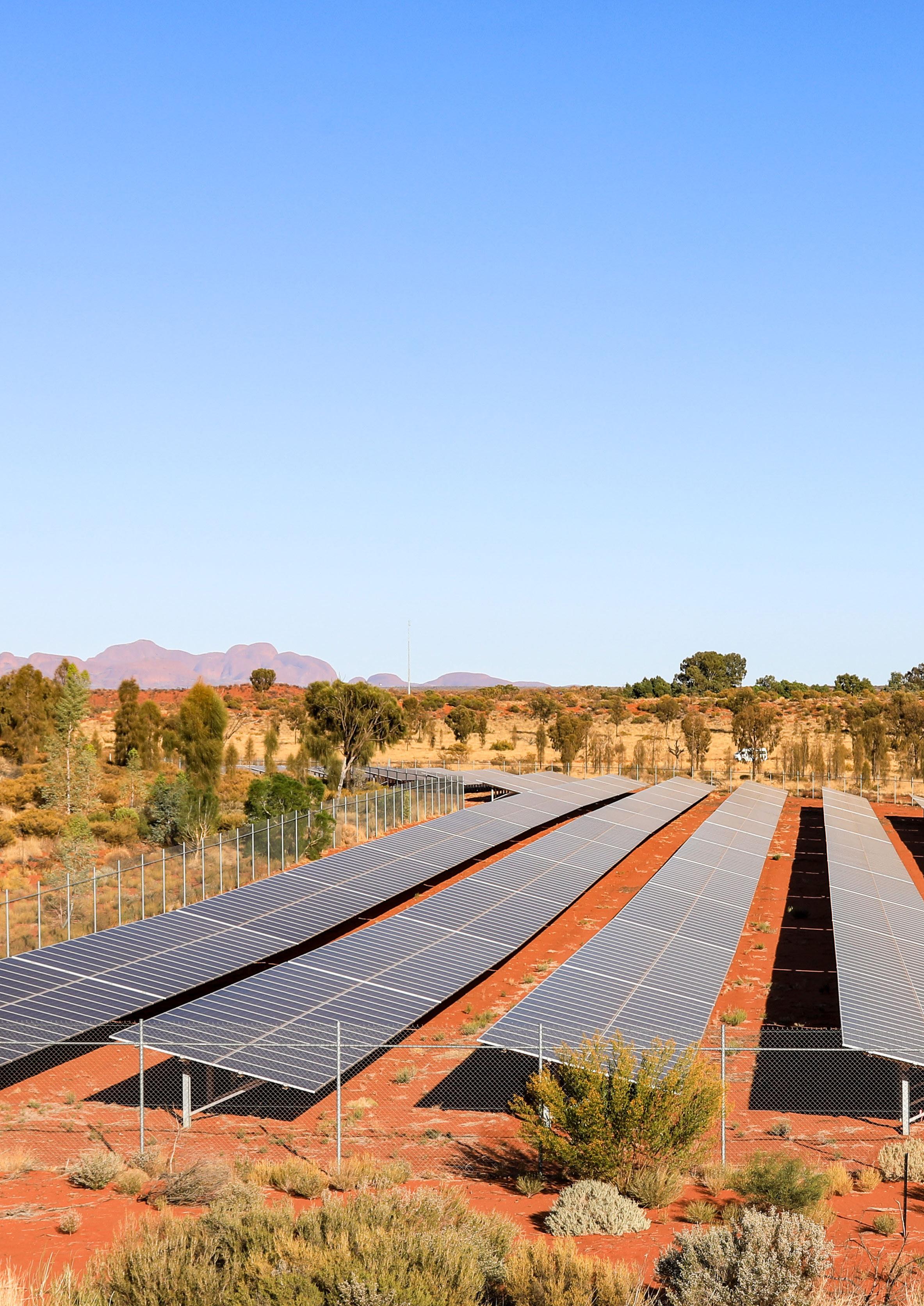