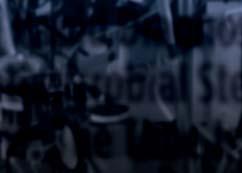
34 minute read
Implementing Rapid Diagnostic Tests to Augment Antimicrobial Stewardship Programs The Time Is Now
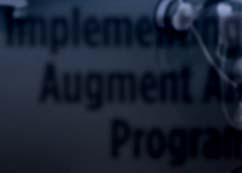
BY SARAH M. WIECZORKIEWICZ, PHARMD, FIDSA, BCPS, BCIDP
For hospitalized, critically ill patients with sepsis, accurate and timely administration of antimicrobial agents is essential to prevent mortality.1,2 Rapid diagnostic tests (RDTs) have revolutionized the treatment of these patients and become one of the most powerful tools for antimicrobial stewardship programs (ASPs). Although routinely used laboratory methods, such as Gram stains, can provide helpful guidance in some clinical circumstances—such as stopping gram-positive coverage when a gram-negative organism is identified for a generally monomicrobial infection, de-escalating antipseudomonal coverage if ruled out, and so forth—they do not predict antimicrobial susceptibility patterns. In addition, routine laboratory methods often result in prolonged use of unnecessarily broad-spectrum antimicrobials or, even more concerning, ineffective therapy.
RDTs provide actionable details, even those without rapid susceptibility results, such as the ability to predict resistance patterns. When combined with the use of local antibiograms, knowledge of intrinsic resistance, and genotypic resistance marker detection, RDTs allow a more rapid, targeted approach to antimicrobial therapy.
The impact of RDTs can be largely attributed to reduced duration of empiric antimicrobial therapy. Prolonged broad-spectrum empiric therapy can lead to an increased burden of Clostridioides difficile infection, antimicrobial resistance, and adverse events, such as acute kidney injury. On the contrary, erroneous empiric antimicrobial coverage can delay effective therapy to detrimental effects and lead to dramatic increases in mortality among critically ill patients.1-8 A recent study found delays in antimicrobial therapy in patients with carbapenem-resistant and -susceptible Enterobacteriaceae infections are more important for mortality than multidrug resistance (MDR).7
However, MDR had been identified as an independent predictor of delays in time to effective therapy (TTET), likely as a result of providing inadequate empiric coverage,9 highlighting the need for RDTs with either genotypic resistance marker detection or rapid antimicrobial susceptibility testing (AST) for geographic areas with problematic multidrug-resistant organisms (MDROs). When combined with ASPs, RDTs demonstrate substantial benefit on patient outcomes, including mortality10 and attributable cost.11
With the availability of many RDT options, diagnostic stewardship is an essential component of ASPs enabling the teams to select the right test, for the right patient, at the right time.12 Furthermore, practical concerns for specific sites should be considered by the ASP, ideally comprising a multidisciplinary group that includes infectious diseases physicians and pharmacists, microbiologists, nurses, infection preventionists, information technology specialists, hospital epidemiologists, and other pertinent invested frontline providers.13 Global considerations to assess when choosing RDT platforms include, but are not limited to, institutional or regional problematic pathogens, the patient population served (ie, pediatric, adult, and/ or geriatric; immunocompromised vs general community), laboratory hours, location (ie, centralized or decentralized), operations and workflow, and leadership support. Specific practical considerations for FDA-cleared RDTs are outlined in the Table.
Barriers to RDT implementation and solutions that are justified by the CDC Core Elements of leadership commitment, accountability, pharmacy expertise (previously drug expertise), action, tracking, reporting, and education have been described.14 This article provides a clinically practical overview on the selection of RDTs for infectious syndromes, including bloodstream infections (BSIs), respiratory tract infections (RTIs), central nervous system (CNS) infections, and gastrointestinal (GI) infections.
Bloodstream Infections
Rapid blood culture identification technologies are among the most commonly used RDTs, and are endorsed by the Infectious Diseases Society of America (IDSA) guidelines for implementing ASPs to optimize antimicrobial use.13 This endorsement is supported by a substantial number of studies that demonstrate ASPs combined with molecular RDTs have favorable clinical outcomes, including a significant reduction in mortality in a meta-analysis of 31 mostly quasi-experimental studies.10,15-23 Available technologies identify organisms through genotypic (eg, nucleic acid amplification testing [NAAT]) and/or phenotypic (eg, biochemical assays, mass spectrometry, nuclear magnetic resonance [NMR] spectrometry) methods. FDA-cleared rapid assays from various manufacturers include peptic nucleic acid fluorescent in situ hybridization, matrix-assisted laser desorption/ionization time-offlight mass spectrometry (MALDI-TOF MS), polymerase chain reaction (PCR), multiplex PCR panels, nanoparticle probe technology, and NMR. Although most RDTs require organism isolation before identification, and previously included none or minimal genotypic resistance testing, this strategy is rapidly evolving with the need to further close the gap between broadspectrum empiric and targeted therapy.
This evolution is exemplified by the T2 Magnetic Resonance (T2MR) technology by T2 Biosystems that can detect the presence of organisms directly from a whole blood specimen without prior isolation. T2Candida, T2Bacteria, T2Resistance, and T2SARSCoV-2 (T2 Biosystems) panels are rapid detection panels that use this strategy. T2Resistance is not available for clinical use in the United States but has been granted a “breakthrough device” designation by the FDA in response to combating resistant infections, and will be useful in immunocompromised patients, as well as patients or regions with a high burden of MDROs. T2Resistance can identify gram-negative markers including KPC,OXA-48, NDM/VIM/IMP, CTX-M, and 14/15AmpC (CMY/DHA), as well as gram-positive markers including vanA/B and mecA/C. The T2SARSCoV-2 test was granted emergency use authorization from the FDA in 2020 to assess patients with signs and symptoms of COVID-19 by detecting nucleic acid from SARS-CoV-2 in upper respiratory secretions and may, in theory, allow earlier discontinuation of unnecessary empiric antimicrobial use in this patient population. T2Bacteria and T2Candida are FDA-cleared, commercially available assays that use a miniaturized MR diagnostic technique that assesses the reaction of water molecules in the presence of magnetic fields, and can detect various targets in 3 to 5 hours.24 Candidemia mortality rates are as high as 30% to 40%, and increase by approximately 50% each day therapy is delayed.24 This is concerning, as prompt antifungal initiation can be inadvertently missed, given the overall sensitivity of blood cultures in diagnosing invasive candidiasis is around 50%.25 The T2Candida assay can be incredibly useful in facilities with high rates of fungal infections (eg, immunocompromised patient population), as it not only detects organisms at densities of 1 colony-forming unit (CFU)/mL compared with 100 to 1,000 CFU/mL required for PCR-based detection,24 but also has the ability to substantially reduce TTET once detected.26 Patients with positive blood cultures identified by T2Candida noted a 27-hour reduction in time to appropriate antifungal therapy (P=0.01).27 Conversely, ASPs can use negative tests to shorten antifungal therapy. Previous data demonstrate this can be associated with a 4.3-day reduction in micafungin use, resulting in a cost savings of $48,440 on antifungals.27
The T2Bacteria assay uses multiplex detection to
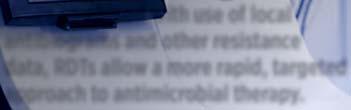
When combined with use of local antibiograms and other resistance data, RDTs allow a more rapid, targeted approach to antimicrobial therapy.
