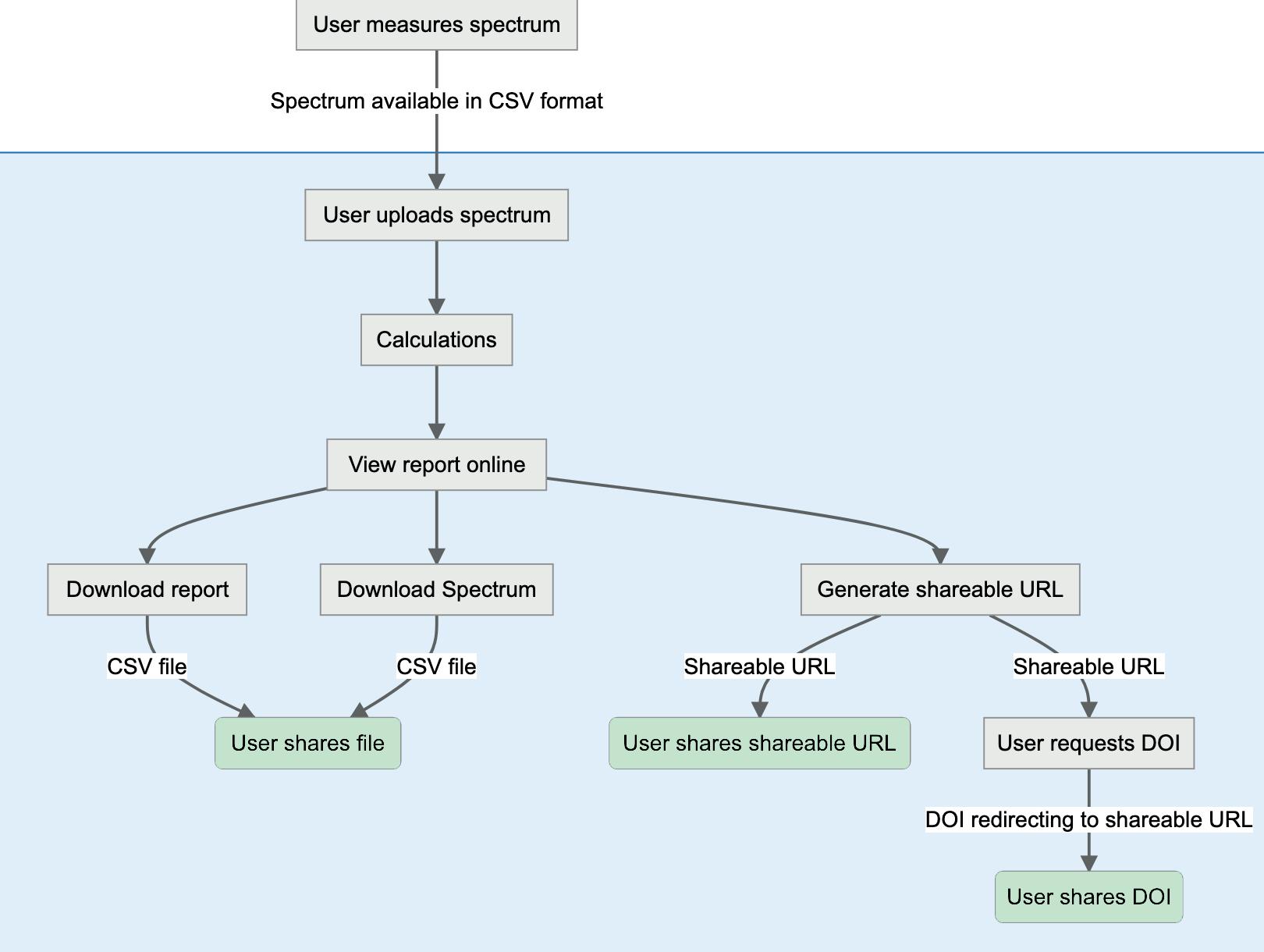
5 minute read
A MEASURE OF THE UNSEEN
Biomedical research on these non-visual effects of light is very much ongoing. We A MEASURE OF THE UNSEEN now hold several key pieces of the puzzle, including the primary role of melanopsin in driving these effects, and a developing understanding of how much light is necessary to support optimal health and performance.1 Over the past decade or so, The 2020 Jean Heap Research Bursary recipient, there has also been considerable interest in optimising lighting solutions for the built Manuel Spitschan, outlines his research subject, environment. 'Human-centric lighting' is luox, a novel open-access platform for quantifying one of the terms that is often used, and the HCL market is large and growing. the non-visual effects of light UNDERSTANDING THE NONVISUAL EFFECTS OF LIGHT
The quality and quantity of light in our environment profoundly affect how we feel, how and when we sleep, and how we appreciate a given space. Over the past few years biomedical research has found that different pathways connect the eye to the brain and influence different functions.
