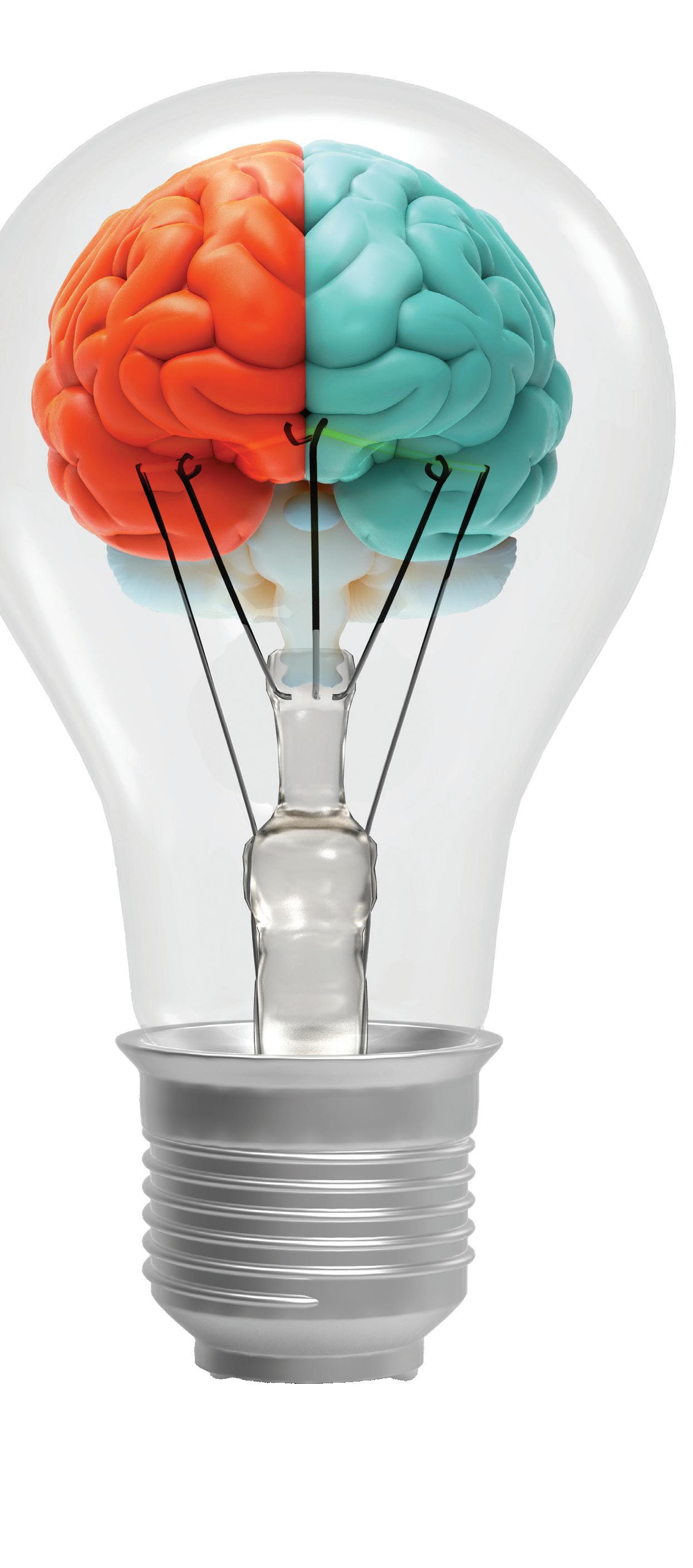
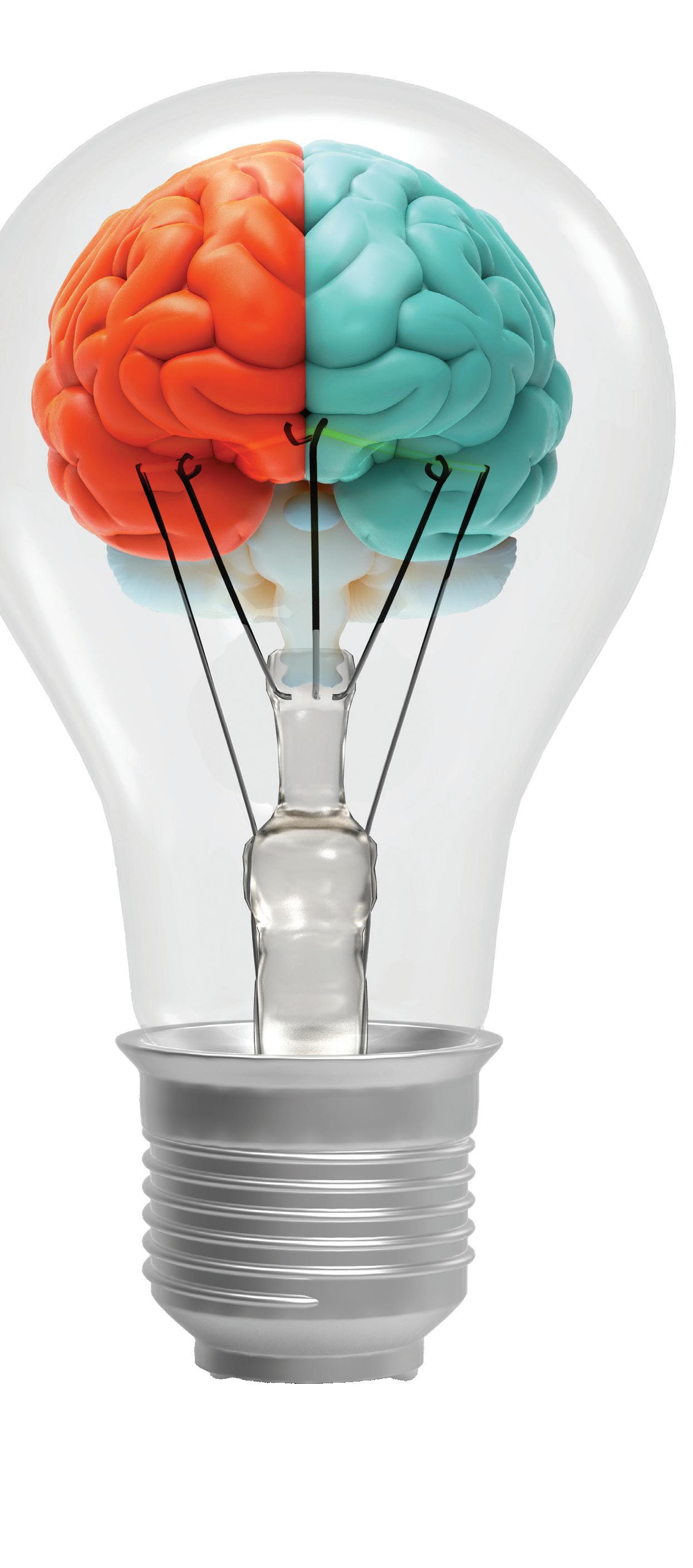
WHERE LEARNING HAPPENS
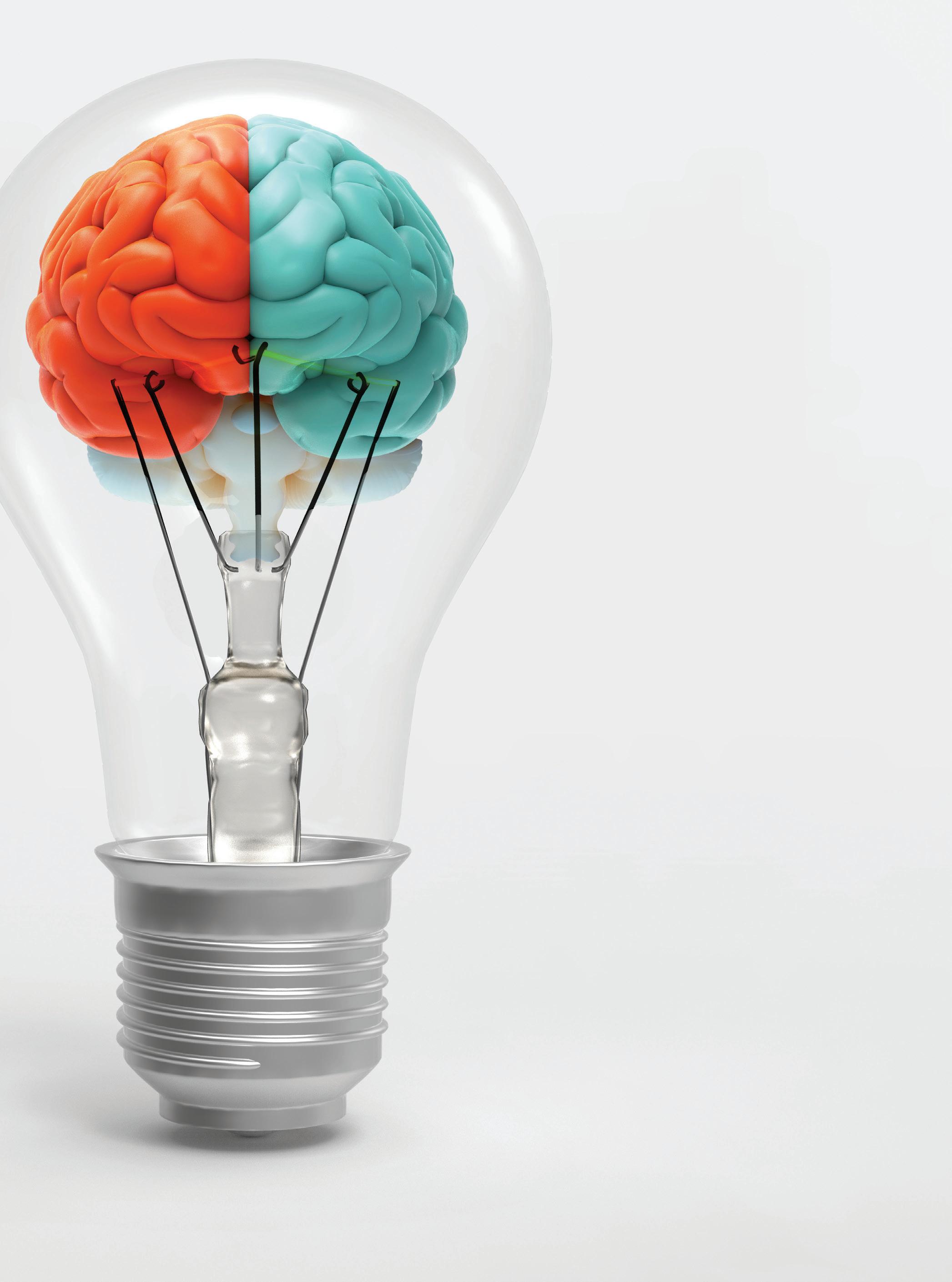
Where Learning Happens
Leveraging Working Memory & Attention in the Classroom
Copyright © 2025 by Marzano Resources
All rights reserved, including the right of reproduction of this book in whole or in part in any form.
555 North Morton Street Bloomington, IN 47404
800.733.6786 (toll free) / 812.336.7700
FAX: 812.336.7790
email: info@MarzanoResources.com MarzanoResources.com
Printed in the United States of America
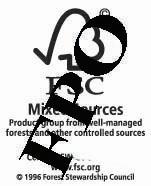
Library of Congress Cataloging-in-Publication Data
Names: Simms, Julia A., author.
Title: Where learning happens : leveraging working memory and attention in the classroom / Julia A. Simms.
Description: Bloomington, IN : Marzano Resources, [2024] | Includes bibliographical references and index.
