
18 minute read
BREAKING NEWS
Ultralight, Flexible Solar Cell Keeps Tech in Shape
An ultralight flexible solar cell, 10 times thinner than the width of a human hair, could hold the key to promising power sources for future wearable technology. An international research team, involving Monash University, has developed a super-thin photovoltaic cell that has high efficiency, supreme mechanical bending and stretching capacity, and capabilities to provide a long-lasting power source. Researchers successfully developed novel mechanically robust photo-absorber materials that can make ultra-flexible solar cells. These cells can achieve a power conversion efficiency of 13% with 97% efficiency retention after 1,000 bending cycles, and 89% efficiency retention after 1,000 stretching cycles. Tests have shown that when this solar cell was treated with a special method, its performance had only dropped 4.8% after a staggering 4,736 hours. It could run for more than 20,000 hours – about 2.5 years – with minimal degradation, and had an estimated shelf life of 11.5 years. At a size of just 2cm squared – roughly the same as an Australian 5c piece – this solar cell is light enough to be supported by a flower petal and can generate 9.9 watts of power per gram. With further testing, this revolutionary device could be used as a replacement for batteries in a number of future technologies such as mobile phones, watches, internet of things (IoT), and in biosensors. Despite its size, these low-cost solar cells can be reproduced easily with continuous printing technology, which makes them ideal for the fast tracking and mass production of wearable technology.
An international research team, involving Monash University, has created a flexible solar cell that could revolutionise future wearable technology. Millions of respirator and surgical masks will be tested at UniSA and Flinders University laboratories to help stop the spread of COVID-19.
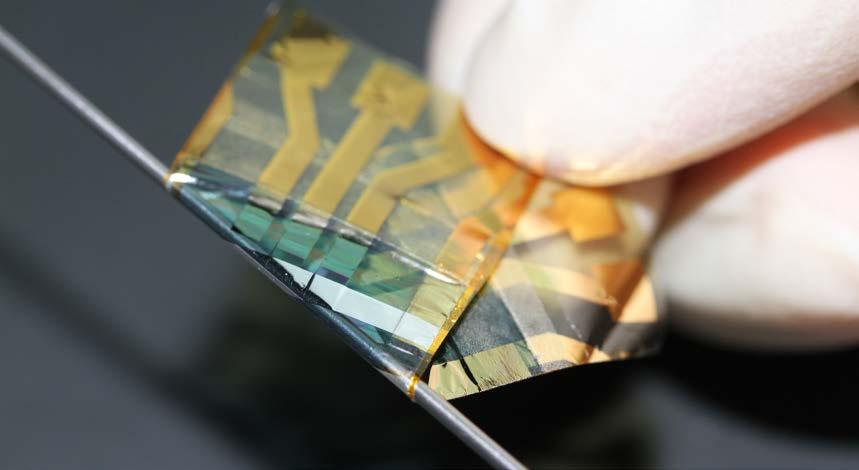
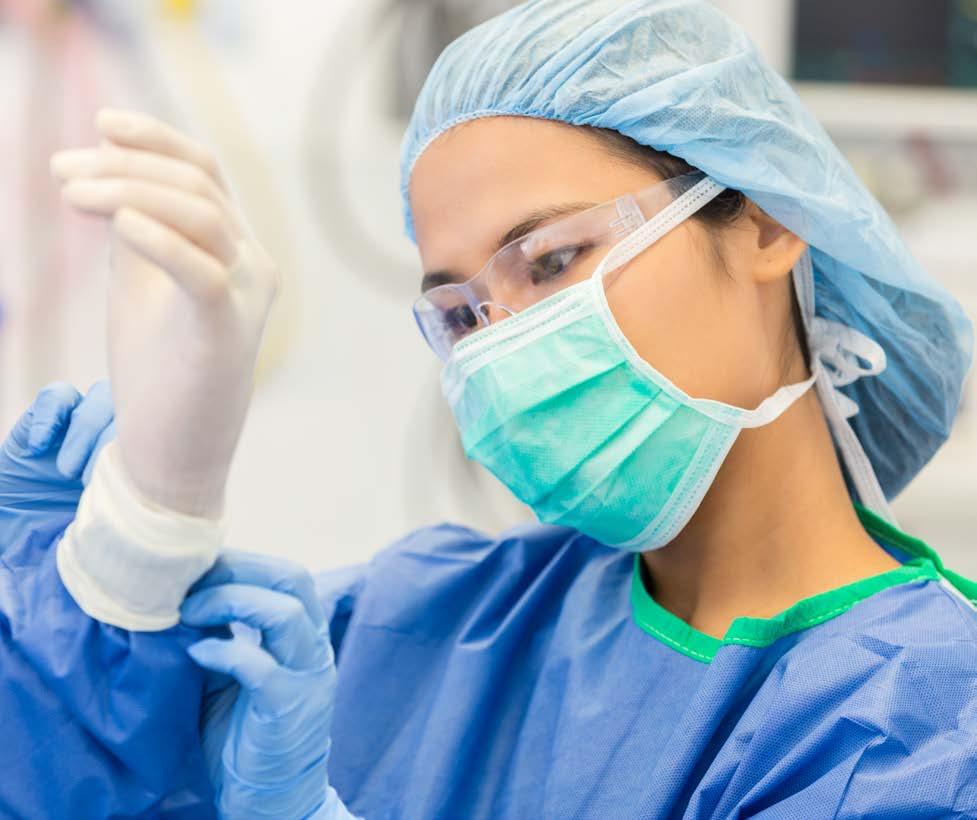
UniSA in Joint Project to Help Halt the Spread of COVID-19
In an Australian first, millions of respirator and surgical masks made by Adelaide packing company Detmold will be tested at the University of South Australia (UniSA) and Flinders University laboratories to help stop the spread of COVID-19. The South Australian State Government is allocating $450,000 for the project where more than 20 million masks will be produced and tested each month for local and national markets. Professor Emily Hilder, Director of UniSA’s Future Industries Institute at Mawson Lakes, where the testing will take place, said the University was excited to collaborate with Flinders University to support local testing of masks. “This both improves our capacity to