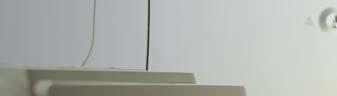
32 minute read
Rapid Diagnostic Methods and Technologies in the Management of Infectious Diseases
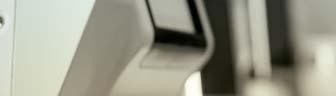

BY KAREN FONG, PHARMD, BCIDP
In the realm of infectious diseases management, rapid diagnostic tests (RDTs) have modernized the field profoundly by providing microbiological diagnoses more quickly and robustly. Modern molecular techniques include direct-specimen rapid amplification and detection platforms and next-generation sequencing, which provide more rapid, sensitive, and comprehensive laboratory diagnoses never before possible compared with conventional microbiology.1 RDTs have revolutionized the microbiological diagnostic pathway, serving as the cornerstone for pathogen identification and resistance testing.
Despite significant progress in diagnostic technologies, broad-spectrum antimicrobials continue to be heavily overused during empiric treatment of infectious disease syndromes.2,3 The detection of genotypic markers of resistance has been made available through newer technologies, but rapid phenotypic antimicrobial susceptibility testing (AST) has been accessible only recently. Genotypic (nucleic acid-based) methods are limited by detecting only searchable resistance patterns and potentially finding resistance genes that are not necessarily from the pathogenic organism.4 According to the Clinical Laboratory Standards Institute (CLSI) and European Committee on Antimicrobial Susceptibility Testing (EUCAST) guidelines, reliable antibiotic resistance diagnostics requires phenotypic testing. Thus, these commercial advances are not yet a replacement for bacterial and fungal cultures, but could provide information to the clinical presentation and support empiric antimicrobial selection by facilitating the anticipation of susceptibility patterns based on local antibiograms.1
Classic AST techniques include broth microdilution, disk diffusion, gradient tests, agar dilution and breakpoint tests, which are based on continuous exposure of a bacterial isolate to a set of
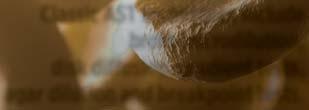
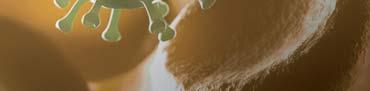
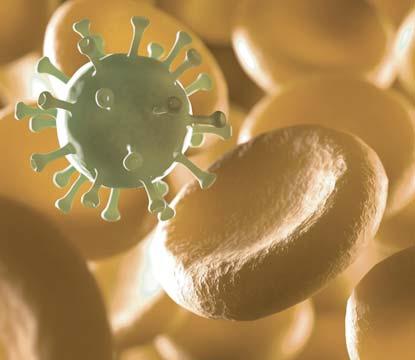
antimicrobials, followed by visual detection of growth. For conventional growth-based AST, several cultivation rounds are required, including enrichment cultivations (ie, blood specimens) to increase the quantity of bacteria, plate cultivations to acquire pure cultures, identification with matrix-assisted laser desorption/ ionization time of flight (MALDI-TOF) mass spectrometry if available, and finally AST and minimum inhibitory concentration (MIC) determination, according to CLSI or EUCAST standards. This entire process may require up to several days for complete results.4 Commercial systems like VITEK 2 (bioMérieux) and BD Phoenix have streamlined and partly automated the follow-up of AST cultures, with turnaround times as short as 2 hours for identification and 4 to 8 hours for susceptibility testing.5-7 These systems use a fluorogenic methodology for organism identification and a turbidimetric method for susceptibility testing. Standardized AST cassettes containing positive controls and wells with increasing concentrations of antibiotic continuously monitor growth and analyze MIC patterns for a large group of organisms through their extensive databases.
The effectiveness of RDTs in the diagnosis and treatment of infectious disease syndromes depends on the benefits and limitations of the methods and compatibility with clinical practice, including antimicrobial stewardship programs (ASPs). In this review, we discuss diagnostic methods and technologies that enable rapid identification and AST for blood culture testing, respiratory infections, and outpatient point of care with current antimicrobial stewardship practices. See the Table for a synopsis of RDT samples, method, specimen, turnaround time, pathogens, and resistance markers.
Blood
For bloodstream infections (BSIs) and blood culture contaminants (eg, coagulase-negative staphylococci), MALDI-TOF (eg, bioMérieux, BD Bruker), polymerase chain reaction (PCR)-based technologies (eg, BioFire FilmArray BCID, GenMark ePlex BCID), and nanoparticle probe technology (eg, Verigene BC-GP and BC-GN) are molecular RDTs that have changed management fundamentally by providing identification much earlier in the course of empiric treatment compared with conventional microbiology cultures.