Identifiers: LCCN 2024028778 (print) | LCCN 2024028779 (ebook) | ISBN 9781943360901 (paperback) | ISBN 9781943360918 (ebook)
Subjects: LCSH: Cognitive learning. | Human information processing in children. | Educational psychology.
Classification: LCC LB1062 .S496 2024 (print) | LCC LB1062 (ebook) | DDC 370.15/23--dc23/eng/20240924
LC record available at https://lccn.loc.gov/2024028778
LC ebook record available at https://lccn.loc.gov/2024028779
Production Team
President and Publisher: Douglas M. Rife
Associate Publishers: Todd Brakke and Kendra Slayton
Editorial Director: Laurel Hecker
Art Director: Rian Anderson
Copy Chief: Jessi Finn
Proofreader: Elijah Oates
Text and Cover Designer: Abigail Bowen
Acquisitions Editors: Carol Collins and Hilary Goff
Content Development Specialist: Amy Rubenstein
Associate Editors: Sarah Ludwig and Elijah Oates
Editorial Assistant: Madison Chartier
To Summer and Liam: You have my attention.
Acknowledgments
Marzano Resources would like to thank the following reviewers:
Chris Bennett
Executive Director of Middle Schools and Accountability
Cleveland County Schools
Shelby, North Carolina
Carrie Cutler
Clinical Associate Professor University of Houston Houston, Texas
David Pillar
Assistant Director
Hoosier Hills Career Center
Bloomington, Indiana
Molly A. Riddle
Assistant Professor of Elementary Mathematics Education
Indiana University Southeast School of Education
New Albany, Indiana
Bo Ryan
Principal/Solution Tree and Marzano Associate/Author
Ana Grace Academy of the Arts
Middle School
Bloomfield, Connecticut
About the Author
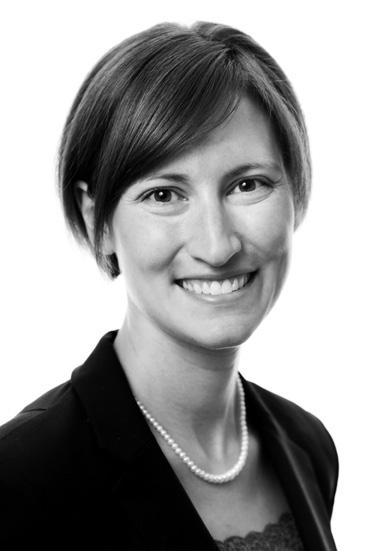
Julia A. Simms is vice president of Marzano Resources. A former classroom teacher, she heads a team that develops research-based resources and provides support to educators as they implement them. Her areas of expertise include effective instruction, learning progressions and proficiency scales, assessment and grading, argumentation and reasoning skills, and literacy development. She has authored or coauthored eleven books, including The Marzano Synthesis , A Handbook for High Reliability Schools , Coaching Classroom Instruction , Questioning Sequences in the Classroom, Teaching Argumentation, Teaching Reasoning, and The New Art and Science of Teaching Reading.
Julia received a bachelor’s degree from Wheaton College and master’s degrees in educational administration and K–12 literacy from Colorado State University and the University of Northern Colorado.
Introduction
In 2014, Mihaly Csikszentmihalyi wrote, “The problem is not how to control children in the classroom, but how to let them have control over their own attention while pursuing goals consonant with the goals of the educational system” (p. 12). In K–12 schools, educators seeking to strengthen student learning and achievement justifiably focus their efforts on assessment, curriculum, and other well-defined areas for which they will find a wealth of evidence-based resources. But growing research, as featured throughout this book, suggests practitioners will greatly benefit from stepping back and thoroughly attending to another fundamental factor at play in student learning: attention itself.
Attention is the gatekeeper to learning. Students must focus on new information to acquire it, organize it, analyze it, and utilize it. Learning a new process or skill requires focused attention during practice. When learning difficulties occur, students who sustain focus and problem-solve are more likely to move forward in their thinking. Attention is a key ingredient for student engagement; students who are not paying attention are unlikely to participate in class discussions, ask or answer questions, or think creatively and critically about the content. Relationships and collaboration are built on the foundation of attentive listening. Focusing on academic goals increases the likelihood of accomplishing them. In short, everything that K–12 educators do in the classroom to promote student learning requires that students pay attention to it for it to be effective. But the science of attention is not simple.
Situated at the intersection of neurological and psychological research, attention theory blends what we know about the physical structures and pathways of the brain with what we know about how students think and process information. To understand how to effectively engage students’ attention, vigilantly protect them from distractions, and productively leverage their working memory, educators must go deeper than simple tips or strategies. If you understand the research on attention and the theories informed and shaped by it, you will be prepared to make real-time decisions that capture, hold, and leverage students’ attention for increased learning. This book is designed to provide that understanding.
Picture an elementary classroom where the teacher, Ms. Garcia, is asking students to demonstrate strategies for multidigit addition, a routine commonly known as number talk.
Although following the instructional protocol for the activity, Ms. Garcia fails to capture all students’ attention or mediate distractions. Sergio and Anaya, distracted by a book they’re interested in, miss the strategies being demonstrated and are unable to meaningfully engage in the subsequent practice activity. Their failure to focus their attention, combined with Ms. Garcia’s failure to capture their attention, has the potential to negatively impact their future mathematics achievement; it could also damage their sense of belonging because of the feelings and possible stigma associated with lack of understanding or skill.
Next, consider a middle school science classroom: As Mr. Thompson explains the procedures for a lab experiment, Jenna is distracted by a notification on her computer desktop. She clicks quickly, laughs at a witty reply to a comment she posted, and misses Mr. Thompson’s warning about the dangers of the diluted hydrochloric acid they’ll be using. As the experiment begins, Jenna opens the vial of hydrochloric acid before putting on her gloves, accidentally spilling some on her hand and burning her skin. Jenna’s susceptibility to distraction creates an unsafe situation, damaging her hand, her learning, and, possibly, the learning of her classmates.