Advertisement
We have, of course, known about cones and rods for a long time. While the cones allow us to see colour, motion and spatial detail under daytime lighting conditions (photopic vision), the rods give us rudimentary vision under lighting typically dimmer than natural twilight (scotopic vision).
As has now become more widely known, around 20 years ago a new type of neuron was identified: the intrinsically photosensitive retinal ganglion cells, or ipRGCs, which are sensitive to light independent of the cones and rods. They respond to light due to the photopigment melanopsin. Melanopsin is most sensitive to short-wavelength, or blue light, with a peak sensitivity near 480nm.
The ipRGCs are involved in a range of effects important for our health and wellbeing. Most prominently, they signal the intensity of environmental illumination and thereby track the light-dark signal. Signals from the ipRGCs are sent to the suprachiasmatic nucleus (SCN), an area buried deep in the brain about the size of a grain of rice. The SCN is our circadian pacemaker, telling our brain and body whether it is day or night – biologically speaking. Through this brain pathway, rhythms in our physiology and behaviour become synchronised to the light-dark cycle in our environment.
For example, the body starts producing the 'sleep hormone' melatonin a few hours before our usual bedtime. Exposure to light in the evening and at night can disturb melatonin production and shift our circadian rhythms. Exposing yourself to light at the wrong time can lead to circadian disruption: the misalignment of our biological clock with solar time, which has negative consequences for mental and physical health in the long run. Recent meta-analyses have shown that melanopsin best explains our current evidence base for the non-visual effects of light,2 simplifying the daunting and challenging task of quantifying the impact a given spectrum of light has. However, the spectral sensitivity of melanopsin and the photopic luminosity curve (Vλ), which is used to calculate (il)luminance, are different. Vλ reflects a weighted combination of the L and M cones in the retina. Consequently, the non-visual effects of light cannot generally be predicted by (il)luminance, requiring metrics reflecting the melanopsin-weighted (or melanopic) signal.
p Fig 1: Workflow for using luox platform
In 2018, the International Commission on Illumination (CIE) published the CIE S 026/E:2018 standard.3 This includes a set of spectral sensitivity curves for the cones, rods and melanopsin. These curves are used to convert a spectrum (giving radiance or irradiance for each wavelength) into a physiologically weighted, alpha-opic quantity for each photoreceptor class.
For melanopsin, the melanopic irradiance, expressed in mW/sqm, is the spectral irradiance weighted by the melanopsin spectral sensitivity. In addition to these fundamental quantities, the standard also formalises the melanopic equivalent daylight illuminance (melanopic EDI), which quantifies the illuminance a standard D65 daylight spectrum would have with the melanopic irradiance. In other words: how bright (measured in lux) would daylight have to be to have a matched melanopic irradiance?
HOW LUOX WORKS
Lighting calculations are often not simple or can require specialised tools. Which is where luox, a novel web platform for facilitating these calculations, comes in.
The user will upload a spectral power distribution (SPD) – radiance or irradiance – and the platform calculates, using browserside computations, a range of key quantities, including (il)luminance, chromaticity and alpha-opic quantities. The spectrum is visualised and can be compared to a range of CIE reference spectra, including CIE Standard Illuminants A and D65, Illuminants C, E, D50, D55 and D75, the F series, and others.
The user can then download these quantities as a comma-separated (CSV) file. Additionally, the spectrum can be shared using a new spectral compression library called spdurl, developed by Michael Herf, which directly encodes the spectrum in a URL. The uploaded spectrum can then be recalled at a later point, simply by pointing to the same URL. Using this scheme, no data are stored on a server. Users are also able to request a digital object identifier (DOI) which creates a permanent meta-data record and URL for a stored record. The workflow is shown in Figure 1.
The luox platform is free – both in the open-source sense and delivered at no cost. A CIE task group is in the process of validating calculated quantities.