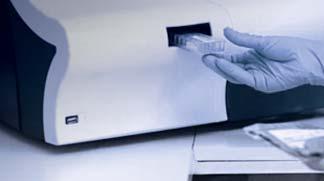
identify common ESKAPE organisms (ie, Enterococcus faecium, Staphylococcus aureus, Klebsiella pneumoniae, Pseudomonas aeruginosa, and Escherichia coli) that pose clinical challenges due to their propensity for MDR.28,29 In a performance assessment study, the T2Bacteria assay identified organism species in 3.61±0.2 to 7.7±1.38 hours, with a per-patient sensitivity of 90% (95% CI, 76%-96%), specificity of 90% (95% CI, 88%-91%), and negative predictive value of 99.7% for proven BSIs.29 Another study duplicated the negative predictive value of 99.8% and further demonstrated the mean time to detection and species identification was 5.5±1.4 hours.28 The T2Bacteria panel has demonstrated the ability to detect pathogens approximately 55 hours faster than standard methods (eg, blood culture) in patients with sepsis.30 Given the previous benefit of RDTs including reductions in TTET, time to optimal therapy, mortality, and the observation of ASP intervention being an independent predictor of survival in MDR infections,31 the T2Bacteria assay is expected to demonstrate favorable clinical outcomes. While T2MR technology reduces time to organism identification by eliminating the need for prior organism isolation, other technologies target rapid phenotypic AST to prevent delays in therapy and avoid unnecessarily prolonged broad-spectrum therapy. One such technology is the Accelerate Pheno system (Accelerate Diagnostics), which uses rapid phenotypic methods to provide AST within 7 hours. Conventional identification with AST can take as long as 90 hours.32 This is particularly important when clinicians are concerned about prolonged exposure of insufficient antimicrobial concentrations (eg, patients with unpredictable pharmacokinetics/pharmacodynamics, augmented renal clearance, or pathogens with a high minimum inhibitory concentration), as inappropriate exposure can result in poor outcomes.32,33 A recent multicenter, randomized controlled trial comparing rapid identification plus phenotypic AST (RAPID; Accelerate Pheno) with standard of care (SOC; MALDI-TOF MS) plus AST using broth microdilution or agar dilution was conducted in patients with gram-negative BSIs.34 The primary outcome measure was time to first antimicrobial modification within 72 hours of randomization. First antimicrobial modification was 6.3 hours faster in the RAPID group compared with the SOC group for overall antimicrobials (median [interquartile range], 8.6 [2.6-27.6] vs 14.9 [3.3-41.1] hours; P=0.02), and 24.8 hours faster for antimicrobials targeted against gramnegative organisms (median [interquartile range], 17.3 [4.9-72] vs 42.1 [10.1-72] hours; P<0.001). Among a subset of 40 patients in the RAPID group and 44 in the SOC group with resistant organisms, time to antimicrobial escalation was 43.3 hours faster in the RAPID group than the SOC group (P=0.01), while no difference was observed in de-escalations. Although the study authors report no significant differences in clinical outcomes, such as length of stay (LOS) and mortality, the ability to escalate therapy more rapidly and reduce time to effective therapy is clinically meaningful nonetheless.34 One recent quasi-experimental study of 830 bacteremic cases, however, has demonstrated a shorter median LOS (6.3-6.7 vs 8.1 days) when the Accelerate Pheno is used, regardless of whether it is paired with or without real-time notification when compared with historical controls lacking rapid diagnostics or antimicrobial stewardship intervention.36 Overall, rapid blood culture identification systems demonstrate a substantial benefit when paired with ASPs for both clinical and economic outcomes.10,11 Probabilistic analyses of RDTs combined with ASPs show an 80% chance of being cost-effective versus a 41% chance without ASPs.11 These data suggest RDTs should be the SOC across all hospitals and continue to evolve with public health needs to slow the progression of resistance and ensure antimicrobial therapy is optimized.