Organism identification using MALDI-TOF is a routine diagnostic component for infectious diseases in current clinical laboratories, first successfully introduced in the early 2000s.8 The analytical strategy using this technique is to reflect the composite proteome of a bacterial cell.9 Bacterial and fungal colonies are applied directly onto a MALDI target plate with matrices for cocrystallizations. Analytes are cocrystallized within the matrix, and then a laser beam provides energy to ionize the analyte to generate separate protonated ions. These ions are accelerated at a fixed potential and separated from one another based on their weights when they fly along the separation tube. The flight time of each ion is measured at the detection panel placed at the end of the flight tube.8 A characteristic spectrum called peptide mass fingerprint (PMF) is generated for the analytes. Identification of the organisms is completed by comparing the PMF of the unknown organism with the PMFs contained in the database or by matching the masses of biomarkers of the unknown organism with the proteome database.9 MALDI-TOF has become the standard protocol for the identification of bacterial and fungal species based on its speed (<1 minute per sample), accurate identification of clinically relevant pathogens, and cost-effectiveness.8
The BioFire FilmArray BCID2 Panel is a multiplexed nucleic acid test performed directly on positive blood culture samples for the simultaneous qualitative detection and identification of multiple bacterial and yeast nucleic acids and selected genetic determinants associated with antimicrobial resistance. This test has an approximate turnaround time of 1 hour. There are 4 main steps when running the BioFire System: 1. lysing the sample by agitation (bead beating) in addition to chemical lysis mediated by the sample buffer; 2. extracting and purifying all nucleic acids from the sample using magnetic bead technology; 3. conducting nested multiplex PCR by performing a single, large-volume, massively multiplex reaction (PCR1) and then performing multiple singleplex second-stage PCR reactions (PCR2) to amplify
sequences within the PCR1 products; and 4. using end point melting curve data to detect and generate a result for reach target on the BioFire
BCID2 Panel array.10
Similarly, the ePlex BCID Panels are automated, qualitative multiplex nucleic acid in vitro diagnostic tests for the simultaneous detection and identification of gram-positive, gram-negative, and fungal pathogens as well as antibiotic resistance genes in positive blood cultures in approximately 1.5 hours. This test uses electrowetting to perform multiplex nucleic acid extraction, amplification, and digestion, and Genmark’s eSensor technology to detect analyte targets.11,12 Finally, Verigene BC-GP and BC-GN use nanogrid technology, a unique gold nanoparticle probe chemistry, which is the driving force behind its platform with a turnaround time of 2.5 hours. Identification of causative pathogens is accompanied by their associated resistance markers. Each nanoparticle is functionalized with either a defined number of oligonucleotides (ie, short pieces of DNA or RNA) or a defined number of antibodies that are specific to a particular protein of interest. The detection of DNA or RNA targets on the Verigene System involves automating nucleic acid extraction and PCR amplification (if necessary) from positive blood culture, automating transfer of eluted nucleic acids for hybridization, primary hybridization of target DNA to capture oligonucleotides on a microarray, secondary hybridization of specific mediator oligonucleotides and gold nanoparticle probes, signal amplification of hybridized probes via a silver staining process, and automated qualitative analysis of results.13
These platforms for BSIs have been associated with decreases in time to effective therapy, hospital length of stay (LOS), and mortality when paired with actionable ASP interventions.14 Benefits of molecular RDTs in BSIs appear cost effective.15 There is a robust synergism between antimicrobial stewardship and RDT in these data, with an 80% chance of cost-effectiveness but only 41.1% in the absence of ASP.
The Accelerate Pheno system from Accelerate Diagnostics has introduced further potential changes in the management of BSIs through an automated rapid phenotypic testing system. This system can yield organism identification and susceptibility interpretation with an MIC, and turnaround time of approximately 2 hours and 7 hours after positive blood culture, respectively. The platform uses automated fluorescence in situ hybridization technology with morphokinetic cellular analysis—a method that tracks, analyzes, and interprets different morphology and kinetic data to determine mass, division rate, anomalous growth patterns, and even detect heterogeneity.16,17
A randomized controlled trial of patients with gramnegative BSIs combined with prospective audit and feedback demonstrated significantly faster antibiotic changes (median decrease, 25 hours for gram-negative antibiotics; P<0.001) and antibiotic escalation (median decrease, 43 hours; P=0.01) with Accelerate Pheno, but there were no differences in clinical outcomes, including mortality and hospital LOS, compared with culture-based methods.18 Five quasiexperimental before-and-after observational studies, analyzing the integration of Accelerate Pheno with ASP intervention, had variable results in patient outcomes. Although median time to optimal or targeted therapy and step-down antimicrobial therapy were significantly shorter with the use of Accelerate Pheno, LOS and duration of therapy (DOT) were not consistently shorter despite ASP intervention.19-24 Nevertheless, Accelerate Pheno seemed to have no benefit on mortality compared with culture-based methods.18-24 Future studies on a larger scale with ASP intervention are needed to assess its impact on clinical outcomes.
The T2Candida Panel and T2Bacteria Panel by T2 Biosystems are 2 nonculture diagnostic tests that use T2 magnetic resonance (T2MR) to identify pathogens in whole blood samples by directly detecting DNA from multiple species of Candida and bacteria, respectively, without the need for cultivating organisms. This platform amplifies microbial cell-associated DNA using a thermostable polymerase and target-specific primers, and detects signals by amplicon-induced agglomeration of superparamagnetic particles and T2MR.25
Candidemia, one of the most common hospital-acquired BSIs in the United States, is associated with high mortality, especially among patients who develop septic shock. Prompt initiation of appropriate antifungal therapy and source control are limited by blood culture insensitivity, prolonged turnaround time (median time to positivity, 2-3 days; range, 1 to ≥7 days) needed to yield growth, and the possibility of negative growth with invasive abdominal candidiasis.26 This delay has led to an overuse of empiric antifungal therapy for suspected invasive candidiasis, a practice of unproven clinical value.27
The T2Candida Panel (T2 Biosystems) is a nonculture diagnostic test with a much shorter turnaround time (3-5 hours), and has entered clinical practice as an adjunctive RDT to cultures.26,28,29 While sensitivity and specificity seem to be much more promising compared with blood cultures, the role of the T2Candida Panel in the early diagnosis and management of candidemia remains unclear.26,29 Based on retrospective, single-center cohort studies, the T2Candida Panel decreased time to appropriate therapy in patients with proven candidemia while shorter antifungal DOT and cost savings were observed in patients without microbiological evidence of invasive candidiasis. However, antifungal discontinuation with negative tests has been inconsistent despite antimicrobial stewardship interventions such as prospective audit and feedback being performed on negative results.30-32 The
Sample of RDTs With Turnaround Time and Pathogens/Resistance Markers Table.