Finally, at the high school level, Ms. Ahmadi has spent several days guiding students through the process of writing compelling college application essays. Aiden, however, is distracted by thoughts of Friday’s football game and the scouts who will be watching him play; he wants to earn a football scholarship at his state’s university. While Aiden’s game play is exceptional, his application essay is not; due to his distraction during instruction— and Ms. Ahmadi’s failure to recognize and mitigate it—Aiden is not offered a scholarship and is unable to attend his desired university.
As illustrated by these instances, almost everything we do in K–12 education involves attention. But, as mentioned previously, attention is not a simple concept, nor is it simple to capture and sustain it in the classroom. As an example of attention’s complexity, consider the debate among cognitive psychologists and neuroscientists about whether attention and consciousness are the same. Many cognitive psychologists equate attention with conscious experience. For example, psychologist William James (1890) stated, “My experience is what I agree to attend to” (p. 402), and Mihaly Csikszentmihalyi (2014)—the psychologist who first described flow experiences—said, “Out of the millions of bits of potential information present in the environment, attention selects an infinitesimal subset and assimilates it into consciousness, thus creating a distinct experience” (p. xvi). But the neuroscientists disagree; they’ll explain that the relationship between consciousness and attention is more complex than simple equivalence. For example, Marvin M. Chun, Julie D. Golomb, and Nicholas B. Turk-Browne (2011)—taking into account the research of Victor A. F. Lamme (2003) and Daniel T. Levin and Daniel J. Simons (1997), respectively—reported that “attention and awareness are not the same” and that “to attend to an object does not ensure awareness” (p. 88). Nevertheless, neuroscientists and psychologists do agree that attention is necessary because the processing capacity of humans (and all other life-forms) is limited (Lindsay, 2020; Oberauer, 2019). Debates such as these are just the tip of the
iceberg in terms of understanding attention, and in this book I argue that understanding attention and how it works is critical for K–12 educators.
Equipped with an in-depth knowledge of attention, educators can not only focus students’ task-related attention by setting optimal, brain-friendly conditions for the classroom but ultimately empower students both in and beyond school. As Jason M. Lodge and William J. Harrison (2019) said, “Increasing our understanding of fundamental attentional processes and how they influence learning in the complex social world will allow educators to develop strategies and tactics for helping students to better manage their own attention” (p. 27). Teachers exert significant influence over what students pay attention to and are uniquely situated to directly teach students the skills they need to focus and manage their attention. Therefore, gaining a comprehensive, interdisciplinary understanding of attention allows us to leverage that influence for maximum student growth. This is what Where Learning Happens is all about.
We will begin by reviewing the available research on attention in chapter 1. While this can be a complex topic, we will approach it as straightforwardly as possible. Then, with chapters 2 through 5, we will dive into the practical applications of that research, exploring how educators can leverage attention-related concepts and theories to help students more effectively achieve their own goals and the goals of the educational system. Chapter 2 offers strategies teachers can use to empower students to deal with distractions— including digital distractions—and debunks the myth of multitasking. Chapter 3 provides approaches teachers can use to create an atmosphere of attention in their classrooms. Chapter 4 transitions to ways teachers can design instruction and instructional materials to capture students’ attention and avoid overloading their working memories. Chapter 5 emphasizes the importance of context: What captures students’ attention or helps them learn in one situation might be less effective in another situation. This final chapter guides teachers to strategically match attentional strategies and instructional approaches to their specific contexts and the unique needs of students.
As always, please accept the strategies presented in this book as high-probability strategies. That is, they have a high probability of producing desired student outcomes given their foundation in the available research. However, context is critically important—the context of your school or classroom may include factors that impact the effectiveness of any given strategy. Therefore, as you implement strategies, please collect data to see whether they are having the desired effects. This can be as simple as the following process.
1. Identify your desired outcome: For example, “Students will check digital notifications in class less frequently.”
2. Collect preimplementation data: For example, have students keep track of how many times they check a digital notification during class (perhaps by keeping a simple tally on a sticky note on their desk).
3. Implement your strategy: For example, teach students about the negative consequences of distractions and how to avoid distractions (as described in chapter 2, page 19).
4. Collect postimplementation data: You can use the same data collection approach you used during step 2, such as a simple student-recorded tally of digital notification checks.
5. Analyze the data: Did your strategy get you closer to your desired outcome (fewer digital notification checks in class)?
If this process shows the strategy is working, excellent and congratulations! If not, please either adapt the strategy until it produces the desired outcomes or try a different strategy. As Robert J. Marzano (2009) stated:
Educators must always look to whether a particular strategy is producing the desired results as opposed to simply assuming that if a strategy is being used, positive results will ensue. If a strategy doesn’t appear to be working well, educators must adapt the strategy as needed or use other strategies. (p. 35)
My hope is the research and strategies presented here will prove useful to you as you strive to leverage attention for greater student learning in your classroom.