Karius testing is a blood test based on next-generation sequencing of microbial cell-free DNA, allowing it to quantify more than 1,000 clinically relevant pathogens that include viruses, bacteria, fungi, and parasites. It may be useful for patients with severe illness, those who are immunocompromised, and/or those with a lack of pathogen identification after standard attempts via other methodologies.37 A prospective pilot study explored the utility of Karius testing as a BSI prediction tool among 47 pediatric patients with relapsed or refractory cancers. Sixteen BSI episodes (15 bacterial) were available for predictive sampling, and a predictive sensitivity of 80% (n=12/15; 95% CI, 55%-93%) was identified for bacterial BSIs.37 Hogan et al performed a retrospective cohort study to determine the real-world impact of the Karius test ordered for all suspected infectious diseases indications.38 Approximately 65% of patients were immunocompromised and 52.4% were children. A total of 82 Karius tests were evaluated with a positivity rate of 61%. The Karius test results led
Table. Selected FDA-Approved Rapid Diagnostic Tests and Clinical Practice Considerations a
Resistance Detection Practical Considerations for Clinical Practice
Detection Time
(Pre-blood culture)
Example Systems (Manufacturer) Organisms on Panel
Technology Type Blood Cultures
• Potential benefit for patients requiring rapid PK/PD dose adjustments (eg, augmented renal clearance) • Ability to rapidly detect resistance patterns • Unreliable MIC for bacteria with multiple morphologies or polymicrobial infections
MRSA and macrolide- lincosamide- streptogramin B phenotypically reported
~1.5 h to identify, and 7 h to antimicrobial susceptibility
Staphylococcus Staphylococcus aureus , lugdunensis ,CoNS, Enterococcus faecalis , Enterococcus faecium , Streptococcus species, Escherichia coli , Klebsiella species, Enterobacter species, Proteus species, Citrobacter species , Pseudomonas aeruginosa , Serratia marcescens , Acinetobacter baumannii, Candida albicans, Candida glabrata CoNS 20 min None • Previously associated with reduction in LOS in CoNS-positive cultures, and reduced TTET in enterococcal bacteremia • Limited number of targets per panel
E. faecalis, E. faecium, Enterococcus species 20 min None 20 min None • Limited number of targets per panel • Useful among community hospitals with low resistance rate
E. coli, Klebsiella pneumoniae, P. aeruginosa
PNA-FISH Accelerate Pheno (Accelerate Diagnostics) Staphylococcus QuickFISH (AdvanDx) Enterococcus QuickFISH (AdvanDx) Gram-negative QuickFISH (AdvanDx) 90 min None • Useful for hospitals with high fungal infections (eg, immunocompromised)
C. albicans, C. glabrata, Candida krusei, Candida parapsilosis, Candida tropicalis
Yeast Traffic Light (AdvanDx) • Prompt identification of MRSA • May miss MRSA with non-mecA resistance
mecA • Prompt differentiation between MRSA and MSSA can facilitate faster TTET
SCCmec, mecA • Limited number of targets
mecA
None 20 min
XpressFISH mecA (AdvanDx) 1 h 2 h
S. aureus
PCR Xpert MRSA/SA BC (Cepheid) S. aureus
StaphSR (BD GeneOhm) • Comprehensive number of targets • Multiple primers allow for identification of multiple organisms • Identifies polymicrobial infections that may be missed by other technologies
mecA, vanA/B, KPC
1 h
A. baumannii, C. albicans, C. glabrata, C. krusei, C. parapsilosis, C. tropicalis, E. coli, Enterobacter , Enterobacteriaceae , cloacae complex Enterococcus species, Haemophilus influenzae, Klebsiella oxytoca, K. pneumoniae , Listeria monocytogenes, Proteus species , P. aeruginosa, Neisseria meningitidis, S. marcescens, Staphylococcus species, S. aureus, Streptococcus species, Streptococcus agalactiae, Streptococcus pneumoniae, Streptococcus
mPCR FilmArray BCID (BioFire Diagnostics) pyogenes
• Most comprehensive organism and resistance detection panels currently available on the market • Gram-positive and gram-negative panels contain pan targets to ensure organism inclusivity • Identifies polymicrobial infections that may be missed by other technologies
mecA, mecC, vanA/B
1.5 h CTX-M, IMP, KPC, NDM, OXA (OXA 23 and -48), OXA VIM
1.5 h 1.5 h None
Bacillus cereus group , Bacillus subtilis group, Corynebacterium, Cutibacterium acnes, Enterococcus, E. faecalis, E. faecium, Lactobacillus, Listeria, L. monocytogenes, Micrococcus, Staphylococcus, S. aureus, Staphylococcus epidermidis, S. lugdunensis, Streptococcus, S. agalactiae
(Group B Streptococcus anginosus Streptococcus ), , S. pneumoniae, S. pyogenes (Group A group Streptococcus ), Pan targets: pan–gram-negative and panCandida A. baumannii, Bacteroides fragilis, Citrobacter, Cronobacter sakazakii, Enterobacter (non- complex, E. coli, cloacae complex), E. cloacae Fusobacterium nucleatum, Fusobacterium necrophorum, H. influenzae, K. oxytoca, K. pneumoniae group , Morganella morganii, N. meningitidis, Proteus, Proteus mirabilis, P. aeruginosa, Salmonella, Serratia, S. marcescens, Stenotrophomonas maltophilia Pan targets: pan–gram-positive and panCandida C. albicans, Candida auris, Candida dubliniensis, Candida famata, C. glabrata, Candida guilliermondii, Candida kefyr, C. krusei, Candida lusitaniae, C. parapsilosis, C. tropicalis, Cryptococcus gattii, Cryptococcus neoformans, Fusarium, Rhodotorula
ePlex BCID-GP panel (GenMark Diagnostics) ePlex BCID-GN panel (GenMark Diagnostics) ePlex BCID-FP panel (GenMark Diagnostics) All organisms 30 min None • Ability to detect a vast array of bacterial and fungal microbes • Useful for facilities with patients at risk for uncommon infections (eg, immunocompromised population, transplant centers) • Lacks ability to detect resistance markers or provide susceptibility reports
MALDI-TOF MALDI-TOF (bioMérieux and Brucker) • Can reduce TTET and unnecessary antimicrobial utilization • Unreliable for detection in polymicrobial bacteremia
CTX-M, IMP, KPC, NDM, OXA, VIM mecA, vanA/B
2.5 h 2.5 h
Acinetobacter species , Citrobacter species , E. coli, Enterobacter species, K. oxytoca, K. pneumoniae, species P. aeruginosa, Proteus
VERIGENE BC-GN (Luminex)
Nanoparticle probe technology S. aureus, S. epidermidis, S. lugdunensis, S. anginosus, S. agalactiae, S. pneumoniae, S. pyogenes, E. faecalis, E. faecium
VERIGENE BC-GP (Luminex)
a Not an all-inclusive list. Table adapted and refined from reference 68. gastrointestinal; LOS, length of stay; MALDI-TOF, matrix-assisted laser ASP, antimicrobial stewardship program; CNS, central nervous system; CoNS, coagulase-negative Staphylococcus ; GI, multiplex polymerase chain reaction; MRSA, methicillin-resistant Staphylococcus aureus ; minimum inhibitory concentration; mPCR, desorption/ionization time of flight; MIC,
(Pre-blood culture) 3-5 h None • Detects presence of organisms directly from blood specimen (ie, prior isolation not required) substantially expediting time to identification • Low limit of detection can detect
mecA/C, vanA/B
3-5 h
pathogens missed by standard testing that is reliant on blood cultures.