respond to the immediate demands due to COVID-19 and also provides new opportunities that will support the long-term viability of manufacturing businesses in South Australia,” Professor Hilder said. South Australian Health Minister Wade said the testing facilities at the two universities would provide ongoing assurance to the public of the quality and safety of equipment being used in our hospitals. “I commend the initiative of the University of South Australia and Flinders University for the important role they are playing to improve the delivery of this vital protective medical equipment to Australian health workers,” said Minister Wade. Innovation and Skills Minister David Pisoni said the locally made medical equipment would start rolling off the production line in May. “In these unprecedented circumstances, science, research, innovation and collaboration have never been more important. With these new testing facilities at UniSA and Flinders University, we will be able to deliver this medical equipment to hospitals within weeks.”
BREAKING NEWS
UQ Ventilator Design Hackathon Puts Engineers to Work
A mission to design ventilators that could serve COVID-19 patients brought together 200 bright engineers and their students at The University of Queensland (UQ). The UQ Ventilator Design Hackathon tasked students and their mentors to design a variety of ventilator components that could be manufactured locally within an emergency time frame. UQ’s Professor Ross McAree said the work engaged students in the broader engineering effort to significantly enhance Queensland’s capability to respond to COVID-19-related ventilator shortages. “We believe that engineering is about working in a team on real-world challenges, not just performing well in an exam,” Professor McAree said. “This is a great opportunity for our students to work with industry professionals to make a lasting impact in the community.” Taking ventilator specifications set out by Australia’s Chief Scientist, Dr Alan Finkel, the design groups have worked with clinical advisor, Brisbane anaesthetist Dr Erich Schulz. Electrical engineering and business student, Rohit Nunna, said the intensive week-long effort involved working remotely and, for some, at the UQ Innovate makerspace. “It’s been a huge positive learning curve,” Nunna said. “I can now really appreciate the beauty of fundamental engineering principles and how they need to be applied to a complex problem like building a ventilator.” Professor McAree said the hackathon would help position Queensland for longer term engineering and manufacturing security around medical devices. “The best ventilator component designs from the hackathon will be further developed and prototyped for evaluation in Queensland hospitals,” he said.