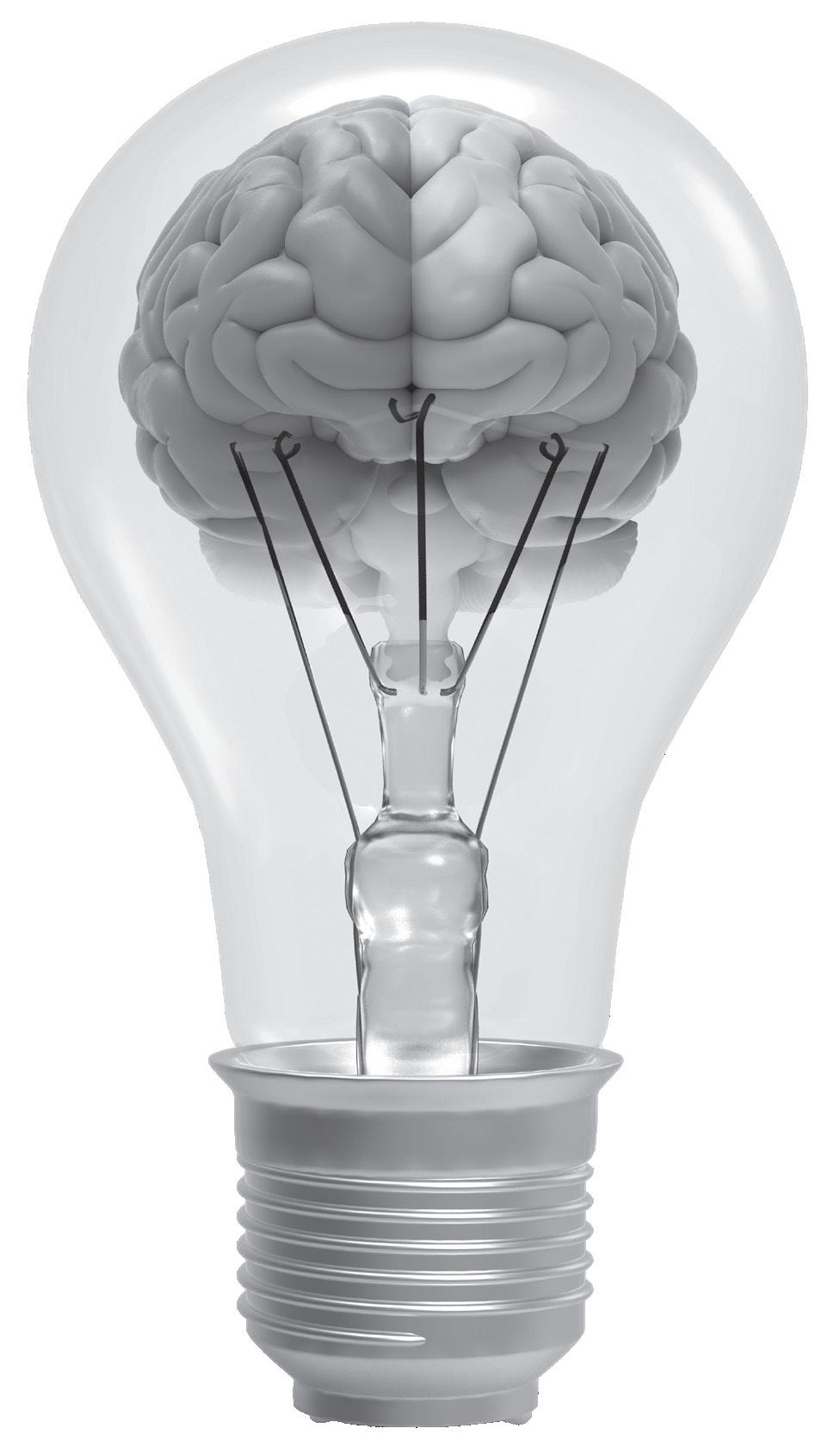
Focusing on Attention Research
To fully appreciate our capacity as educators to strengthen student learning through sensitivity to attention, it helps to understand the history of attention research and related technological advancements. This background conveys both the value of this area of focus and why its potential with regard to classroom application may seem untapped. At the beginning of the 21st century, cognitive neuroscientists Amir Raz and Jason Buhle (2006) compiled a timeline of landmarks in the study of attention from the late 19th century onward. Attention studies began as early as 1868, when Dutch ophthalmologist Franciscus Donders first attempted to measure attention. In the late 19th and early 20th centuries, psychologists such as William James included attention in their studies of human thought and behavior. Unfortunately, in the 1920s, the rise of behaviorism put a damper on attention research for several decades. Behaviorism placed emphasis on behaviors that could be observed; because attentional processes are not directly observable, the field was neglected. However, after World War II, cognitive psychology reengaged with attention studies. Michael I. Posner (as cited in gocognitive, 2011) posited that failures of attention during World War II—such as soldiers losing focus while monitoring radar or military disasters precipitated by attempts to multitask—were one of the reasons for the reinvigoration of attention research. Later, in the 1980s, the rise of neuroimaging technology brought new neuroscientific perspectives to the study of attention, leading to substantial growth and discovery in the field over the ensuing decades.
Neuroimaging technology has unlocked a wealth of information educators can use to transform their students’ learning. Before the advent of this technology, attention and its role in learning were often studied using classroom observations, student self-reports, and adult reports about students’ behaviors. Classroom observations were unreliable; a student might be looking at the teacher but contemplating social plans, snack choices, or a love
interest (Keller, Davidesco, & Tanner, 2020). Student self-reports had at least two drawbacks: (1) asking students to report on their attention during a lesson distracted them from the lesson, and (2) asking students to report on their attention after a lesson resulted in poor remembrance or reluctance to report lapses in attention (Robison, Miller, & Unsworth, 2020). Finally, adult reports about students’ behavior were subject to similar socialdesirability biases and tended to focus on broad behaviors rather than attention-specific skills and abilities (Gallen et al., 2023). Since at least 2000, neuroimaging, as well as eye-tracking technology, has facilitated considerable growth and progress in the field of attention studies, giving rise to nuanced understandings of attentional networks and providing a neurological basis for the practical, psychologically grounded ways we might conceive of the attention types we can modulate in the classroom. Because of the close intertwining of neuroscience and cognitive psychology in attention research and theory after 2000, we will begin our discussion of attention in this chapter with a brief review of the major neuroimaging techniques and their uses for attention research, as well as a basic diagram of the brain and its structures and areas. From there, we will explore eye tracking, attentional networks, the means to measure attention, and the major types of attention.