Example Systems (Manufacturer) Organisms on Panel
Technology Type
E. faecium, S. aureus, K. pneumoniae, P. aeruginosa, E. coli
NMR T2Bacteria C. albicans, C. tropicalis, C. krusei, C. glabrata, C. parapsilosis
T2Candida • T2Bacteria Panel detects 90% of all ESKAPE pathogens and T2Candida detects the 5 most common sepsis-causing fungal pathogens 1 h None • Implementation may be most useful for hospitals with pediatric and neonatal patients • Associated with a rapid time to diagnosis of viral infections • Not intended to replace traditional diagnostic testing for CNS infections
Central Nervous System
E. coli (K1 serotype only), H. influenzae, L. monocytogenes, N. meningitidis (encapsulated strains only) , S. agalactiae, S. pneumoniae, C. neoformans/gattii , cytomegalovirus, enterovirus, herpes simplex viruses 1 and 2, human herpesvirus 6, human parechovirus, and varicella-zoster virus
mPCR FilmArray ME (BioFire Diagnostics)
Gastrointestinal
1 h None • Cannot differentiate between live and dead organisms • Infants and young children <3 y likely test positive for C. difficile due to high rates of colonization; pediatric centers may blind C. difficile results or include clinical decision support to interpret positives in this population with caution • Many GI pathogens may be shed asymptomatically for prolonged periods complicating positive results • Should not be used as a test of cure • Can detect multiple pathogens; h owever, the clinical significance to polymicrobial GI infections is unknown
5 h None • Consider implementing in high-prevalence units (eg, pediatrics)
<2 h None
Campylobacter species ( jejuni, coli, upsaliensis ), Clostridioides difficile (toxin A/B), Plesiomonas shigelloides,Salmonella species, Yersinia enterocolitica , Vibrio species ( parahaemolyticus, vulnificus ), Vibrio cholerae , enteroaggregative E. coli , enteropathogenic E. coli , enterotoxigenic stx1/ , Shiga-like toxin–producing E. coli E. coli E. coli 0157, Shigella /enteroinvasive E. stx2, coli , adenovirus F 40/41, astrovirus, norovirus GI/GII, rotavirus A, Sapovirus (I, II, IV, V), Cryptosporidium , Cyclospora cayetanensis , Giardia lamblia Entamoebahistolytica ,
mPCR FilmArray GP (BioFire Diagnostics) Campylobacter species ( jejuni, coli, lari ) , C. difficile toxin A/B,enterohemorrhagic E. coli , enterotoxigenic E. coli , E. coli O157, Salmonella species, Shigella species (boydii, sonnei, flexneri, dysenteriae ), Vibrio species( cholerae ), Y. enterocolitica (not in US), adenovirus F40/41, Norovirus GI/GII, rotavirus A, Cryptosporidium species ( parvum, hominis), E. histolytica, G. lamblia
xTAG GPP (Luminex Corp) Salmonella species, Campylobacter group, Shigella species, Vibrio group, Yersinia enterocolitica , Shiga toxin 1 (stx1), Shiga toxin 2 (stx2), norovirus, rotavirus
VERIGENE Enteric Pathogens Test in the Gastro (Luminex) Rotavirus, astrovirus, adenovirus 2.5 h None
InGenius Gastrointestinal Viral Elite Panel (ELITechGroup)
• Implementation encouraged in institutions with high rates of inappropriate prescribing for respiratory tract infections • Can rule viral etiologies in or out • In conjunction with procalcitonin, can assist ASP in reducing antimicrobial utilization
mecA/C and MREJ KPC, NDM, OXA- 48-like, VIM, IMP, CTX-M • First pneumonia panel with 15 bacterial isolated and resistance detection capabilities
1 h 1 h None 90 min None <2 h None
Adenovirus, coronavirus, human metapneumovirus, human rhinovirus/enterovirus, influenza A, influenza B, parainfluenza virus, Acinetobacter calcoaceticus-baumannii RSV, E. coli, H. influenzae, complex, E. cloacae complex, Klebsiella aerogenes, K. oxytoca, K. pneumoniae group, Moraxella catarrhalis, , P. aeruginosa, S. marcescens, species Proteus S. aureus, S. agalactiae, S. pneumoniae, S. pyogenes, Chlamydia pneumoniae, Legionella pneumophila, Mycoplasma pneumoniae Adenovirus, coronavirus HKU1, coronavirus NL63, coronavirus 229E, coronavirus OC43, human metapneumovirus, human rhinovirus/enterovirus, influenza A, influenza A/H1, influenza A/H3, influenza A/H1-2009, influenza B, parainfluenza virus 1, parainfluenza Virus 2, parainfluenza virus 3, parainfluenza virus 4, RSV, Bordetella pertussis, C. pneumoniae, M. pneumoniae; RP2 panel adds Bordetella parapertussis
Respiratory
mPCR The BioFire FilmArray Pneumonia (PN) panel FilmArray RP and RP2 (BioFire Diagnostics) Adenovirus, coronavirus (229E, HKU1, NL63, OC43), human metapneumovirus, human rhinovirus/enterovirus, influenza A, influenza A H1, influenza A H1-2009, influenza A H3, influenza B, parainfluenza 1, parainfluenza 2, parainfluenza 3, parainfluenza 4, RSV A, RSV B, C. pneumoniae, M. pneumoniae
ePlex Respiratory Pathogen Panel (GenMark Diagnostics) Adenovirus, human metapneumovirus, influenza A, influenza A (subtype H1), influenza A (subtype H3), influenza B, parainfluenza 1, parainfluenza 2, parainfluenza 3, parainfluenza 4, rhinovirus, RSV A, RSV B, Bordetella pertussis, Bordetella parapertussis/B. bronchiseptica, Bordetella holmesii
VERIGENE Respiratory Pathogens Flex Test (Luminex) <3 h None
Influenza A, influenza A H1, influenza A H3, influenza B, RSV A, RSV B, rhinovirus/ enterovirus, parainfluenza virus 1, parainfluenza virus 2, parainfluenza virus 3, parainfluenza virus 4, human metapneumovirus, adenovirus, coronavirus HKU1, coronavirus NL63, coronavirus 229E, coronavirus OC43, human bocavirus, Chlamydophila pneumoniae, M. pneumoniae
NxTAG Respiratory Pathogens Panel (Luminex)
a Not an all-inclusive list. Table adapted and refined from reference 68. gastrointestinal; LOS, length of stay; MALDI-TOF, matrix-assisted laser ASP, antimicrobial stewardship program; CNS, central nervous system; CoNS, coagulase-negative Staphylococcus ; GI, methicillin-resistant Staphylococcus aureus ; multiplex polymerase chain reaction; MRSA, minimum inhibitory concentration; mPCR, desorption/ionization time of flight; MIC, peptic nucleic acid fluorescent in situ hybridization; pharmacokinetic/pharmacodynamic; PNA-FISH, nuclear magnetic resonance; PK/PD, ; NMR, methicillin-sensitive S. aureus
MSSA,
to a positive clinical impact in 7.3%, negative clinical impact in 3.7%, no clinical impact 86.6%, and indeterminant in 2.4%. Cases with a positive Karius result and clinical impact, bacteria, and/or fungi were involved compared with viruses or parasites. Karius testing was evaluated in 55 patients with febrile neutropenia.39 Sensitivity and specificity were 85% (41/48) and 100% (14/14), respectively. Time to diagnosis was shorter compared with traditional blood culture methodology (87%) and could have allowed early antimicrobial optimization in 47% of patients (ie, addition of antimicrobials [20%] mostly against anaerobes [12.7%], antivirals [14.5%], and/or antifungals [3.6%] and antimicrobial de-escalation [27.3%]). These data suggest that Karius testing may have a future role in enabling prompt initiation of antimicrobials specifically in immunocompromised hosts, although a clear role including optimal patient population has yet to be fully elucidated.