Method Specimen Turnaround time Pathogens/resistance markers
<1 min All organisms Enterococcus faecalis, Enterococcus faecium, Listeria monocytogenes, Staphylococcus spp, Staphylococcus aureus, Staphylococcus epidermidis, Staphylococcus lugdunensis, Streptococcus spp, Streptococcus agalactiae, Streptococcus pneumoniae, Streptococcus pyogenes, Acinetobacter baumannii, Bacteroides fragilis, Escherichia coli, Enterobacter cloacae complex, Haemophilus influenzae, Klebsiella aerogenes, Klebsiella oxytoca, Klebsiella pneumoniae, Neisseria meningitidis, Proteus spp, Pseudomonas aeruginosa, Salmonella, Serratia marcescens, Stenotrophomonas maltophilia, Candida albicans, Candida auris, Candida glabrata, Candida krusei, Candida parapsilosis, Candida tropicalis, Cryptococcus neoformans Resistance markers: mecA, mecC, vanA, vanA/B, CTX-M, IMP, KPC, mcr-1, NDM, OXA-48-like, OXA
1 h
Pure culture
Mass spectrometry Positive blood culture
mPCR
Blood MALDI-TOF (bioMérieux, Brucker) FilmArray Blood Culture Identification
(BioFire) Bacillus cereus group, Bacillus subtilis group, Corynebacterium, Cutibacterium acnes, Enterococcus spp, E. faecalis, E. faecium, Lactobacillus, Listeria spp, L. monocytogenes, Micrococcus spp, Staphylococcus spp, S. aureus, S. epidermidis, S. lugdunensis, Streptococcus spp, S. agalactiae, Streptococcus anginosus, S. pneumoniae, S. pyogenes, A. baumannii, B. fragilis, Citrobacter spp, Cronobacter sakazakii, E. coli, E. cloacae complex, Enterobacter (non-cloacae complex), Fusobacterium necrophorum, Fusobacterium nucleatum, H. influenzae, K. oxytoca, K. pneumoniae, Morganella morganii, N. meningitidis, Proteus spp, Proteus mirabilis, P. aeruginosa, Salmonella, Serratia spp, S. marcescens, S. maltophilia, C. albicans, C. auris, Candida dubliniensis, Candida famata, guilliermondii, Candida kefyr, C. krusei, Candida lusitaniae, C. glabrata, Candida C. parapsilosis, C. tropicalis, Cryptococcus gattii, C. neoformans, Fusarium, Rhodotorula Resistance markers: mecA, mecC, vanA, vanA/B, CTX-M, IMP, KPC, NDM, OXA, VIM E. faecalis, E. faecium, Listeria spp, Micrococcus spp, Staphylococcus spp, S. aureus, S. epidermidis, S. lugdunensis, Streptococcus spp, S. agalactiae, S. anginosus, S. pneumoniae, S. pyogenes, Acinetobacter spp, Citrobacter spp, E. coli, Enterobacter spp, K. oxytoca, K. pneumoniae, Proteus spp, P. aeruginosa, S. marcescens Resistance markers: mecA, vanA, vanA/B, CTX-M, IMP, KPC, NDM, OXA, VIM
1.5 h 2.5 h
Positive blood culture Positive blood culture E. faecalis, E. faecium, Staphylococcus spp, S. aureus, S. lugdunensis, Streptococcus spp, A. baumannii, Citrobacter spp, E. coli, Enterobacter spp, Klebsiella spp, Proteus spp, P. aeruginosa, S. marcescens, C. albicans, C. glabrata
Identification: 2 h Susceptibility: 7 h
Positive blood culture E. faecium, S. aureus, E. coli, K. pneumoniae, P. aeruginosa
3-5 h C. albicans, C. glabrata, C. krusei, C. parapsilosis, C. tropicalis
3-5 h 1 d 1,250 bacteria, fungi, parasites, and viruses
Whole blood Whole blood Whole blood
mPCR, electrowetting, eSensor technology mPCR, gold nanoparticle technology Fluorescence- labeled nucleic acid probe, morphokinetic cellular analysis mPCR, magnetic resonance mPCR, magnetic resonance Next-generation sequencing
ePlex Blood Culture Identification
(GenMark)
Verigene Blood Culture Test
(Luminex)
Accelerate Pheno
(Accelerate Diagnostics)
T2Bacteria Panel
(T2 Biosystems)
T2Candida Panel
(T2 Biosystems)
Karius Test
Adenovirus; coronavirus types 229E, HKU1, NL63, and OC43; SARS-CoV-2; human metapneumovirus; human rhinovirus/enterovirus; influenza A, including subtypes H1, H3, and H1-2009; influenza B; parainfluenza virus types 1-4; respiratory syncytial virus; Bordetella parapertussis; Bordetella pertussis; Chlamydia pneumoniae; Mycoplasma pneumoniae Adenovirus groups B, C, and E; coronavirus types 229E, HKU1, OC43, and NL63; influenza A virus subtypes; influenza B virus; human metapneumovirus; parainfluenza virus types 1-4; respiratory syncytial virus types A and B; and rhinovirus Adenovirus; coronavirus types 229E, HKU1, NL63, and OC43; SARS-CoV-2; metapneumovirus; rhinovirus/enterovirus; influenza A; influenza A H1; influenza AvH1- 2009; Influenza A H3; influenza B; parainfluenza types 1-4; respiratory syncytial virus A and B; C. pneumoniae, M. pneumoniae Adenovirus, human metapneumovirus, influenza A subtypes, influenza B, parainfluenza types 1-4, respiratory syncytial virus A/B, rhinovirus/enterovirus, coronavirus subtypes, human bocavirus, C. pneumoniae, M. pneumoniae, Legionella pneumophila Adenovirus, human metapneumovirus, influenza A subtypes, influenza B, parainfluenza Bordetella parapertussis/ types 1-4, respiratory syncytial virus A/B, rhinovirus, bronchiseptica, Bordetella holmesii, B. pertussis Acinetobacter calcoaceticus-baumannii complex, K. oxytoca, S. marcescens, E. cloacae complex, K. pneumoniae group, S. aureus, E. coli, Moraxella catarrhalis, S. agalactiae H. influenzae, Proteus spp, S. pneumoniae, K. aerogenes, P. aeruginosa, S. pyogenes, C. pneumoniae, L. pneumophila, M. pneumoniae,
Pathogens: adenovirus, human rhinovirus/wnterovirus, parainfluenza virus, coronavirus, influenza A, respiratory syncytial virus, human metapneumovirus, influenza B Resistance markers: CTX-M, NDM, mecA/C and MREJ, IMP, OXA-48like, KPC, VIM Pathogens: Acinetobacter spp, C. pneumoniae, Citrobacter freundii, E. coli, E. cloacae complex, Haemophilus influenza, K. oxytoca, K. pneumoniae, Klebsiella variicola, Legionella pneumophila, Moraxella catarrhalis, Morganella morganii, M. pneumoniae, Proteus spp, P. aeruginosa, S. marcescens, S. aureus, S. maltophilia, S. pneumoniae Resistance markers: CTX-M, KPC, mecA, NDM, OXA-23, OXA-24, OXA-48, OXA-58, TEM, VIM
Respiratory
mPCR NPS 45 min
FilmArray Respiratory Panel 2.1
(BioFire) NPS 6 h
mPCR, electrowetting, eSensor technology
eSensor XT-8 Respiratory Viral Panel
(GenMark Diagnostics) NPS <2 h
mPCR, electrowetting, eSensor technology mPCR NPS <3 h
ePlex Respiratory Pathogen Panel 2
(GenMark Diagnostics)
NxTAG Respiratory Pathogen Panel
(Luminex) mPCR NPS <2 h
Verigene Respiratory Pathogens Flex Test
(Luminex) 1 h
Tracheal aspirate, BAL
mPCR
FilmArray Pneumonia Panel
(BioFire) <5 h
Tracheal aspirate, BAL
mPCR
Unyvero Lower Respiratory Tract Panel
(Curetis) Real-time PCR Nasal 1 h MRSA
Xpert MRSA NxG test,
GeneXpert (Cepheid) 6-13 min SARS-CoV-2, influenza A/B, respiratory syncytial virus A/B, GAS 18-36 min SARS-CoV-2, influenza A/B, respiratory syncytial virus, GAS