Neuroimaging
Since the 1980s and 1990s, cognitive neuroscience—the study of the brain’s structures, functions, and activity—has benefited from significant progress in techniques that noninvasively observe the brain through neuroimaging (Litwińczuk, Trujillo-Barreto, Muhlert, Cloutman, & Woollams, 2023). As Tomoyo Morita, Minoru Asada, and Eiichi Naito (2016) explained, neuroimaging “enabled the measurement and imaging of brain activity without injuring the brain while an individual was engaged in mental and/or physical activities” (p. 1). Neuroimaging is generally used to measure (1) electrical activity of specific cell groups in the brain and (2) changes in blood flow to the brain during cognitive activity (Morita et al., 2016). Table 1.1 shows which of the most widely used neuroimaging techniques are used for each purpose, along with the abbreviation used for each technique.
Table 1.1: Neuroimaging Techniques
Electrical Activity
Electroencephalography (EEG)
Magnetoencephalography (MEG)
Changes in Blood Flow
Near-infrared spectroscopy (NIRS)
Functional magnetic resonance imaging (fMRI)
Positron emission tomography (PET)
Let’s take a closer look at what each of the five techniques entails, focusing first on those that measure electrical activity before reviewing those that capture changes in blood flow.
1. Electroencephalography (EEG): Electrodes that detect oscillating voltage signals in the brain (picture a horizontal line with peaks and valleys at intervals) are placed on the scalp. Research has indicated that signals at particular frequencies (8–13 hertz; known as the alpha band) are associated with shifts
in attention. Fluctuations in the voltage signals can be measured in milliseconds, providing a precise timeline of attentional shifts in the brain (Keller et al., 2020; Morita et al., 2016; Payne & Sekuler, 2014; Van Diepen, Foxe, & Mazaheri, 2019).
2. Magnetoencephalography (MEG): A helmet that contains superconducting quantum interference devices, or SQUIDs, is placed on the head; these sensors detect the faint magnetic fields generated by electrical activity in the brain. Magnetic fields are more easily detected through the skull and scalp than electrical fields, which allows for locations within the brain to be identified with more precision. However, for SQUIDs to work properly, they must be kept close to absolute zero (−459 degrees Fahrenheit) and housed in a magnetically shielded room, making MEG systems expensive to purchase and maintain (Feys et al., 2022; Hegazy & Gavvala, 2022; Morita et al., 2016).
3. Near-infrared spectroscopy (NIRS): Placed on the scalp are sensors called optodes, some of which shine near-infrared light into the head. This light can penetrate several centimeters through the skin, skull, and protective brain fluid and follows a curved path back to the surface of the head, where other optodes receive and measure it. Because molecules in various brain tissues called chromophores absorb different amounts of light, these measurements provide insight into the structure and timing of brain functions (Gervain et al., 2011; Grossmann, Oberecker, Koch, & Friederici, 2010; MinagawaKawai, Mori, Hebden, & Dupoux, 2008; Morita et al., 2016; Otsuka, 2014; Taga, Asakawa, Maki, & Koizumi, 2003).
4. Functional magnetic resonance imaging (fMRI): The body is placed inside a scanner that creates a strong magnetic field, forcing the body’s protons to align with that field. Then, the scanner sends radio waves through the body, nudging the aligned protons and making them wobble (called precession); protons in different kinds of tissue wobble differently, allowing the scanner to create a detailed picture of brain structures. When a brain area becomes active, it requires more oxygen, and blood flow increases to that area. This increase in blood flow influences proton precession, providing detailed information about the location of brain activity (Crosson et al., 2010; Glover, 2011; Gruber, Froeling, Leiner, & Klomp, 2018; Morita et al., 2016; Schramm et al., 2023).
5. Positron emission tomography (PET): A small dose of a radioactive molecule (called a radiotracer) is ingested or injected into the body; the type of radiotracer is determined by the target organ or process (brain scans typically use fluorodeoxyglucose). The body is then placed inside a scanner. When the radiotracers reach the target area, they begin to decay and produce small amounts of energy that the scanner detects and uses to create images (Berti, Mosconi, & Pupi, 2014; Crișan et al., 2022; Jiang, Chalich, & Deen, 2019; Mauss & Robinson, 2009; Shukla & Kumar, 2006; Zhu, Lee, & Shim, 2011).
In general, techniques that measure electrical activity (EEG and MEG) can precisely detect changes in brain activity over time (that is, they have high temporal resolution), but their identification of the location of brain activity is less precise (they have low spatial resolution). Techniques that measure changes in blood flow (fMRI, NIRS, and PET) have the opposite profile: They have low temporal resolution (less precise measurements of changes over time) and high spatial resolution (very precise measurements of brain activity locations; Crosson et al., 2010). Table 1.2 lists additional benefits and challenges of each technique.
Table 1.2: Comparison of Neuroimaging Techniques
Electroencephalography (EEG)
Magnetoencephalography (MEG)
Near-infrared spectroscopy (NIRS)
Milliseconds Poor Fair Fair
Milliseconds Poor Fair High
Tens of milliseconds Fair Easy Low
Functional magnetic resonance imaging (fMRI) Seconds Good Difficult High
Positron emission tomography (PET)
Tens of seconds Good Difficult High
Source: Crosson et al., 2010; Morita et al., 2016; Quaresima & Ferrari, 2019; Sakai, 2022.
Given the benefits and challenges shown in table 1.2, cognitive neuroscientists typically select the technique that best fits their needs and resources, or they might use two or more techniques together to achieve the necessary detail (Ebrahimzadeh et al., 2022; Fernandez-Iriondo et al., 2022; Warbrick, 2022). Chiahui Yen, Chia-Li Lin, and MingChang Chiang (2023) provided an example:
fMRI and EEG are the most widely used and well-established neuroimaging techniques. fMRI and EEG provide complementary information about brain activity. fMRI measures changes in blood oxygen levels, allowing brain activity to be assessed with excellent spatial resolution. In contrast, an EEG records the brain’s electrical activity with high temporal resolution. By focusing on these two techniques, we can better understand brain dynamics, integrating the spatial and temporal aspects of brain function. (p. 2)
When working with children, NIRS and newer forms of EEG are often preferred because they are portable and less sensitive to movement than MEG, fMRI, and PET (Morita et al., 2016).