Respiratory
Acute RTIs are most commonly caused by viral organisms. However, differentiating between viral and bacterial pneumonia is a significant challenge, and often results in unnecessary antimicrobial use for viral infections,40 especially when bacterial coinfection cannot be ruled out. To date, no single test exists to make this differentiation, but several RDTs together can assist ASPs in the clinical decision-making process such as respiratory pathogen (viral/bacterial) panels, methicillin-resistant S. aureus (MRSA) nasal PCR, and biomarker expression (eg, procalcitonin). Procalcitonin is a pro-inflammatory biomarker previously associated with a reduction in antimicrobial therapy for lower RTIs,41 and may be useful for ruling out bacterial coinfections.42 However, a clear cutoff between bacterial and viral pneumonia remains to be elucidated,43 and interpretation is limited among some patient populations (eg, those needing hemodialysis).44 In the future, other promising biomarker approaches assessing host gene expression to categorize acute RTIs as either viral or bacterial may become more readily available for ASPs.45 Nonetheless, when ordering respiratory diagnostic assays, it is important to consider whether the test will affect clinical management. For example, influenza is the only respiratory virus of which treatment is available, suggesting identification by NAAT can facilitate prompt initiation or discontinuation of anti-influenza therapy during influenza season. However, ordering the same test for a mildly ill patient not expected to receive treatment, or during a period of low prevalence, does not provide value. Of note, NAAT is favored over traditional influenza antigen–based
diagnostic tests, as they are significantly more sensitive, resulting in more accurate and usable results.46 More recently, multiplex PCR panels for respiratory viruses have become more common, and are able to optimize anti-influenza therapy.47 Clinical and economic outcomes vary across respiratory diagnostic studies, largely due to heterogeneity and variability in the quality of currently available data. Nonetheless, reduced antimicrobial utilization with rapid viral panels has been described among pediatric48 and adult patients.49 Overall, respiratory pathogen panels play an important role in RTI diagnostics. Novel bacterial panels have become available, but clinical outcomes have yet to be assessed. The IDSA Diagnostics Committee recently released clinical and diagnostic recommendations for management of acute RTIs.50 When bacterial pneumonia is diagnosed in patients with risk factors for resistance or CNS infections require at high risk for mortality, grampositive coverage for MRSA is prompt diagnostic often empirically initiated. Because S. aureus is a pathogen routinely identifi cation and implicated in pneumonia, and a common colonizer of the nares, a antibiotics, if MRSA nasal PCR can be used to appropriate. help discontinue unnecessary vancomycin. A meta-analysis of 22 studies and 5,163 patients idenSource: Clin Infect Dis. 2004;39(9):1267-1284. tified a positive predictive value of 44.8% and negative predictive value of 96.5% using 10% prevalence,51 indicating a negative result can be particularly useful to rule out MRSA pneumonia thereby decreasing days of vancomycin therapy, especially among patients who are not critically ill. ASPs can use a combination of these RDTs in conjunction with a patient’s clinical picture to help ensure that those who require antibiotics are treated effectively and those who have solely viral infections are spared unnecessary antimicrobials.
Central Nervous System
CNS infections are medical emergencies that are associated with considerable mortality and rapid decline that requires prompt diagnostic identification and antimicrobial administration.52 Diagnostic testing for CNS infections is particularly challenging, as more than 20 available diagnostic tests exist and various testing combinations are ordered in clinical practice.53 ASPs are incredibly important for such scenarios, as they can help guide medical teams, especially when a limited amount of cerebrospinal fluid (CSF) volume is obtained. Low-volume yield of CSF during lumbar puncture is commonly seen, especially among pediatric patients. Identifying viral etiologies by PCR can facilitate diagnostics, reduce hospital LOS, and reduce duration of unnecessary antimicrobial therapy.54 However, rapid
bacterial identification expedites appropriate antimicrobial utilization and improves clinical outcomes.55,56
The only clinically used, FDA-approved rapid panel is the BioFire FilmArray meningitis/encephalitis (ME) panel (BioFire Diagnostics), with a turnaround time of 1 hour and only 2 minutes of hands-on time. It is a nucleic acid–based panel that requires a small volume of CSF (0.2 mL), and has 14 total targets, including E. coli (K1 serotype only), Haemophilus influenzae, Listeria monocytogenes, Neisseria meningitidis (encapsulated strains only), Streptococcus agalactiae, Streptococcus pneumoniae, Cryptococcus neoformans/gattii, cytomegalovirus, enteroviruses, herpes simplex viruses 1 and 2, human herpesvirus 6, human parechovirus, and varicella-zoster virus.57 In a study of 1,560 CSF samples, the ME panel established an 84.4% positive and greater than 99% negative agreement to traditional testing methods.56,58 The ME panel also results in a faster time to diagnosis by 10.3 hours compared with pathogen-specific PCR testing.54 There have been reports of both false-positive and false-negative results leading to concerns for institutions when deciding on implementation. A recent meta-analysis was performed to determine the sensitivity and specificity of the ME panel.59 The investigators pooled data from 8 studies (N=3,059) and reported a sensitivity and specificity with confidence intervals of 90% (95% CI, 86%-93%) and 97% (95% CI, 94%-99%), respectively. The highest proportion of false positives was associated with S. pneumoniae followed by S. agalactiae, and the highest proportion of false negatives was for herpes simplex viruses 1 and 2, enteroviruses, and C. neoformans/gattii. Despite promising potential, the ME panel cannot yet replace traditional testing methods for ME diagnosis, and knowledge of limitations is essential for utilization.56,58 It may be particularly helpful in hospitals with high CNS infection prevalence (eg, pediatric and neonatal patients).