Solving a Mystery in 126 Dimensions
One of the fundamental mysteries of chemistry has been solved by Australian scientists – and the result may have implications for future designs of solar cells, organic light-emitting diodes and other next-gen technologies. Benzene – which comprises six carbon atoms matched with six hydrogen atoms – is the smallest molecule that can be used in the production of opto-electronic materials, which are revolutionising renewable energy and telecommunications tech. It is also a component of DNA, proteins, wood and petroleum. The mystery around the structure of the molecule arises because although it has few atomic components it exists in a state comprising not just four dimensions – like our everyday ‘big’ world – but 126. Measuring a system that complex – and tiny – has until now proved impossible, meaning that the precise behaviour of benzene electrons could not be discovered. Now, however, scientists led by Timothy Schmidt from the ARC Centre of Excellence in Exciton Science and the University of New South Wales (UNSW) in Sydney have succeeded in unravelling the mystery. Professor Schmidt, with colleagues from UNSW and CSIRO’s Data61, applied a complex algorithm-based method called dynamic Voronoi Metropolis sampling (DVMS) to benzene molecules in order to map their wavefunctions across all 126 dimensions “What we found was very surprising,” said Professor Schmidt. “The electrons with what’s known as up-spin double bonded, while those with down-spin remained single.” “That wasn’t what we were expecting, but it could be good news for future technological applications. Essentially, it reduces the energy of the molecule, making it more stable, by getting electrons, which repel each other, out of each other’s way.”
L to R: Giordano Masnata, Isabelle Fleming, Mitchell Davis and Mitchell Heard. Picture by Mark Calleja.
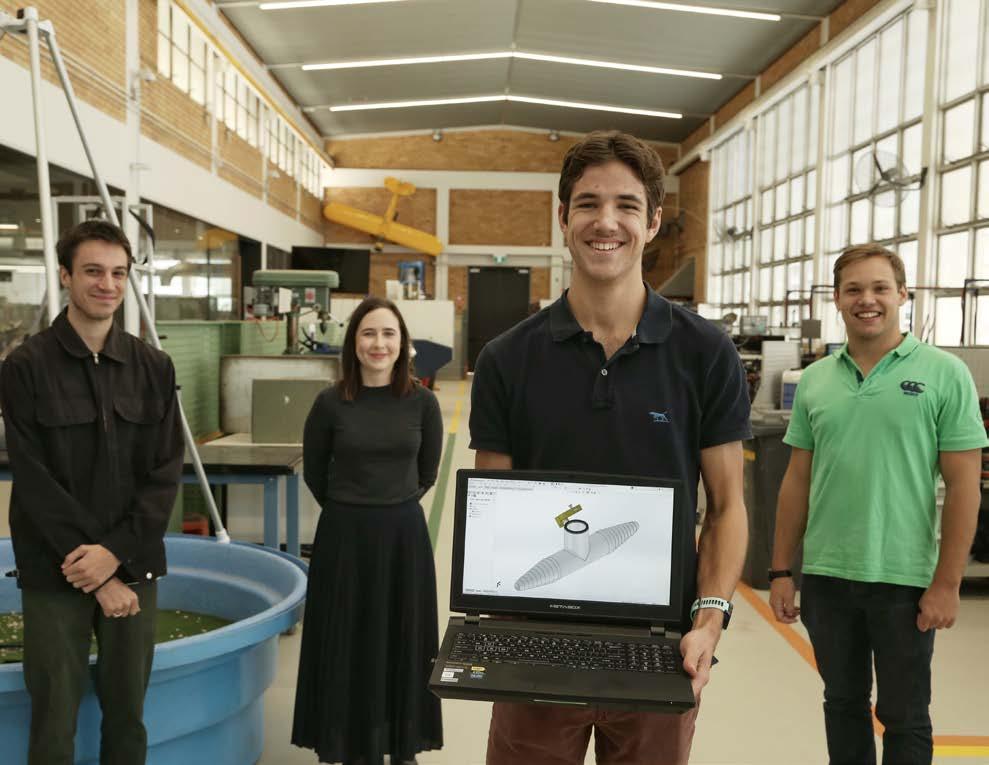
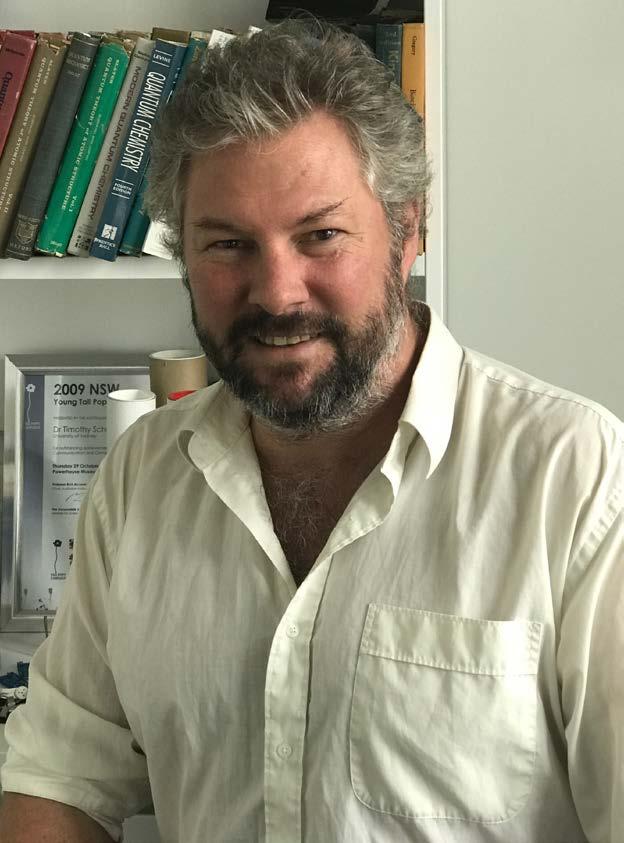
Professor Timothy Schmidt, unravelling the mystery of benzene. Credit Exciton Science.
