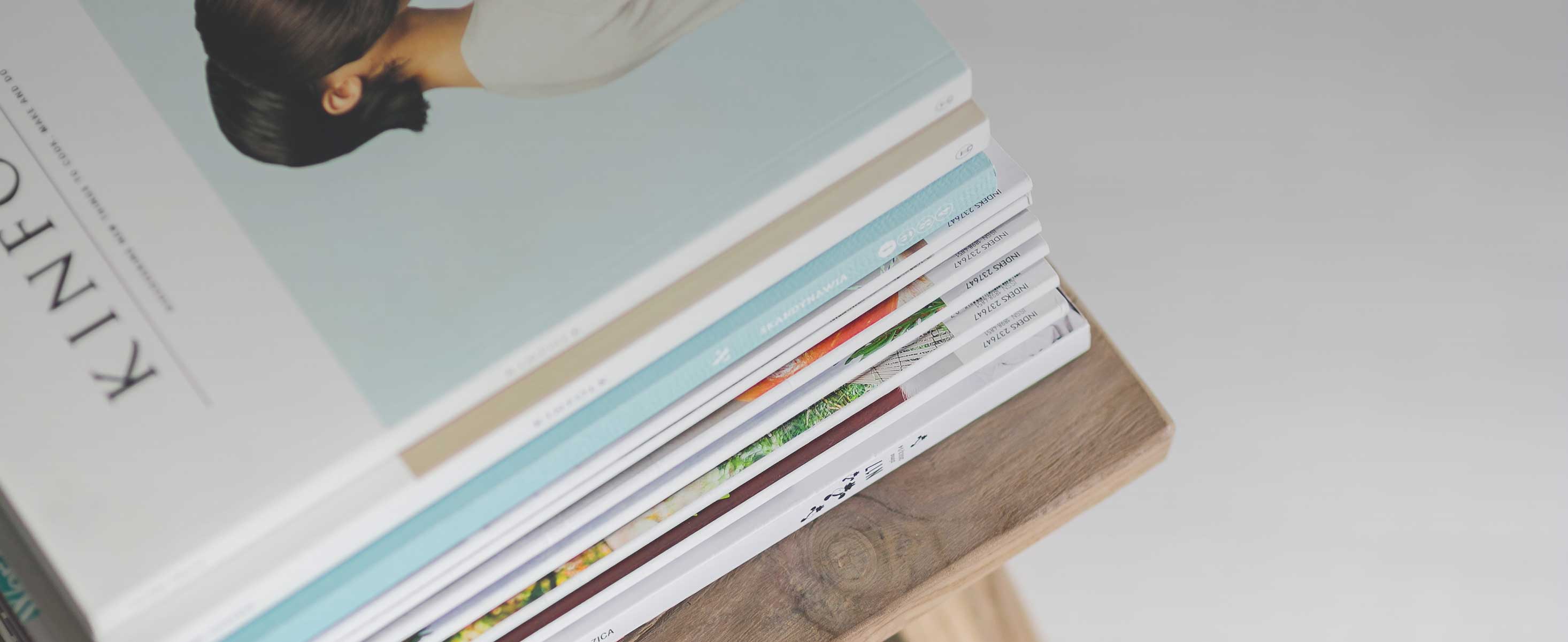
5 minute read
A Look into Conventional and mRNA Vaccines in Application to COVID-19
from Mindscope Issue 10
by MindScope
By Annie Chen, Biochemistry Major, 2021
Vaccines help the body to safely build immunity against disease by imitating an infection. The use of vaccines has drastically prevented infections including, but not limited to, measles, chickenpox, hepatitis B, tetanus, polio, and yearly influenza.
Advertisement
The conventional methods to develop vaccines include using (1) live, attenuated pathogens that use a weakened version of the living virus or bacteria to mimic the actual infection within individuals with healthy immune systems.4 Several examples of live, attenuated vaccines include those for measles, mumps, rubella, and chickenpox. (2) Inactivated pathogens also fight viruses and bacteria by deactivating the germ during the process of designing the vaccine; however, this method requires multiple doses to maintain immunity.4 An example of inactivated vaccines is used to prevent polio. (3) Subunit vaccines fight against viruses and bacteria by including essential antigens, rather than the entire parts of virus or bacteria, to mimic infection with fewer vaccine side effects.4 An example of a subunit vaccine includes the DTaP vaccine to prevent pertussis (whooping cough). (4) Finally, conjugated vaccines fight against bacteria by connecting or conjugating to a bacteria’s polysaccharide coating to allow the immune system to react.4 A conjugated vaccine was developed to prevent Haemophilus influenzae type B (Hib).