Regardless of which technique cognitive neuroscientists use, they are typically collecting data on what is happening in the brain and where it is happening. Therefore, to understand their work, we also need a broad understanding of the geography of the brain. Figure 1.1 depicts the major brain areas and structures.
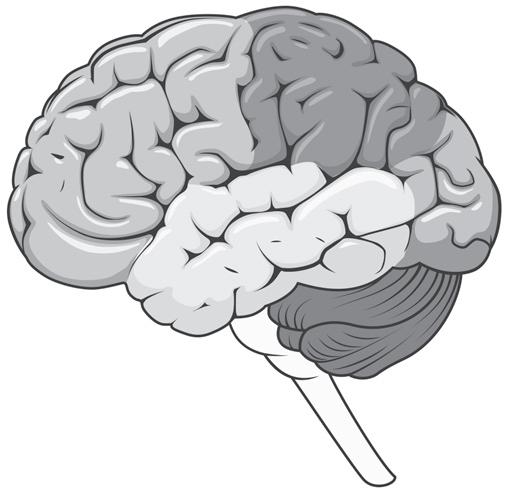
Figure 1.1: Geography of the brain.
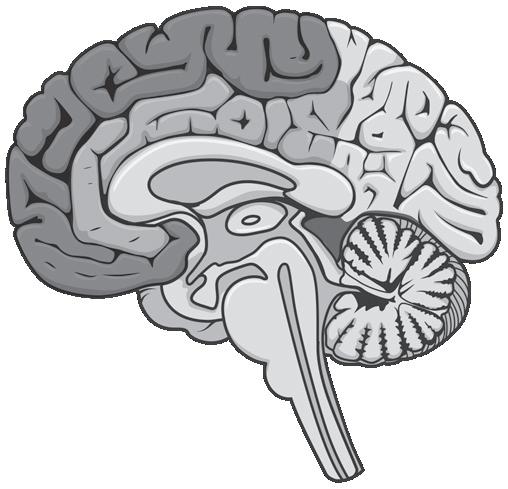
Cognitive neuroscientists are still investigating and discovering additional brain areas involved with attention (for example, Nakajima, Schmitt, & Halassa, 2019), but most agree that attention is managed by a neural network extending between the parietal, temporal, and frontal brain areas (everywhere except the back of your head). This network is closely linked to brain areas that process sensory signals, which makes sense given the relationship between sensory stimuli and attention. Lodge and Harrison (2019) explained that “given the important role of attention in guiding decisions and actions, the neural areas involved in attention are heavily connected to brain areas involved in processing basic sensory signals and the planning and generation of motor actions” (p. 24). Neuroimaging paints a fascinating picture of the brain’s attention-related processes and structures, but it is not the only way to measure attention. Given the close association between attention and visual gaze, eye tracking is another commonly used approach.
Eye Tracking
Where we look is often (but not always) an indication of where our attention is focused (Busjahn et al., 2014; Stern & Shalev, 2013). As Andy J. King, Nadine Bol, R. Glenn Cummins, and Kevin K. John (2019) observed, there is “a direct link between where one looks and what one cognitively attends” (p. 150). Early ideas about connections between the eyes and mind (Just & Carpenter, 1980) led to experiments in the 1990s and early 2000s that found that visual gaze often correlates with attentional focus (Chelazzi et al., 1995) and that eye movements are a mechanism for allocating and managing attention (Corbetta & Shulman, 2002; Rizzolatti, Riggio, & Sheliga, 1994; Slykhuis, Wiebe, & Annetta, 2005).
Occipital
However, the correlation between gaze and attention is not perfect; as conveyed at the opening of the chapter, just because a student is looking at the teacher does not guarantee their attention is on them. A student looking out the window or staring into space might be thinking about a question the teacher asked or a topic related to the content at hand (Rosengrant, n.d.). Nevertheless, research by Keren Shavit-Cohen and Elana Zion Golumbic in 2019 found that looking away from a speaker resulted in worse comprehension than keeping one’s eyes on the speaker. Thus, while not an ironclad indicator of attention, visual gaze can provide valuable information about attentional focus and is often captured using eye-tracking technology.
Eye-tracking devices use specialized glasses or small cameras attached to computer, tablet, or smartphone screens. These cameras capture images of the eyes at extremely high frame rates, yielding data about what individuals are looking at (fixations), how long they look at it (dwell time), and when they shift their gaze to something different (saccades; Frutos-Pascual & Garcia-Zapirain, 2015; Kohout, Kruikemeier, & Bakker, 2023; Rosengrant, n.d.). Saccades typically happen several times per second; this is because high-resolution vision (or central vision) is available only on a specific area of the retina (called the fovea), so we must shift our eyes to bring specific objects into focus (Lindsay, 2020). Lodge and Harrison (2019) explained:
Central vision, as opposed to lower-resolution peripheral vision, is the only part of the visual field that has the visual resolution necessary for many common visual tasks, such as reading, recognizing faces, or watching television. Humans thus make approximately two to four saccades per second to move objects of interest into central vision, resulting in a greater attentional focus for those objects than for objects in peripheral vision. (p. 25)
Whatever is in our central vision is the object of our overt attention; we can pay overt attention to only one thing at a time. In contrast, we can pay covert attention to several locations in our peripheral vision simultaneously; we perceive multiple movements and objects around us without looking directly at them (Kowler, 2011; Nakayama & Martini, 2011). Visual attention researchers, such as Marisa Carrasco (2011), have found that covert and overt attention work cooperatively:
Humans deploy covert attention routinely in many everyday situations, such as searching for objects, driving, crossing the street, playing sports and dancing. Covert attention allows us to monitor the environment and guides our eye movements (overt attention) to locations of the visual field where salient and/or relevant information is. (p. 1487)
Covert attention has ramifications for the K–12 classroom. Subtle cues and gestures, such as silent signals for needing help or bathroom breaks, can be used to manage the classroom while minimizing disruptions. Posting news or updates in a consistent spot in the classroom allows students to covertly check to see if there is anything new that might require their overt attention.