GI Infection Cultures
Acute gastroenteritis is a leading cause of morbidity and mortality and responsible for an estimated 47.8 million episodes annually in the United States.60,61 Gastroenteritis is characterized by acute onset of diarrhea with or without vomiting and caused by a wide variety of bacteria, viruses, and parasites as well as other noninfectious cases. Identification of an infectious agent is critical for patient care and infection prevention practices; however, the etiologic pathogen goes unidentified in approximately 80% of cases.60,62,63
Conventional methods of identification (eg, antigen testing, microscopic examinations, and culture) are time-consuming, expensive, and have limited sensitivity.64 Multiplex PCR-based GI panels, such as the FilmArray, have emerged as a more rapid and accurate diagnostic tool to diagnose gastroenteritis, although clinical outcomes data are limited. The BioFire FilmArray GI panel combines 22 enteric pathogens into a single cartridge-based test with a turnaround time of less than 2 hours. Assay performance was assessed and demonstrated 100% and 94.5% or higher sensitivity for 12 of 22 and 7 of 22 targets, respectively, and 97.1% or higher specificity for all targets.65
Although symptoms are often self-limiting, some patients may benefit from antimicrobials and experience delays in therapy due to lengthy testing methods. Hospitalization and further testing such as colonoscopy or abdominal ultrasonography may be required in patients with multiple comorbidities or severe illness pending test results, leading to unnecessary patient isolation and more extensive infection prevention practices. Beal et al evaluated the clinical and economic impact of the FilmArray GI panel compared with historical controls using traditional stool culture testing methodology.66 The positivity rate of the GI panel was 32.8% compared with 6.7% for the historical controls. The GI panel resulted in faster results reported in the electronic health record (8.94 vs 54.75 hours), less additional stool tests ordered overall (0.58 vs 3.02 additional tests; P=0.0001), fewer antibiotic days per patient (1.73 [95% CI, 1.41-2.04] and 2.12 [95% CI, 1.892.35] days per patient; P=0.06), fewer imaging studies (0.18 vs 0.39 imaging studies per patient; P=0.0002), shorter LOS (5.2±3.2 vs 5.6±3.4 days; P=0.14), and a cost savings of approximately $294 per patient.
A similar rate of positivity was observed in another study comparing the FilmArray GI panel with historical stool culture controls (29.2% vs 4.1%, respectively).67 Clinical outcomes were also improved with the GI panel; patients were less likely to undergo endoscopy (8.4% vs 9.6%; P=0.008) or abdominal radiology (29.4% vs 31%; P=0.002) and less likely to receive antimicrobials (36.2% vs 40.9%; P<0.001).67
The prevalence of GI coinfection in the United States is not well known compared with developing countries; however, the FilmArray GI panel commonly detects coinfection in up to 32.9%.59-61,65-67 This may be because the PCR is not able to differentiate between asymptomatic colonization and infection.
Conclusion
The development and implementation of RDTs have significantly augmented ASPs and improved clinical outcomes for patients with suspected or confirmed infections. When combined with stewardship initiatives, RDTs improve the timeliness and effectiveness of antimicrobial optimization. Although outcomes data are most substantive for BSIs, RTIs, and CNS infections, GI panels are offering advances for patients suffering from these conditions. Awareness of the benefits they may provide, along with a critical assessment of institutional needs, can optimize patient care while minimizing harm associated with inappropriate antimicrobial utilization.
References
1. Evans L, Rhodes A, Alhazzani W, et al. Surviving Sepsis Campaign: international guidelines for management of sepsis and septic shock 2021. Crit Care Med. 2021;49(11):1974-1982.
2. Kumar A, Ellis P, Arabi Y, et al. Initiation of inappropriate antimicrobial therapy results in a fivefold reduction of survival in human septic shock. Chest. 2009;136(5):1237-1248.
3. Ibrahim EH, Sherman G, Ward S, et al. The influence of inadequate antimicrobial treatment of bloodstream infections on patient outcomes in the ICU setting. Chest. 2000;118(1):146-155.
4. Kumar A, Roberts D, Wood KE, et al. Duration of hypotension before initiation of effective antimicrobial therapy is the critical determinant of survival in human septic shock. Crit Care Med. 2006;34(6):1589-1596.
5. Paul M, Kariv G, Goldberg E, et al. Importance of appropriate empirical antibiotic therapy for methicillin-resistant Staphylococcus aureus bacteraemia. J Antimicrob Chemother. 2010;65(12):2658-2665.
6. Paul M, Shani V, Muchtar E, et al. Systematic review and metaanalysis of the efficacy of appropriate empiric antibiotic therapy for sepsis. Antimicrob Agents Chemother. 2010;54(11):4851-4863.
7. Lodise TP, Berger A, Altincatal A, et al. Antimicrobial resistance or delayed appropriate therapy—does one influence outcomes more than the other among patients with serious infections due to carbapenem-resistant versus carbapenem-susceptible Enterobacteriaceae? Open Forum Infect Dis. 2019;6(6):ofz194.
8. Zasowski EJ, Claeys KC, Lagnf AM, et al. Time is of the essence: the impact of delayed antibiotic therapy on patient outcomes in hospital-onset enterococcal bloodstream infections. Clin Infect
Dis. 2016;62(10):1242-1250.
9. Beganovic M, Timbrook TT, Wieczorkiewicz SM. Predictors of time to effective and optimal antimicrobial therapy in patients with positive blood cultures identified via molecular rapid diagnostic testing. Open Forum Infect Dis. 2019;6(1):ofy350.
10. Timbrook TT, Morton JB, McConeghy KW, et al. The effect of molecular rapid diagnostic testing on clinical outcomes in bloodstream infections: a systematic review and meta-analysis.
Clin Infect Dis. 2017;64(1):15-23.
11. Pliakos EE, Andreatos N, Shehadeh F, et al. The cost-effectiveness of rapid diagnostic testing for the diagnosis of bloodstream infections with or without antimicrobial stewardship. Clin
Microbiol Rev. 2018;31(3):e00095-17.
12. Messacar K, Parker SK, Todd JK, et al. Implementation of rapid molecular infectious disease diagnostics: the role of diagnostic and antimicrobial stewardship. J Clin Microbiol. 2017;55(3):715-723.
13. Barlam TF, Cosgrove SE, Abbo LM, et al. Executive summary: implementing an antibiotic stewardship program: guidelines by the Infectious Diseases Society of America and the Society for Healthcare Epidemiology of America. Clin Infect Dis. 2016;62(10):1197-1202.