Although conventional vaccines have prevented certain types of infections, there are still obstacles to create vaccines that can prevent infectious pathogens that evade the immune system, can be applicable to non-infectious diseases such as cancer, and can be produced for rapid and large-scale use.3 Certainly, with the COVID-19 pandemic, there has been a rapid search and development of an effective vaccine against the spike protein, which is found on the surface of the virus and allows it to enter human cells.
Recent research has made strides in nucleic acid therapeutic interventions, including DNA-based and protein-based approaches, as emerging alternatives to conventional vaccines.3 Notably, the advent of messenger RNA (mRNA) for vaccine development provides promising safety, efficacy, and production benefits compared to conventional vaccines.
Messenger RNA is a single-stranded molecule that is transcribed (copied) from a strand of DNA and translated into proteins in the cytoplasm. In the first step, a template strand on the parental double-stranded DNA containing the genetic code is transcribed into single-stranded mRNA in the nucleus of the cell. Next, the mRNA travels from the nucleus to the cytoplasm where protein-synthesizing machinery, known as ribosomes, begin to “read” the RNA genetic code in groups of three nucleotides (known as codons) and creates proteins based on the genetic information. Finally, the cell carries the protein to designated locations where the protein performs its function.
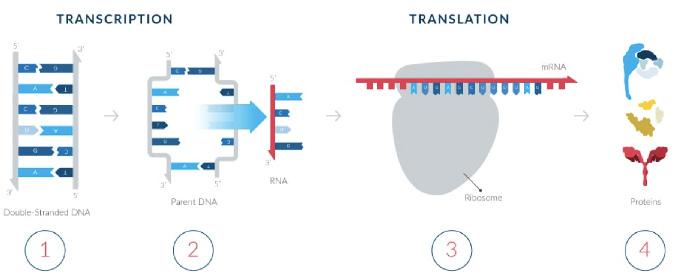
The process of creating messenger RNA (mRNAs) and Proteins
Photo courtesy of Moderna
Ultimately, it is the synthesis of proteins from mRNA instructions separates the benefits of mRNAs from conventional methods of vaccine development, like using live and attenuated or inactivated pathogens. The benefits of an mRNA vaccine include (1) no risk of infection with an mRNA vaccine as mRNA is noninfectious and non-integrating, but rather works by providing instructions to our cells to make proteins in the cytoplasm; (2) normal cellular degradation of mRNA which allows researchers to regulate the in vivo half-life of mRNAs through different delivery methods and avoid metabolic toxicity; (3) stability and high translatability of mRNAs allows efficient delivery of mRNA molecules through carrier molecules and rapid translatability in the cytoplasm; (4) minimal genetic vector of mRNA allows multiple administration of mRNA vaccines and avoids anti-vector immunity; and (5) rapid, inexpensive, and scalable manufacturing of mRNA vaccines due to high amounts of in vitro transcription reactions.3
The widespread use of mRNA vaccines is seen with the COVID-19 vaccine, which was developed rapidly with the help of worldwide collaboration and research funding. Two widely used COVID-19 vaccines developed by Moderna and Pfizer-BioNTech work with the main principle of using the virus’s genetic instruction in mRNA form to build spike proteins. The virus that causes COVID-19 is termed SARS-CoV-2, which has studded spike proteins on its surface like a crown. The vaccine containing the fragile mRNA is wrapped in a lipid nanoparticle bubble, which fuse to our cells when injected.1,2 This fusion allows mRNA to enter our cells, prompting our cells to then read mRNA to build spike proteins and later degrade the mRNA.