NPS or throat NPS or throat
Isothermal nucleic acid amplification Real-time PCR
(Abbott)
ID NOW Xpert Xpress CoV-2/ Flu/RSV plus
(Cepheid) Adenovirus, coronavirus, human metapneumovirus, influenza A, influenza A subtype H1, influenza A subtype H3, influenza A subtype H1-2009, influenza B, parainfluenza virus, B. pertussis, C. pneumoniae, human rhinovirus/enterovirus, respiratory syncytial virus, M. pneumoniae
mPCR NPS 1 h
FilmArray Respiratory Panel EZ
(BioFire)
BAL, bronchoalveolar lavage; GAS, group A Streptococcus ; MRSA, methicillin-resistant S. aureus ; NPS, nasopharyngeal swab
stewardship potential of the T2Candida Panel, if any, appears minimal and heavily contingent on the effectiveness of the ASP intervention as clinicians are particularly apprehensive about de-escalation in patients already at substantially high risk for fungal infections.
The T2Bacteria Panel by T2 Biosystems has a similar turnaround time of 3 to 5 hours for the detection of BSIs caused by 5 bacteria: Enterococcus faecium, Staphylococcus aureus, Klebsiella pneumoniae, Pseudomonas aeruginosa, and Escherichia coli. Its sensitivity, specificity, negative predictive value (NPV), positive percent agreement, and negative percent agreement, paired with blood cultures, are all more than 90%.25,33,34 Compared with blood cultures (mean, 71.7 [SD, 39.3] hours), there was a shorter time from initiation of testing to detection and identification of pathogens (7.70 [SD, 1.38] hours), but a 10% false-positive rate was observed for its targeted organisms.25 Additionally, the T2Bacteria assay appeared to detect 25% more positives than blood culture in a small, prospective noninterventional study in the emergency department, which were deemed to be associated with evidence of infection, but true positivity remains unclear.33 Interestingly, 20 patients were identified in a study of 233 participants with 21 (9%) discordant results, positive T2Bacteria cases and negative blood cultures. Eleven (52.5%) cases had probable BSI, 4 (19%) had possible BSI, and 6 (28.5%) were presumed false positives.35 Closed-space and localized infections (mostly pyelonephritis and abscess) and recent use of antimicrobial agents were characteristics among the probable and possible BSIs with discrepancies.35
In a recent evaluation of both platforms, Giannella et al conducted a systematic review of 14 controlled studies, the majority being observational, comparing T2MR with blood culture for the detection of bacterial and fungal BSIs. Patients testing positive were found to have received targeted antimicrobial therapy faster (by 42 hours; P<0.001). Patients who tested negative were de-escalated from empiric therapy faster (by 7 hours; P=0.02).36 Length of stay in the ICU (mean difference, –5.0 days; P=0.03) and hospital LOS (–4.8 days; P=0.03) were shorter with T2MR, but no difference was observed for mortality (28.9% vs 29.9%; RR [rate ratio], 1.02; P=0.86).36 Further prospective, ideally interventional, studies are needed to justify the role of T2MR along with ASPs in patient care.37
The Karius Test is a novel metagenomic microbiological diagnostic test that uses next-generation sequencing (NGS) to detect degraded microbial DNA released into the plasma, also known as cell-free DNA, from 1,250 bacteria, fungi, parasites, and viruses to facilitate the improvement of infectious disease diagnosis by elevating diagnostic yield, shortening time to diagnosis, and avoiding invasive testing for specimen collection.38-40 Given its noninvasive nature and that only 5 mL of whole blood is required, NGS has been coined “liquid biopsy” by the company. Once the sample is received in the laboratory, quantitative results in molecules per microliter with a reference range based on pooled results from 167 asymptomatic donors are available by the next day.40 Plasma proceeds to processing through DNA extraction; sequencing, where billions of DNA fragments are simultaneously and independently sequenced with the goal to interrogate all genetic sequences present in the specimen; and analyze against a database of pathogen genomes.40
While clinical data are still limited, this new technology may have a particular niche in the diagnosis and identification of infectious etiologies for pneumonia, bacteremia, endovascular infections, and febrile neutropenia (FN) despite pretreatment with antibiotics.38,39,41 For complicated community-acquired pneumonia (CAP), NGS results were retrospectively compared with standard culture methods and found to be pathogen positive in 45 (98%) of 46 hospitalized children with the causative pathogen identified in 41 (89%). In 32 (70%) children, NGS was the only method for pathogen identification. Management changed in 36 (78%) children based on NGS results, with antibiotic spectrum narrowing in 29 (81%).42 In a prospective study of 55 patients with FN, NGS sensitivity and specificity for definite, probable, and possible cases of infection were 85% (41/48) and 100% (14/14%), respectively. Real-time NGS results could have optimized antimicrobials earlier in 47% of patients, by addition of antibacterials (20%; mostly against anaerobes, 12.7%), antivirals (14.5%), and/or antifungals (3.6%), and antimicrobial de-escalation in 27.3% of cases.43
Furthermore, NGS was assessed retrospectively in 31 patients with either hematologic malignancy or hematopoietic stem cell transplant (HSCT) who had persistent fever and/or imaging suspicious for infection. NGS results escalated antibiotics in 28% of cases (9/32) and de-escalated antibiotics in 31% of cases (10/32) with overall management changed in 59% (19/32) of patients. Sensitivity and specificity were 80% and 58% for clinically significant infection, respectively. Some notable uncommon and difficult-to-diagnose organisms detected were Nocardia, Legionella, Toxoplasma, and Pneumocystis jirovecii. 44 In 3 studies of mostly HSCT recipients
and leukemia or lymphoma patients, the sensitivity of NGS for biopsy-proven/probable Aspergillus and non-Aspergillus invasive mold infections (IMI) was found to be 51%.45-47 The specificity and PPV were estimated to be 100% based on no findings of false positives in 19 controls, while NPV was estimated to be 81% to 99%.45 When combined with serum galactomannan, NGS had an improved sensitivity of 84%.45 Future larger studies are needed to validate the utility of this test in these patient populations.