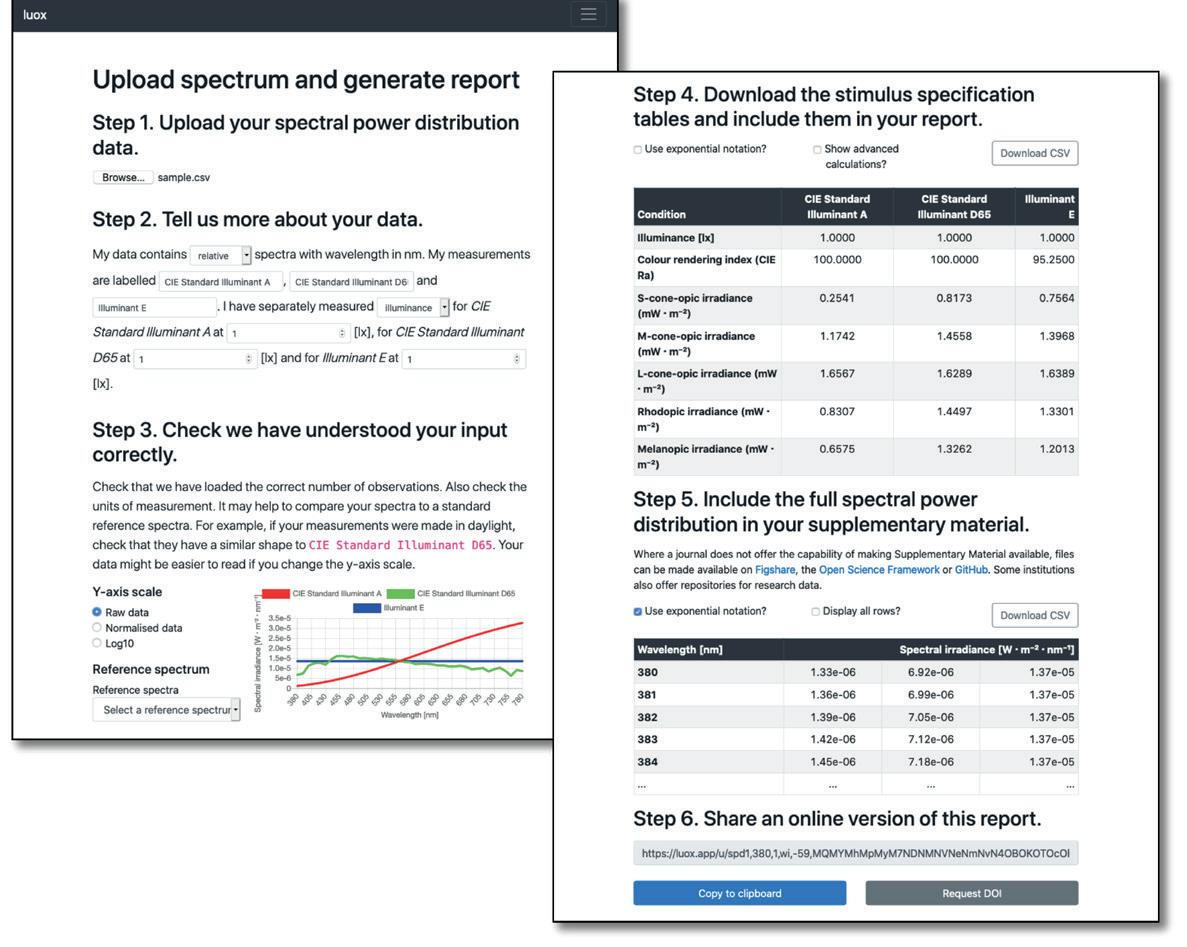
p Fig 2: The process step by step The luox platform is at https://luox.app/ and the source code is available at https:// github.com/luox-app/luox under the GPL-3.0 License, making all calculations transparent and allowing contributions from interested users. Manuel Spitschan is currently a research fellow at the University of Oxford and a visiting fellow at the Centre for Chronobiology, Basel. For any questions, comments or suggestions, the author can be contacted at manuel.spitschan@psy.ox.ac.uk
The 2020 Jean Heap Research Bursary was awarded to Manuel Spitschan, PhD, for his project, luox.app: Development, validation and refinement of a free, open-access tool for calculations related to light and lighting. In addition to the SLL bursary, development of luox was funded by the Wellcome Trust (Research Enrichment – Open Research, 204686/Z/16/C) and the van Houten Fund of the University of Oxford (VH-148).
References
1 https://www.preprints.org/ manuscript/202012.0037/v1 2 https://onlinelibrary.wiley. com/doi/full/10.1111/jpi.12655 3 https://cie.co.at/publications/ cie-system-metrology-opticalradiation-iprgc-influencedresponses-light-0