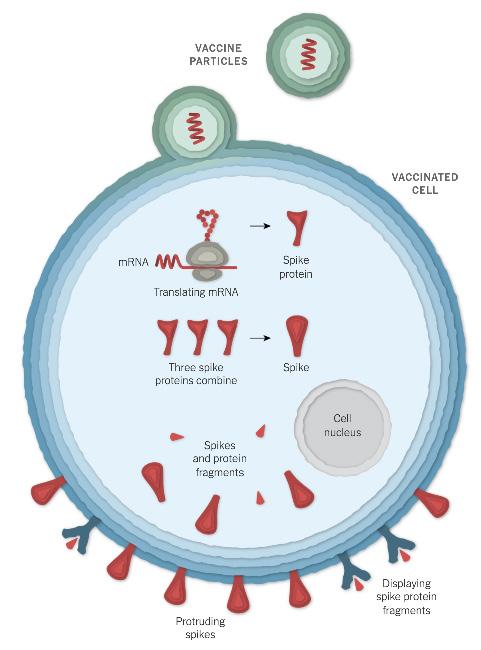
Entry of Vaccine Particles (mRNA) into a Cell and Creating Spike Protein Fragments.
Photo courtesy of New York Times.
Once spike proteins have been produced by the host cells and subsequently degraded, the fragments of spike proteins and proteins are taken up by an antigen-presenting cell.1,2 These antigen-presenting cells present the spike proteins on their surface, and this can activate immune cells including helper T cells, killer T cells and B cells. When B cells encounter the presented spike proteins and are activated by helper T cells, the B cells begin to proliferate and make antibodies against these spike proteins.1,2 This means if the SARS-CoV-2 enters the body, these antibodies bind to the virus’s spike proteins and this binding prevents the virus from entering our cells.
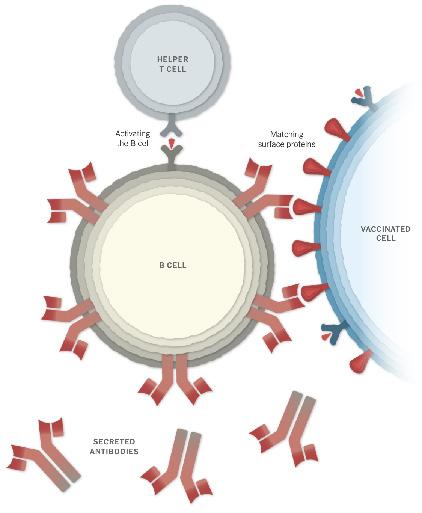
Recognition and Activation of B cells to make antibodies.
Photo courtesy of New York Times.
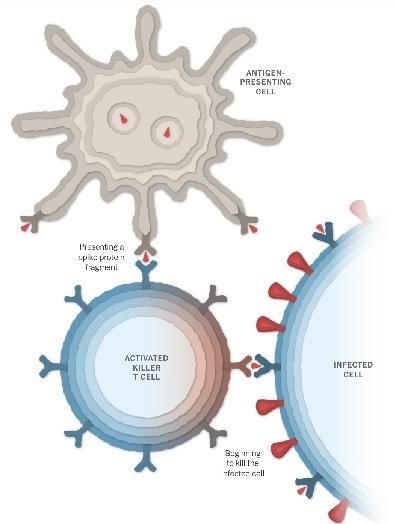
Activation of Killer T cells by AntigenPresenting Cells to Destroy infected cell.
Photo courtesy of New York Times.
Both the Moderna and Pfizer-BioNTech vaccine require two doses; however, the Moderna vaccine is given 28 days apart, whereas the Pfizer vaccine is given 21 days apart. The advances in nucleic acid therapeutics provides a promising future for mRNA vaccines.
[1] Corum, J., & Zimmer, C. (2020, December 05). How Moderna’s vaccine works. Retrieved February 26, 2021, from https://www.nytimes.com/interactive/2020/health/ moderna-covid-19-vaccine.html.
[2] Corum, J., & Zimmer, C. (2020, December 05). How the Pfizer-BioNTech vaccine works. Retrieved February 26, 2021, from https://www.nytimes.com/interactive/2020/ health/pfizer-biontech-covid-19-vaccine.html.
[3] Understanding vaccines and vaccine safety. (2015, November 30). Retrieved February 26, 2021, from https:// www.cdc.gov/vaccines/hcp/conversations/providerresources-safetysheets.html.