Respiratory Infections Respiratory Viral Panels
Pneumonia is a major contributor to morbidity and mortality in the United States, accounting for 1.2 million hospitalizations and 63,000 deaths annually.48,49 Although recommendations from the American Thoracic Society/Infectious Diseases Society of America (IDSA) for empiric antibiotic therapy in CAP are based on targeting the major treatable respiratory bacterial organisms, hospitalized patients are more than twice as likely to harbor respiratory viruses as bacteria, and influenza only marginally accounts for the respiratory viruses with the propensity to cause pneumonia.50-53 Antibiotics are commonly overused, because distinguishing between bacterial and viral etiologies in lower respiratory tract infections (LRTIs) is difficult due to similar manifestations.54 Antibiotic therapy may be withheld safely in patients diagnosed with only viral pneumonia as long as these infections may be discriminated from those with concomitant bacterial etiologies.55
As an important intervention to reduce the inappropriate use of antibiotics, antimicrobial stewardship guidelines advocate rapid testing for broad panels of respiratory viruses.56 The BioFire FilmArray Respiratory Panel 2.1 is a PCR-based multiplex nucleic acid test intended for use with the BioFire System, which simultaneously identifies nucleic acids from 18 different viruses and 4 bacteria associated with acute respiratory tract infections from a single nasopharyngeal specimen.57 The eSensor XT-8 Respiratory Viral Panel (GenMark Diagnostics) is based on the principles of competitive DNA hybridization and electrochemical detection. This panel includes assays for 8 viruses, including adenovirus groups, coronavirus types, influenza A virus, influenza B virus, human metapneumovirus, parainfluenza virus types, respiratory syncytial virus types, and rhinovirus. Nucleic acids first are extracted from the specimen for real-time PCR assays. The target DNA is mixed with the signal probe solution specific for the different viral targets, and hybridization to signal probes occurs immediately if the applicable target DNA is present. The solution is pumped through the cartridge’s microfluidic chamber and the target DNA/signal probe complex reacts with the preassembled capture probe specific for individual viral targets. An electrical current is swept across each electrode and target DNA is analyzed by electrochemical detection.58,59 The ePlex Respiratory Pathogen Panel 2 (GenMark Diagnostics) is a qualitative nucleic acid multiplex test that detects 19 of the most common viruses and 2 bacterial respiratory pathogens in nasopharyngeal specimens. As described for the eSensor XT-8 Respiratory Viral Panel, this test uses GenMark’s eSensor technology, based on the principles of competitive DNA hybridization and electrochemical detection, combined with electrowetting microfluidics.60 The NxTAG Respiratory Pathogen Panel (Luminex) is also a qualitative test that simultaneously detects and identifies nucleic acids from 18 respiratory viruses and 3 bacteria in nasopharyngeal swabs collected from individuals with clinical signs and symptoms of an acute respiratory tract infection. Nucleic acid extraction is followed by loading extracted nucleic acid to pre-plated test wells, and then multiplex reverse transcriptasePCR and hybridization occurs with data acquisition on the MAGPIX instrument.61,62 Lastly, the Verigene Respiratory Pathogens Flex Test (Luminex) uses reverse transcription, PCR, and microarray hybridization to detect gene sequences of 13 viruses (adenovirus, human metapneumovirus, influenza A subtypes, influenza B, parainfluenza 1-4, respiratory syncytial virus A and B, and rhinovirus), and 3 bacteria (Bordetella parapertussis/bronchiseptica, Bordetella holmesii, and Bordetella pertussis).63
According to a multicenter, retrospective cohort analysis of adult patients admitted with suspected pneumonia in 179 hospitals nationwide, only a minority of patients were tested for a fraction of the potential respiratory viruses, with most tested for influenza. Patients with positive viral test results often received a prolonged duration of unnecessary antibacterial courses even with concurrent negative bacterial tests.64 The IDSA’s Diagnostics Committee suggests that the combination of respiratory viral testing and procalcitonin (PCT) may be more likely to exclude bacterial coinfection with confidence in a meaningful time frame.65 The respiratory viral panel (RVP) combined with PCT and either direct or indirect (automated best practice alert) ASP intervention had a higher proportion of antibiotic discontinuation or deescalation and significantly reduced antibiotic days of therapy, but this effect was observed only with ASP intervention.66-68 Reduction in antibiotic days of therapy observed with RVP and PCT combination with a varying level of ASP intervention appears to be similar, if not greater, and more consistent compared with solely PCT or RVP use with ASP intervention, but more robust head-to-head comparisons are needed to confirm these speculations.54,66,67,69,70
Lower Respiratory Tract Infection Panels
The BioFire FilmArray Pneumonia Panel and Curetis
Unyvero Lower Respiratory Tract (LRT) Panel are multiplex nucleic acid panels for the simultaneous detection and identification of nucleic acid sequences from microorganisms and resistance markers in sputumlike or bronchoalveolar lavage (BAL)-like specimens obtained from patients suspected to have LRTIs.71,72 The BioFire FilmArray Pneumonia Panel is intended for use with the BioFire System, identifying 8 viruses, 8 resistance genes, and 3 atypical bacteria using qualitative targets. Fifteen typical bacterial targets are reported semiquantitatively with bins of 104, 105 , 106, or 107 or more genomic copies/mL of bacterial nucleic acid in the specimen to estimate the relative abundance of nucleic acid from common bacteria in a sample.71 Although semiquantitative analysis may improve clinical specificity, airway colonizers cannot be distinguished from invasive pathogens. The Curetis Unyvero LRT Panel is purely qualitative, and able to detect 29 bacterial pathogens and 19 resistance genes. This panel automates and integrates DNA purification, 8 parallel multiplex end point PCR reactions, and detection of nucleic acid using hybridization on PCR chamber arrays in a single-use cartridge from LRTI pathogens in a single respiratory specimen.72