BREAKING NEWS
Better Predictions of the Working Life of Industrial Components
A niggling disparity between an established mathematical model to predict creep crack growth behaviour in materials in high-temperature environments and actual data prompted Dr Warwick Payten to re-asses the approach and revise the model. Dr Payten is a senior research scientist in the nuclear fuel cycle group at ANSTO and one of Australia’s leading experts in determining the remaining operational life of materials in hightemperature environments. In research published in Engineering Fracture Mechanics, Payten shared a revised model to predict creep growth rates that was validated in a range of materials. “Being able to more accurately predict crack growth in real components is highly useful because it allows you to potentially extend the life of operating industrial plants and conventional, solar and nuclear power stations with confidence,” said Payten. “Engineers and those of us who work in fracture mechanics have been aware for many decades that the current equations developed in the 1980s by Nikbin Smith and Webster (NSW) are overly conservative,” Payten added. In the NSW equation to determine plane strain crack growth, which is used in all the engineering codes, you multiply the plane stress crack growth by a multiaxial factor of 30 or 50. This produces a high estimate for failure, or shorter operational life for the component. After testing and validating the new model a range of different materials, including carbon, steels, stainless steels, inconels and superalloys, the materials used to construct current and future power reactors, Payten said he was confident the model could be used almost universally. Payten recommends that the universal crack growth equations and the FEA code be changed to provide a more realistic prediction of component life.