14. Wenzler E, Timbrook TT, Wong JR, et al. Implementation and optimization of molecular rapid diagnostics in bloodstream infections: a clinical review. Am J Health Syst Pharm. 2018;75(16):1191-1202.
15. Aitken SL, Hemmige VS, Koo HL, et al. Real-world performance of a microarray-based rapid diagnostic for gram-positive bloodstream infections and potential utility for antimicrobial stewardship. Diagn Microbiol Infect Dis. 2015;81(1):4-8.
16. Wong JR, Bauer KA, Mangino JE, et al. Antimicrobial stewardship pharmacist interventions for coagulase-negative staphylococci positive blood cultures using rapid polymerase chain reaction.
Ann Pharmacother. 2012;46(11):1484-1490.
17. Nagel JL, Huang AM, Kunapuli A, et al. Impact of antimicrobial stewardship intervention on coagulase-negative Staphylococcus blood cultures in conjunction with rapid diagnostic testing. J Clin Microbiol. 2014;52(8):2849-2854.
18. Wenzler E, Wang F, Goff DA, et al. An automated, pharmacistdriven initiative improves quality of care for Staphylococcus aureus bacteremia. Clin Infect Dis. 2017;65(2):194-200.
19. Bookstaver PB, Nimmich EB, Smith TJ 3rd, et al. Cumulative effect of an antimicrobial stewardship and rapid diagnostic testing bundle on early streamlining of antimicrobial therapy in gram-negative bloodstream infections. Antimicrob Agents
Chemother. 2017;61(9):e00189-17.
20. Pogue JM, Heil EL, Lephart P, et al. An antibiotic stewardship program blueprint for optimizing Verigene BC-GN within an
Institution: a tale of two cities. Antimicrob Agents Chemother. 2018;62(5):e02538-17.
21. Rivard KR, Athans V, Lam SW, et al. Impact of antimicrobial stewardship and rapid microarray testing on patients with gram-negative bacteremia. Eur J Clin Microbiol Infect Dis. 2017;36(10):1879-1887.
22. Walker T, Dumadag S, Lee CJ, et al. Clinical impact of laboratory implementation of Verigene BC-GN Microarray-based assay for detection of gram-negative bacteria in positive blood cultures.
J Clin Microbiol. 2016;54(7):1789-1796.
23. Huang AM, Newton D, Kunapuli A, et al. Impact of rapid organism identification via matrix-assisted laser desorption/ionization time-of-flight combined with antimicrobial stewardship team intervention in adult patients with bacteremia and candidemia.
Clin Infect Dis. 2013;57(9):1237-1245.
24. Pfaller MA, Wolk DM, Lowery TJ. T2MR and T2Candida: novel technology for the rapid diagnosis of candidemia and invasive candidiasis. Future Microbiol. 2016;11(1):103-117.
25. Pappas PG, Kauffman CA, Andes DR, et al. Clinical Practice
Guideline for the Management of Candidiasis: 2016 Update by the Infectious Diseases Society of America. Clin Infect Dis. 2016;62(4):e1-e50.
26. Wilson NM, Alangden G, Tibbetts RJ, et al. T2 magnetic resonance assay improves timely management of candidemia.
J Antimicrob Stewardship. 2017;1(1):12-18.
27. Patch ME, Weisz E, Cubillos A, et al. Impact of rapid, cultureindependent diagnosis of candidaemia and invasive candidiasis in a community health system. J Antimicrob Chemother. 2018;73(suppl 4):iv27-iv30.
28. De Angelis G, Posteraro B, De Carolis E, et al. T2Bacteria magnetic resonance assay for the rapid detection of ESKAPEc pathogens directly in whole blood. J Antimicrob Chemother. 2018;73(suppl 4):iv20-iv6.
29. Nguyen MH, Clancy CJ, Pasculle AW, et al. Performance of the
T2Bacteria Panel for diagnosing bloodstream infections: a diagnostic accuracy study. Ann Intern Med. 2019;170(12):845-852.
30. Drevinek P, Hurych J, Antuskova M, et al. Direct detection of
ESKAPEc pathogens from whole blood using the T2Bacteria
Panel allows early antimicrobial stewardship intervention in patients with sepsis. Microbiologyopen. 2021;10(3):e1210.
31. Perez KK, Olsen RJ, Musick WL, et al. Integrating rapid diagnostics and antimicrobial stewardship improves outcomes in patients with antibiotic-resistant gram-negative bacteremia.
J Infect. 2014;69(3):216-225.
32. Kerremans JJ, Verboom P, Stijnen T, et al. Rapid identification and antimicrobial susceptibility testing reduce antibiotic use and accelerate pathogen-directed antibiotic use. J Antimicrob
Chemother. 2008;61(2):428-435.
33. Lodise TP Jr, Lomaestro B, Drusano GL. Piperacillin-tazobactam for Pseudomonas aeruginosa infection: clinical implications of an extended-infusion dosing strategy. Clin Infect Dis. 2007;44(3):357-363.
34. Roberts JA, Paul SK, Akova M, et al. DALI: defining antibiotic levels in intensive care unit patients: are current beta-lactam antibiotic doses sufficient for critically ill patients? Clin Infect Dis. 2014;58(8):1072-1083.
35. Banerjee R, Komarow L, Virk A, et al. Randomized trial evaluating clinical impact of RAPid IDentification and susceptibility testing for gram negative bacteremia: RAPIDS-GN. Clin Infect Dis. 2021;73(1):e39-e46.
36. Dare RK, Lusardi K, Pearson C, et al. Clincal Impact of
Accelerate Pheno Rapid Blood Culture Detection System in bacteremic patients. Clin Infect Dis. 2021;73(11):e4616-e4626.
37. Goggin KP, Gonzalez-Pena V, Inaba Y, et al. Evaluation of plasma microbial cell-free DNA sequencing to predict bloodstream infection in pediatric patients with relapsed or refractory cancer.
JAMA Oncol. 2019;6(4):552-556.
38. Hogan CA, Yang S, Garner OB, et al. Clinical impact of metagenomic next-generation sequencing of plasma cell-free DNA for the diagnosis of infectious diseases: a multicenter retrospective cohort study. Clin Infect Dis. 2021;72(2):239-245.
39. Benamu E, Gajurel K, Anderson JN, et al. Plasma microbial cellfree DNA next generation sequencing in the diagnosis and management of febrile neutropenia. Clin Infect Dis. 2021;ciab324. doi:10.1093/cid/ciab324
40. Fleming-Dutra KE, Hersh AL, Shapiro DJ, et al. Prevalence of inappropriate antibiotic prescriptions among US ambulatory care visits, 2010-2011. JAMA. 2016;315(17):1864-1873.