Molecular testing for bacterial pathogens was not addressed by the current pneumonia guidelines, because their performance and potential impact on clinical decision making are still undetermined.53,73 The clinical applicability of these panels is likely to be in patients who have new or worsening lung infiltrates, are moderately to severely ill, and/or have received empiric antibiotics prior to obtaining cultures.65 Rand et al found a sensitivity of 97.8% (95% CI, 94.3%-99.4%), specificity of 80.4% (95% CI, 74.5%85.4%), PPV of 80% (95% CI, 75.5%-84%), and NPV of 97.8% (95% CI, 94.3%-99.1%) for the BioFire FilmArray Pneumonia Panel for bacterial pathogens compared with endotracheal and BAL cultures.74 Kolenda et al observed similar sensitivity and specificity for the panel, but 60.5% of bacterial targets detected by the panel were not recovered by culture. Also, 76.9% of discordant results corresponded to commensal oral flora bacteria and/or 105 copies/mL or less of bacterial nucleic acids.75 The high sensitivity may be useful to deter inappropriate prescribing of antibiotics by ruling out bacterial coinfections, but positive results should be interpreted with caution. Resistance genes can be detected in concordance with results by culture but cannot be linked definitively to the microorganism(s) detected. Culture results are still required in conjunction with panel results to confirm susceptibility or resistance.76 The BioFire FilmArray Pneumonia Panel may have the potential to reduce unnecessary antimicrobial exposure and increase the appropriateness of empiric antibiotic therapy based on observed antimicrobial de-escalation in 63 of 159 (40%) and escalation in 35 (22%) hospitalized pneumonia patients.77 The Curetis Unyvero LRT Panel has reported similar diagnostic accuracy, but there are comparable interpretation challenges and not all genes could be attributed to an organism, highlighting the necessary continuation of current culture methods with antimicrobial susceptibility testing.78,79 Results from Pickens et al predicted antibiotic de-escalation from unnecessary methicillin-resistant Staphylococcus aureus (MRSA) and P. aeruginosa coverage in 65.9% (405/615) of patients.80 Interpretation challenges with pneumonia panels, especially with resistance genes, may be assisted by ASPs, but this requires further exploration.
Clinical Utility of Screening for MRSA With Nasal PCR
The Xpert MRSA NxG test, from GeneXpert Cepheid, has become a robust ASP tool for de-escalation from MRSA therapy in predominantly patients with suspected or confirmed pneumonia.81 This serves as a qualitative ancillary test to rule out MRSA pneumonia by detecting MRSA DNA directly in nasal swabs from patients at risk for nasal colonization. The technology involves real-time PCR for the amplification of MRSA-specific DNA targets and fluorogenic targetspecific hybridization probes for the real-time detection of the amplified DNA.82 Current guidelines for CAP recommend the routine use of the MRSA nasal PCR for the de-escalation of MRSA coverage.53 With an NPV of more than 95% combined with ASP implementation, the MRSA nasal PCR was found to significantly reduce unnecessary vancomycin use and associated costs.83-85
Outpatient Antimicrobial Prescribing And Diagnostic Potential
Respiratory Infections
The RDTs have been used mainly in the inpatient setting, but millions of patients are affected by respiratory tract infections (RTIs) in the outpatient setting annually, resulting in millions of outpatient antibiotic prescriptions.86-88 Based on population database evaluations in the United States, up to half of prescriptions are considered inappropriate based on indication, drug, dose, or duration prescribed.87,88 If RDTs could be performed closer to healthcare providers, this could cause a paradigm shift from presumptive to definitive treatments of infectious disease syndromes in outpatient clinics.4 Primary care logistics require RDT technologies to accommodate the time constraints of brief office visits for RTIs, which on average are only 15 minutes.89
Common RDT platforms such as agglutination or lateral flow tests are very simple, require minimal familiarity and almost no equipment, and provide rapid results in a few minutes. In a lateral flow test, the specimen is placed onto a strip to migrate across the zone where the antigen or antibody is captured, and visual
identification occurs after a certain period. The sensitivity and qualitative nature of these results may limit their interpretation.90 Agglutination assays work by observing carrier particles binding to target analytes in visible clumps. Interpretation may be limited by low sensitivity and inconclusive results in weak reactions.90
Molecular point-of-care testing, using ID NOW and BinaxNOW (Abbott), Cobas Liat PCR (Roche Diagnostics), Xpert Xpress (Cepheid), BD Veritor Plus (BD), and FilmArray Respiratory Panel EZ (BioFire), is increasingly available in the outpatient setting, but their use is limited by regulations for testing (Clinical Laboratory Improvement Amendments [CLIA] Act waivers). If CLIA-waived, these tests may have limited implementation due to cost, lack of laboratory oversight, and even achieving practical turnaround time (<20 minutes) during primary care visits.91 ID NOW is an automated multiplex assay that uses isothermal nucleic acid amplification technology for the qualitative detection of influenza A/B, respiratory syncytial virus (RSV) A/B, SARS-CoV-2, and Group A Strep nucleic acids from nasal, nasopharyngeal, and/or throat swabs. Templates, similar to primers, designed to target viral RNA or bacterial DNA are used for amplification. Fluorescent-labeled molecular beacons are used to identify each of the amplified RNA or DNA targets. Results are available within 6 to 13 minutes.92-95 The Xpert Xpress CoV-2/Flu/RSV Plus qualitatively detects RNA from SARS-CoV-2, influenza A/B, and/or RSV in nasopharyngeal swabs in approximately 36 minutes.96,97 Also, the Xpert Xpress Strep A test is available in the same platform for Group A Strep DNA detection in throat specimens in 18 minutes.98 The Cepheid GeneXpert Xpress instrument automates and integrates preparation, nucleic acid extraction, amplification, and detection of the target sequences by using real-time PCR. The BioFire FilmArray Respiratory Panel EZ, a simplified version of the BioFire FilmArray Respiratory Panel, was demonstrated to significantly improve the appropriate use of antibiotics and was associated with a decrease in clinic appointment duration in an outpatient pediatric clinic.99 The turnaround time of 1 hour likely will limit the clinical utility of this test compared with the other tests. Additionally, this simplified test is uncertain to reduce the use of downstream healthcare resources, including radiological and laboratory tests, telephone calls, and follow-up appointments.100
A major limitation of molecular diagnostic tests is appropriate utilization and interpretation.101,102 When an organism is detected, pathogenicity may not necessarily be reflected; colonization or prolonged shedding also should be considered.88,103 Clearly, there is an opportunity for collaboration between the laboratory and clinicians to incorporate these tests into ASP and diagnostic stewardship efforts to mitigate the risk for overtesting and misinterpretation.101,102
Conclusion
As the field of infectious disease management continues to evolve, RDTs have been increasingly recognized for their significant impact on clinical outcomes, antimicrobial use, and cost savings. For the management of BSIs, invasive candidiasis, and inpatient and outpatient respiratory infections, there is a multitude of technological advances. A comprehensive chart of the RDTs discussed with respect to detection method, sample type required, turnaround time, pathogen identification, and resistance gene detection is shown in the Table. Evaluations of the efficacy of these technologies are imperative and should ensure their value is not only increasing the accuracy and speed of diagnosis, but also changing clinical management, improving patient outcomes, and yielding overall cost-effectiveness.