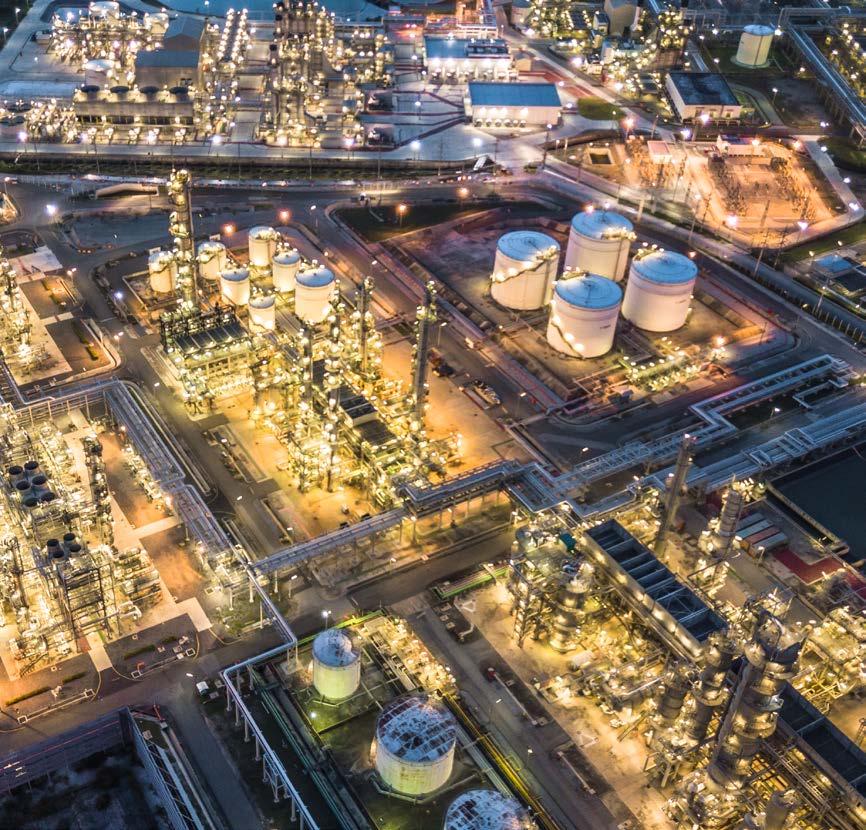
Griffith 3D Print Experts Create PPE for Frontline Workers

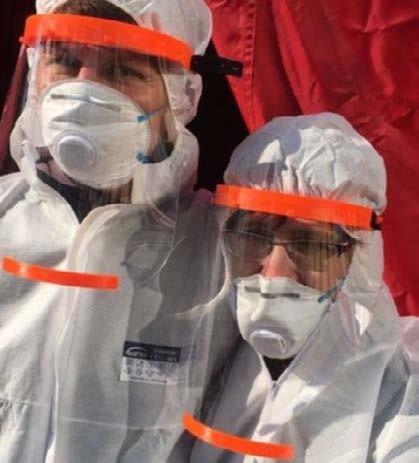
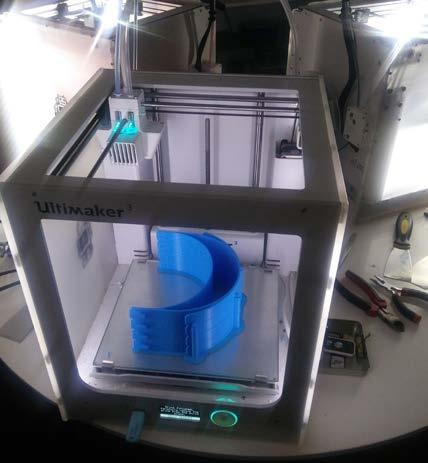
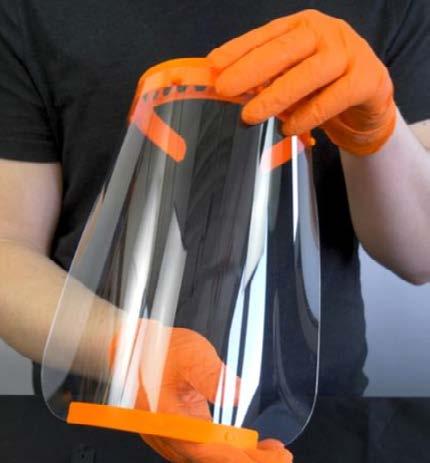
Griffith University’s expertise and 3D printers are being used to help manufacture essential protective equipment for frontline health workers across Australia. As part of a call-out by the Queensland State Government’s health authorities, hospital-grade designs were shared with volunteers with access to 3D printers. Face shields and other personal protective equipment (PPE) are being created using 3D printers from a number of facilities and schools across the university to help maintain supplies during the COVID-19 crisis. Griffith’s Advanced Design and Prototyping Technologies Institute (ADaPT) and Queensland College of Art (QCA) design studio are among the teams that have recently swung into action, after a request for help from Metro North Hospital and Health Service. Metro North operates five hospitals on Brisbane’s northside, including Royal Brisbane and Women’s Hospital and The Prince Charles Hospital. According to Adapt Manager Derek Smith, ADaPT was perfectly placed to respond. “ADaPT already works closely with the Gold Coast University Hospital on a range of projects, and we’ve been able to move fast to make PPE parts,” Smith said. “We’re leading the different disciplines across the university that are working on this project, from design and engineering to health. It is fantastic to see our community rising to the challenge.” QCA Technical Officers Chris Little and Alex Innocenti have been running several 3D printers around the clock, making hundreds of face shields. “We’re one part of a very big project worldwide, but together, we can help frontline staff in the fight against COVID-19,” Little said.