41. Schuetz P, Wirz Y, Sager R, et al. Effect of procalcitonin-guided antibiotic treatment on mortality in acute respiratory infections: a patient level meta-analysis. Lancet Infect Dis. 2018;18(1):95-107.
42. Timbrook T, Maxam M, Bosso J. Antibiotic discontinuation rates associated with positive respiratory viral panel and low procalcitonin results in proven or suspected respiratory infections. Infect
Dis Ther. 2015;4(3):297-306.
43. Self WH, Balk RA, Grijalva CG, et al. Procalcitonin as a marker of etiology in adults hospitalized with community-acquired pneumonia. Clin Infect Dis. 2017;65(2):183-190.
44. Grace E, Turner RM. Use of procalcitonin in patients with various degrees of chronic kidney disease including renal replacement therapy. Clin Infect Dis. 2014;59(12):1761-1767.
45. Tsalik EL, Henao R, Nichols M, et al. Host gene expression classifiers diagnose acute respiratory illness etiology. Sci Transl Med. 2016;8(322):322ra11.
46. Vemula SV, Zhao J, Liu J, et al. Current approaches for diagnosis of influenza virus infections in humans. Viruses. 2016;8(4):96.
47. Green DA, Hitoaliaj L, Kotansky B, et al. Clinical utility of ondemand multiplex respiratory pathogen testing among adult outpatients. J Clin Microbiol. 2016;54(12):2950-2955.
48. Doan Q, Enarson P, Kissoon N, et al. Rapid viral diagnosis for acute febrile respiratory illness in children in the emergency department. Cochrane Database Syst Rev. 2014;(9):CD006452.
49. Brendish NJ, Malachira AK, Armstrong L, et al. Routine molecular point-of-care testing for respiratory viruses in adults presenting to hospital with acute respiratory illness (ResPOC): a pragmatic, open-label, randomised controlled trial. Lancet Respir Med. 2017;5(5):401-411.
50. Hanson KE, Azar MM, Banerjee R, et al. Molecular testing for acute respiratory tract infections: clinical and diagnostic recommendations from the IDSA’s Diagnostics Committee. Clin Infect
Dis. 2020;71(10):2744-2751.
51. Parente DM, Cunha CB, Mylonakis E, et al. The clinical utility of methicillin-resistant Staphylococcus aureus (MRSA) nasal screening to rule out MRSA pneumonia: a diagnostic meta-analysis with antimicrobial stewardship implications. Clin Infect Dis. 2018;67(1):1-7. 52. Tunkel AR, Hartman BJ, Kaplan SL, et al. Practice guidelines for the management of bacterial meningitis. Clin Infect Dis. 2004;39(9):1267-1284.
53. Wootton SH, Aguilera E, Salazar L, et al. Enhancing pathogen identification in patients with meningitis and a negative Gram stain using the BioFire FilmArray Meningitis/Encephalitis panel.
Ann Clin Microbiol Antimicrob. 2016;15:26.
54. Messacar K, Breazeale G, Robinson CC, et al. Potential clinical impact of the film array meningitis encephalitis panel in children with suspected central nervous system infections. Diagn Microbiol Infect Dis. 2016;86(1):118-120.
55. Blaschke AJ, Holmberg KM, Daly JA, et al. Retrospective evaluation of infants aged 1 to 60 days with residual cerebrospinal fluid (CSF) tested using the FilmArray Meningitis/Encephalitis (ME) panel. J Clin Microbiol. 2018;56(7):e00277-18.
56. Leber AL, Everhart K, Balada-Llasat JM, et al. Multicenter evaluation of BioFire FilmArray meningitis/encephalitis panel for detection of bacteria, viruses, and yeast in cerebrospinal fluid specimens. J Clin Microbiol. 2016;54(9):2251-2261.
57. He T, Kaplan S, Kamboj M, et al. Laboratory diagnosis of central nervous system infection. Curr Infect Dis Rep. 2016;18(11):35.
58. Hanson KE, Couturier MR. Multiplexed molecular diagnostics for respiratory, gastrointestinal, and central nervous system infections. Clin Infect Dis. 2016;63(10):1361-1367.
59. Tansarli GS, Chapin KC. Diagnostic test accuracy of the BioFire
FilmArray meningitis/encephalitis panel: a systematic review and meta-analysis. Clin Microbiol Infect. 2020;26(3):281-290.
60. Scallan E, Griffin PM, Angulo FJ, et al. Foodborne illness acquired in the United States—unspecified agents. Emerg Infect Dis. 2011;17(1):16-22.
61. Scallan E, Hoekstra RM, Angulo FJ, et al. Foodborne illness acquired in the United States—major pathogens. Emerg Infect
Dis. 2011;17(1):7-15.
62. Axelrad JE, Joelson A, Nobel Y, et al. The distribution of enteric infections utilizing stool microbial polymerase chain reaction testing in clinical practice. Dig Dis Sci. 2018;63(7):1900-1909.
63. Axelrad JE, Joelson A, Nobel YR, et al. Enteric infection in relapse of inflammatory bowel disease: the utility of stool microbial PCR testing. Inflamm Bowel Dis. 2017;23(6):1034-1039.
64. Riddle MS, DuPont HL, Connor BA. ACG clinical guideline: diagnosis, treatment, and prevention of acute diarrheal infections in adults. Am J Gastroenterol. 2016;111(5):602-622.
65. Buss SN, Leber A, Chapin K, et al. Multicenter evaluation of the
BioFire FilmArray gastrointestinal panel for etiologic diagnosis of infectious gastroenteritis. J Clin Microbiol. 2015;53(3):915-925.
66. Beal SG, Tremblay EE, Toffel S, et al. A gastrointestinal PCR panel improves clinical management and lowers health care costs.
J Clin Microbiol. 2018;56(1):e01457-17.
67. Axelrad JE, Freedberg DE, Whittier S, et al. Impact of gastrointestinal panel implementation on health care utilization and outcomes. J Clin Microbiol. 2019;57(3):e01775-18.
68. Beganovic M, McCreary EK, Mahoney MV, et al. Interplay between rapid diagnostic tests and antimicrobial stewardship programs among patients with bloodstream and other severe infections.
J Appl Lab Med. 2019;3(4):601-616.
About the author
Sarah M. Wieczorkiewicz, PharmD,
FIDSA, BCPS, BCIDP, is an infectious diseases clinical specialist in Chicago, Illinois, and has 15 years of clinical experience.