References
1. Graf EH, et al. Curr Infect Dis Rep. 2020;22(2):5. 2. Li J, et al. Medicine (Baltimore). 2016;95(19):e3587. 3. Mashalla Y, et al. Int J Clin Pract. 2017;71(12) doi: 10.1111/ijcp.13042. 4. Vasala A, et al. Front Cell Infect Microbiol. 2020;10:308. 5. VITEK 2 Identification Cards. https://www.biomerieuxdiagnostics.com/vitekr-2-identification-cards 6. VITEK 2. https://www.biomerieux-diagnostics.com/vitekr-2-0 7. Liu ZK, et al. Med Princ Pract. 2005;14(4):250-254. 8. Yoon EJ, et al. Antibiotics (Basel). 2021;10(8):982. 9. Singhal N, et al. Front Microbiol. 2015;6:791. 10. BioFire Blood Culture Identification (BCID2) Panel Instruction Booklet. https://www.biofiredx.com/support/ documents/#toggle-id-3 11. ePlex Blood Culture Identification Panels. https://www. genmarkdx.com/wp-content/uploads/2021/01/BCID-
Compendium_GNMK-IMC-7165-A.pdf 12. Huang TD, et al. J Clin Microbiol. 2019;57(2). 13. VERIGENE NanoGrid Technology. https://www.luminexcorp. com/verigene-nanogrid-technology/ 14. Timbrook TT, et al. Clin Infect Dis. 2017;64(1):15-23. 15. Pliakos EE, et al. Clin Microbiol Rev. 2018;31(3):e00095-17. 16. Charnot-Katsikas A, et al. J Clin Microbiol. 2018;56(1)e01166. 17. Chasteen A. In: Staff CE, ed. ASM Microbe Conference 2016. https://www.youtube.com/watch?v=sBtOUlzuJ78. 18. Banerjee R, et al. Clin Infect Dis. 2021;73(1):e39-e46. 19. Dare RK, et al. Clin Infect Dis. 2021;73(11):e4616-e4626. 20. Ehren K, et al. Clin Infect Dis. 2020;70(7):1285-1293. 21. Sheth S, et al. Antimicrob Agents Chemother. 2020;64(9):e00578. 22. MacVane SH, et al. J Antimicrob Chemother. 2021;76(9):2453-2463. 23. Robinson ED, et al. Clin Infect Dis. 2021;73(5):783-792. 24. Bhalodi AA, et al. Clin Infect Dis. 2021;ciab921. 25. Nguyen MH, et al. Ann Intern Med. 2019;170(12):845-852. 26. Pappas PG, et al. Clin Infect Dis. 2016;62(4):e1-e50. 27. Clancy CJ, et al. Curr Opin Infect Dis. 2019;32(6):546-552. 28. Gill CM, et al. Diagn Microbiol Infect Dis. 2019;95(2):162-165.
29. Mylonakis E, et al. Clin Infect Dis. 2015;60(6):892-899. 30. Patch ME, et al. J Antimicrob Chemother. 2018;73(suppl_4):iv27-iv30. 31. Steuber TD, et al. Diagn Microbiol Infect Dis. 2020;97(4):115086. 32. Bomkamp JP, et al. J Clin Microbiol. 2020;58(3):e01408-e01419. 33. Voigt C, et al. J Emerg Med. 2020;58(5):785-796. 34. Drevinek P, et al. Microbiologyopen. 2021;10(3):e1210. 35. Kalligeros M, et al. BMC Infect Dis. 2020;20(1):326. 36. Giannella M, et al. Expert Rev Med Devices. 2021;18(5):473-482. 37. Weinrib DA, et al. Ann Intern Med. 2019;170(12):888-889. 38. Goggin KP, et al. JAMA Oncol. 2020;6(4):552-556. 39. Hogan CA, et al. Clin Infect Dis. 2021;72(2):239-245. 40. Morales M. Clinical Microbiology Newsletter. 2021;43(9):69-79. 41. To RK, et al. Pediatr Infect Dis J. 2021;40(5):486-488. 42. Dworsky ZD, et al. Hosp Pediatr. 2022;12(4):377-384. 43. Benamu E, et al. Clin Infect Dis. 2021 Apr 19;ciab324. 44. Yu J, et al. Transplant Cell Ther. 2021;27(6):500 e501-500 e506. 45. Hill JA, et al. Clin Infect Dis. 2021;73(11):e3876-e3883. 46. Hong DK, et al. Diagn Microbiol Infect Dis. 2018;92(3):210-213. 47. Armstrong AE, et al. Pediatr Blood Cancer. 2019;66(7):e27734. 48. Kung HC, et al. Natl Vital Stat Rep. 2008;56(10):1-120. 49. Agency for Healthcare Research and Quality. http://www.ahrq. gov/research/sep08/0908RA40.htm 50. Burk M, et al. Eur Respir Rev. 2016;25(140):178-188. 51. Piralla A, et al. J Clin Virol. 2017;92:48-51.