BREAKING NEWS
Seeking the Sounds of Superfluids at Swinburne
A Swinburne University of Technology study published recently examines the propagation of energy as sound waves in a quantum gas, revealing for the first time strong variations in the nature of the sound wave as a function of temperature. At low energies, this energy travels via the collective movement of many particles moving in sync – essentially, as sound waves – quantified using quasiparticles known as phonons. Below the superfluid transition temperature (Tc) these sound waves in a unitary Fermi gas can propagate without collisions and are driven by ripples in the phase of the superfluid order parameter (wave-function)—this mode is known as the Bogoliubov-Anderson (BA) phonon. Above Tc, the sound waves become more strongly-damped, and collisions play a dominant role: < Tc In the colder, superfluid mode, damping is dominated by collisions with thermally excited quasiparticles and is well described by (QRPA) theory. > Tc Above the transition temperature, the stronglydamped mode occurs at the crossover between collisionless hydrodynamic regimes. >> Tc At even higher temperatures, collective propagation of sound-wave vanishes, and excitation is dominated by the energy of individual particles.
Strong similarities were identified in the temperature dependence of sound in the unitary Fermi gas and the behaviour of phonons in liquid helium, which was one of the first superfluids identified historically. The study of many-body quantum systems with strong interparticle interactions is of great interest for the understanding of novel materials. The study also provides quantitative benchmarks for dynamical theories of strongly-correlated fermions.

Researchers at Swinburne’s Centre for Translational Atomaterials have developed a highly efficient solar absorbing film.

Graphene Solar Heating Film Offers New Opportunity for Efficient Thermal Energy Harvesting
Researchers at Swinburne’s Centre for Translational Atomaterials have developed a highly efficient solar absorbing film that absorbs sunlight with minimal heat loss and rapidly heats up to 83°C in an open environment. The graphene metamaterial film has great potential for use in solar thermal energy harvesting and conversion, thermophotovoltaics (directly converting heat to electricity), solar seawater desalination, wastewater treatment, light emitters and photodetectors. The researchers have developed a prototype to demonstrate the photo-thermal performance and thermal stability of the film. They have also proposed a scalable and low-cost manufacturing strategy to produce this graphene metamaterial film for practical applications. “In our previous work, we demonstrated a 90 nm graphene metamaterial heat-absorbing film,” said Professor Baohua Jia, founding Director of the Centre for Translational Atomaterials. “In this new work, we reduced the film thickness to 30 nm and improved the performance by minimising heat loss. This work forms an exciting pillar in our atomaterial research.” Lead author Dr Keng-Te Lin said, “Our cost-effective and scalable structured graphene metamaterial selective absorber is promising for energy harvesting and conversion applications. Using our film an impressive solar to vapour efficiency of 96.2% can be achieved, which is very competitive for clean water generation using renewable energy source.” Co-author Dr Han Lin added, “In addition to the long lifetime of the proposed graphene metamaterial, the solar-thermal performance is very stable under working conditions, making it attractive for industrial use. The 30 nm thickness significantly reduced the amount of the graphene materials, thus the costs, making it accessible for real life applications.”
BREAKING NEWS
Materials with adaptable properties, like those of wood and bone, can provide safer structures, save money and resources, and reduce their environmental impact, claim the team at Johns Hopkins University. “Imagine a bone implant or a bridge that can self-reinforce where a high force is applied without inspection and maintenance. It will allow safer implants and bridges with minimal complication, cost and downtime,” said Sung Hoon Kang, an assistant professor in the Department of Mechanical Engineering, Hopkins Extreme Materials Institute, and Institute for NanoBioTechnology at Johns Hopkins University. Bones use cell signals to control the addition or removal of minerals taken from blood around them. Inspired by these natural materials, Kang and colleagues sought to create a materials system that could add minerals in response to applied stress. The team started with materials that convert mechanical forces into electrical charges as scaffolds that can create charges proportional to external force placed on it. The team’s hope was that these charges could serve as signals for the materials to start mineralisation from mineral ions in the environment. Kang and colleagues immersed polymer films of these materials in a simulated body fluid mimicking ionic concentrations of human blood plasma. After the materials incubated in the simulated body fluid, minerals started forming on the surfaces. The team found they could control the types of minerals formed by controlling the fluid’s ion composition. Kang hopes that these materials can someday be used as scaffolds to accelerate treatment of bone-related disease or fracture, smart resins for dental treatments or other similar applications.