52. Jain S, et al. N Engl J Med. 2015;373(24):415-427. 53. Metlay JP, et al. Am J Respir Crit Care Med. 2019;200(7):e45-e67. 54. Huang DT, et al. N Engl J Med. 2018;379(20):1973. 55. Ruuskanen O, et al. Lancet. 2011;377(9773):1264-1275. 56. Barlam TF, et al. Clin Infect Dis. 2016;62(10):e51-77. 57. BioFire Respiratory Panel 2.1 (RP2.1). https://www.fda.gov/ media/142696/download 58. Technology. https://www.genmarkdx.com/education/ technology/ 59. Pierce VM, et al. J Clin Microbiol. 2012;50(11):3458-3465. 60. Babady NE, et al. J Clin Microbiol. 2018;56(2):e01658. 61. NxTAG Respiratory Pathogen Panel. https://www.luminexcorp. com/nxtag-respiratory-pathogen-panel-2/ 62. NxTAG Respiratory Pathogen Panel + SARS-CoV-2 [package insert]. https://www.fda.gov/media/146495/download 63. VERIGENE Respiratory Pathogens Flex Test. https://www. luminexcorp.com/respiratory-pathogens-flex-test/#overview 64. Klompas M, et al. Infect Control Hosp Epidemiol. 2021;42(7):817-825 65. Hanson KE, et al. Clin Infect Dis. 2020;71(10):2744-2751. 66. Moradi T, et al. Clin Infect Dis. 202;71(7):1684-1689. 67. Lee CC, et al. J Am Med Dir Assoc. 2020;21(1):62-67. 68. Timbrook T, et al. Infect Dis Ther. 2015;4(3):297-306. 69. Schuetz P, et al. Cochrane Database Syst Rev. 2012(9):CD007498. 70. Srinivas P, et al. Pharmacotherapy. 2019;39(6):709-717. 71. BioFire FilmArray Pneumonia Panel. https://www.biofiredx. com/products/the-filmarray-panels/filmarray-pneumonia/ 72. Unyvero Application Manual - Lower Respiratory Tract (LRT). 2021.
73. Kalil AC, et al. Clin Infect Dis. 2016;63(5):e61-e111. 74. Rand KH, et al. Open Forum Infect Dis. 2021;8(1):ofaa560. 75. Kolenda C, et al. Open Forum Infect Dis. 2020;7(11):ofaa484. 76. Yoo IY, et al. Int J Infect Dis. 2020;95:326-331. 77. Monard C, et al. Crit Care. 2020;24(1):434. 78. Klein M, et al. Multicenter Evaluation of the Unyvero Platform for Testing Bronchoalveolar Lavage Fluid. J Clin Microbiol. 2021;59(3):e02497. 79. Collins ME, et al. J Clin Microbiol. 2020;58(5):e02013-e02019. 80. Pickens C, et al. Diagn Microbiol Infect Dis. 2020;98(4):115179. 81. Parente DM, et al. Clin Infect Dis. 2018;67(1):1-7. 82. Xpert MRSA NxG 2019. https://www.cepheid.com/Package%20 Insert%20Files/Xpert-MRSA-NxG-US-IVD-ENGLISH-PackageInsert-301-4055-Rev-E.pdf 83. Willis C, et al. Am J Health Syst Pharm. 2017;74(21):1765-1773. 84. Meng L, et al. Open Forum Infect Dis. 2021;8(4):ofab099. 85. Smith MN, et al. J Crit Care. 2017;38:168-171. 86. Drekonja DM, et al. Infect Control Hosp Epidemiol. 2015;36(2):142-152. 87. Fleming-Dutra KE, et al. JAMA. 2016;315(17):1864-1873. 88. King LM, et al. BMJ. 2018;363:k3047. 89. Linder JA, et al. Clin Ther. 2003;25(9):2419-2430. 90. Rapid Diagnostic Test (RDT). http://www.globalhealthprimer. emory.edu/targets-technologies/rapid-diagnostic-test.html 91. Cawcutt KA, et al. Clin Infect Dis. 2021;72(12):e1115-e1121. 92. ID NOW Influenza A & B 2 [product insert]. https://www. globalpointofcare.abbott/en/product-details/id-nowinfluenza-ab-2.html 93. ID NOW RSV [package insert]. https://www.globalpointofcare. abbott/en/product-details/id-now-rsv.html 94. ID NOW COVID-19 [product insert]. https://www.fda.gov/ media/136525/download 95. ID NOW Strep A 2 [package insert]. https://www.globalpointofcare.abbott/en/product-details/id-now-strep-a-2.html 96. Xpert Xpress CoV-2/Flu/RSV plus. https://www. cepheid.com/en_US/tests/Critical-Infectious-Diseases/ Xpert-Xpress-CoV-2-Flu-RSV-plus 97. Xpert Xpress CoV-2/Flu/RSV plus. 2021. https://www. cepheid.com/en_US/tests/Critical-Infectious-Diseases/ Xpert-Xpress-CoV-2-Flu-RSV-plus 98. Xpert Xpress Strep A. https://bit.ly/3NjiKqs-IDSE 99. Beal SG, et al. Pediatr Infect Dis J. 2020;39(3):188-191. 100. Fenton J, et al. Pediatr Infect Dis J. 2020;39(9):e282-e283. 101. Patel R, et al. Clin Infect Dis. 2018;67(5):799-801. 102. Messacar K, et al. J Clin Microbiol. 2017;55(3):715-723. 103. Caliendo AM, et al. Clin Infect Dis. 2013;57 suppl 3:S139-S170.
Dr. Fong reported no relevant financial disclosures.
About the author
Karen Fong, PharmD, BCIDP, is a clinical pharmacist, Infectious Diseases and Antimicrobial Stewardship, in the Department of Pharmacy, at the University of Utah Health, in Salt Lake City, Utah.