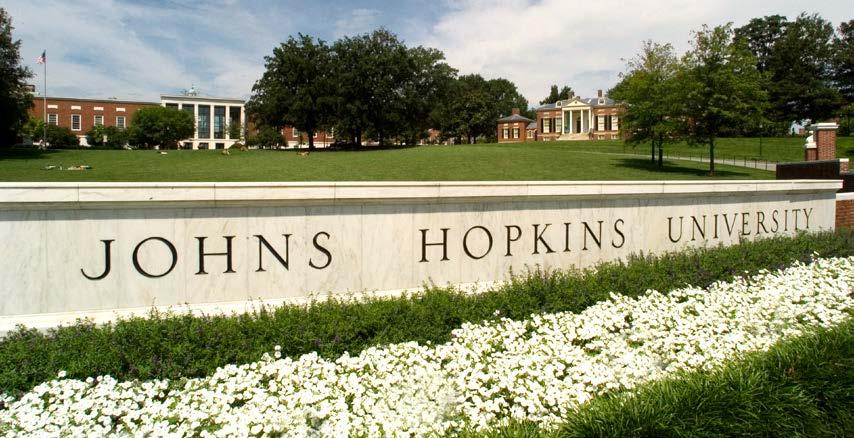
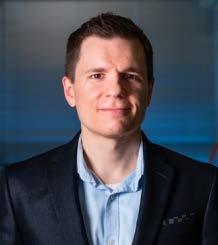
Professor Rob MacFarlane studies materials science and engineering at MIT. Image credit: Lillie Paquette, MIT School of Engineering.
3Q: Robert Macfarlane on Cleaning Your Smartphone Screen
To help curb the spread of the COVID-19 virus, public health experts have emphasised the need for cleaning and disinfecting frequently touched surfaces. But what about our smartphones and other personal electronic devices that we touch throughout the day? Many smartphone manufacturers advise against using cleaning products or disinfectants to clean touchscreens. Robert Macfarlane, the Paul M. Cook Assistant Professor in Materials Science and Engineering at MIT, is developing new techniques to manufacture materials via self-assembly that could eventually be used to create protective coatings, adhesives, sensors, and other types of devices. Macfarlane explained that placing an ‘oleophobic’ coating on a surface — a material that does not have a strong affinity for binding to oily substances — minimises the amount of oils that are left behind. Oleophobic coatings are commonly made of fluorinated polymers or fluorocarbons, which are classes of molecules that do not really have strong binding affinity for either water-soluble or oil-soluble materials. While using rubbing alcohol or an alcohol-based wipe is unlikely to remove this coating entirely, prolonged exposure to alcohols in high concentration could potentially disrupt this coating by either removing small patches of the fluorocarbons or altering the coating’s distribution so that it is not entirely uniform across a surface. “The oleophobic coatings that we already use are not entirely impossible to clean, and they probably already prevent microbial adhesion at least to a limited degree. However, they don’t completely eliminate the possibility of contamination, which is something we are now thinking about a lot more given the current pandemic,” Macfarlane explained.
Team of Canadian and Italian Researchers Breaking New Ground in Materials Science
A study by a team of researchers from Canada and Italy recently published in Nature Materials could usher in a revolutionary development in materials science, leading to big changes in the way companies create modern electronics. The goal was to develop two-dimensional materials, which are a single atomic layer thick, with added functionality to extend the revolutionary developments in materials science that started with the discovery of graphene in 2004. These results will foster more studies on a wide range of two-dimensional conjugated polymers with different lattice symmetries, thereby gaining further insights into the structure versus properties of these systems. The Italian and Canadian team demonstrated the synthesis of large-scale two-dimensional conjugated polymers, also thoroughly characterising their electronic properties. They achieved success by combining the complementary expertise of organic chemists and surface scientists.
BREAKING NEWS
A global research team, headed by Monash University, is pioneering a breakthrough recyclable material that could revolutionise the construction industry, assist disaster-affected areas, and become used in space exploration. Researchers are leading the development of archimats – an emerging area of ‘architectured’ materials that have an organised intertwined or interlocked inner architecture. As a result, archimats have an extra degree of freedom expanding the design space that conventional composite materials, such as concrete, cannot possess. Archimats can be engineered to have superior strength, enhanced ductility, a high tolerance to damage, good thermal insulation and sound absorption. They can also better absorb energy, as well as provide improved compliance and flexibility. One way to achieve this superior property profile, especially of metallic materials, is through severe plastic deformation (SPD) – a special metalworking technique that results in an ultrafine grain size or nanocrystalline structure. The structural patterns caused by SPD processing can improve the mechanical characteristics and physical properties of materials. With the potential to revolutionise the construction, materials and production industries, archimats can be used: • In the construction industry to reduce the use of concrete and cut carbon dioxide emissions associated with its production; • To build or rebuild in arid or disaster-affected zones. This includes rapidly deployable and removable structures in danger areas, such as a town or city impacted by fire, for first responders and displaced citizens; • In extra-terrestrial and space construction. The European
Space Agency is already considering this type of architectured material for the construction of a lunar base; and • In smart toys and games, such as 2D and 3D puzzles.
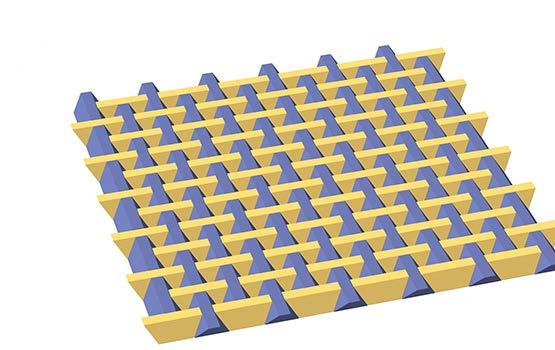
Mortarless construction: rapidly deployable pavements composed of interlocked tetrahedronshaped blocks made from different materials. Image courtesy of Monash University.
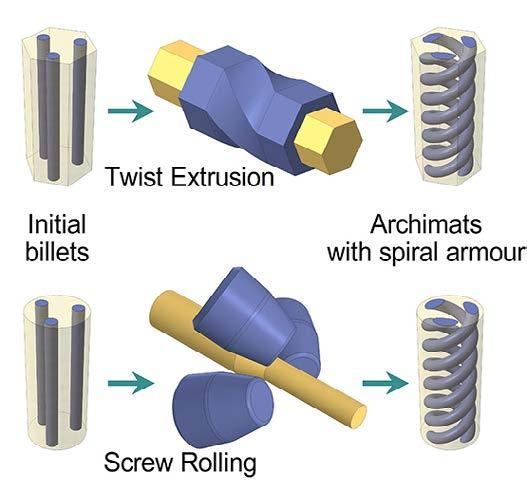
Employing the SPD processes of twist extrusion and screw rolling to produce archimats with spiral armour. Image courtesy of Monash University.
Interfaces the Key in Atomically-Thin, ‘High Temperature’ Superconductors
An international FLEET collaboration publishing a review of atomically-thin ‘high temperature’ superconductors finds that each has a common driving mechanism: interfaces. The team, including researchers from the University of Wollongong, Monash University and Tsinghua University (Beijing), found that interfaces between materials were the key to superconductivity in all systems examined. The enhancement of superconductivity at interfaces (interface superconductivity enhancement effect) in atomically-thin superconductors is a unique tool for discovering new hightemperature superconductors, and could be used to finally unlock the elusive mechanism behind high-temperature superconductivity. Systems studied include: • Elemental metals grown on semiconductors • Single-layer iron-based superconductors • Atomically-thin cuprate (copper based) superconductors The review investigated the role of molecular-beam epitaxy (MBE), scanning tunnelling spectroscopy (STM/STS), scanning transmission electron microscopy (STEM), physical properties measurement system (PPMS), in fabricating and identifying atomically-thin superconductors.
Breakthrough in Energy Storage Set to Drive a Cleaner Future
Deakin’s Institute for Frontier Materials researchers, in collaboration with researchers from the University of Western Australia and Monash University, have discovered that super concentrated ionic liquids are key to achieving better batteries for future use. The new work by ARC Centre of Excellence for Electromaterials Science (ACES) PhD student, Dmitrii Rakov and co-led by Professor Maria Forsyth and Dr Fangfang Chen presents an important breakthrough in the field, paving the way for the next generation of high energy density batteries for use in households and industries worldwide. Professor Forsyth said this breakthrough is a key to achieving a clean energy future. “This finding is very significant because it explains for the first time why an ideal combination of sodium metal anode and safe, high performing ionic liquid electrolytes can lead to much better batteries all round.” “Using molecular simulations coupled with experimental design, this is the first time we’ve been able to explain this optimised behaviour. Society is craving increased battery life, energy density and safety for state of the art Lithium ion batteries that can be used in energy storage for applications such as powering transportation and clean energy for homes,” Professor Forsyth said. Current worldwide progress has been constrained by the type of electrolytes commonly used to make batteries. While their work has demonstrated the mechanism for sodium metal batteries, the team said the findings are applicable to other advanced batteries including lithium and zinc metal batteries.