While eye-tracking technology has many benefits—noninvasive; objective; and much easier, cheaper, and more precise than manual observation and coding (Hessels & Hooge, 2019; Holmqvist & Andersson, 2017)—it’s not perfect. Until the mid-2010s (when the technology became portable), most eye-tracking studies were conducted in laboratories, making it difficult to generalize results to classroom settings (Fuhl, Tonsen, Bulling, & Kasneci, 2016; Keller et al., 2020). Also, Marcus Nyström, Richard Andersson, Kenneth Holmqvist, and Joost van de Weijer (2012) found that the accuracy and precision of eye-tracking data could vary depending on the equipment operator, the calibration method and frequency, and even the eye color of the user. Still, given the close association between visual gaze and attention, it can be a useful technique. In fact, taken together with neuroimaging technology, it has helped fuel the field of attention studies and given way to the breadth of research educators can apply to the classroom, including the description of neural attentional networks.
Attentional Networks
Perfect agreement on the functions and mechanisms of attention in the brain does not exist. There are a number of models of attention, each with nuanced theories and sometimes conflicting findings about the neural basis of attention and the neural regions, structures, and neurotransmitters involved in the process (for example, see Corbetta & Shulman, 2002; Desimone & Duncan, 1995; Pessoa, Kastner, & Ungerleider, 2003). However, there is some consensus around the major components of attentional networks, especially since the rise of neuroimaging has allowed cognitive neuroscientists to observe brain activity with far more accuracy than previous techniques allowed. Perhaps the most influential model of attentional networks in the brain was first articulated by Michael I. Posner and Steven E. Petersen in 1990. In their 2012 update of the model, Petersen and Posner described the functions and brain locations of the model’s three networks: (1) the alerting network, (2) the orienting network, and (3) the executive control network.
Alerting Network
The alerting network is like a security guard; it pays attention and waits in readiness to act if something happens; its primary job is to be sensitive to incoming stimuli. This readiness comes in two different flavors: Phasic alertness is readiness in relation to a specific task. For example, if you let students know that you’ll be randomly selecting one of them to answer a question about information you’re about to present, they will likely activate phasic alertness. They will pay attention to the information in order to be ready to answer the question. Tonic alertness is a general, task-agnostic sense of readiness. Tonic alertness might cause a student to notice their name when it is spoken in a crowded lunchroom or commons area.
In terms of brain structures, the alerting network is primarily located in the frontal and parietal lobes (front and top of the head; see figure 1.1, page 9). Fascinatingly, the alerting network does not seem to be equally distributed between the left and right hemispheres of the
brain; it is “heavily lateralized to the right hemisphere” (Petersen & Posner, 2012, p. 75). That is, people with injuries to the right hemisphere of their brain profoundly fail to notice things happening on their left side, but left-brain injuries do not produce commensurate neglect of the right side. In terms of research, the alerting network is the least studied of the three.
Orienting Network
The classic metaphor for the orienting network is that of a spotlight; it decides which incoming stimuli will receive attentional priority. It might turn attention toward one stimulus while ignoring others. There are several reasons why the orienting network might decide to prioritize a stimulus. With goal-driven influences, the stimulus might be important for a specific task or to reach a goal. For example, if a teacher instructs students to search for specific information in a text or visual (such as finding a peninsula on a map), the orienting network prioritizes the target feature (land almost surrounded by water). With stimulus-driven influences, the stimulus itself might be very compelling (bright color, loud noise, and so on); in the classroom, teachers often present important words or phrases in large, bold, or brightly colored fonts to take advantage of stimulusdriven prioritization. With experience-driven influences, the stimulus might have been previously rewarded or previously signaled a threat. Many positive behavior support interventions use experience-driven stimuli—receiving or seeing others receive awards for desirable behaviors—to help students’ orienting networks prioritize specific actions and behavioral choices. We further discuss these three different influences and their applications to K–12 education in chapter 2 (page 19).
The orienting network is located largely in the parietal (upper back) lobe and in areas of the temporal and frontal (sides and upper front) lobes that closely correspond to the senses, such as the frontal eye field in the frontal lobe (see figure 1.1, page 9). The orienting network is probably the best studied of the three networks.
Executive Control Network
The executive control network is just what it sounds like; in the same way that a business executive might keep an organization running smoothly by analyzing data, selecting actions, making decisions, planning, reflecting, and so on, the executive control network is responsible for keeping the brain running smoothly from an attentional perspective. In K–12 education, the executive control network is often referred to using terms such as metacognition, dispositions, or habits of mind; it is used when students regulate their performance on specific tasks, including goal setting, planning, monitoring, evaluating, and inhibiting unproductive responses. In the neuroscience literature, the executive control network is most often described as the network that “manages the ability to solve conflictual information” (Giovannoli, Martella, & Casagrande, 2021, p. 1) or described using terms such as “control of goal directed behavior” and “conflict resolution” (Peng & Miller, 2016, p. 78). It handles cognitive tasks such as error detection, selfregulation of thoughts or emotions, and resistance of habitual responses, among others. For example, a student writing an essay for an exam uses the executive control network to
analyze the essay question, decide what information to include in their response, and— throughout the exam—inhibit their fear response to testing situations.
A classic task used to measure the executive control network is called the Stroop task (Stroop, 1935). It highlights the conflict resolution characteristics of the executive control network. In this task, an individual is given a list of color names such as red, blue, yellow, green, and so on. However, the words are printed in ink that is a different color than the text indicates. The word red might be printed in green ink, blue might be printed in purple ink, and so forth. The individual is asked to name the ink colors; success requires the executive control network to ignore the printed words and focus on the color of the ink.
Probably the most important brain structure for the executive control network is called the anterior cingulate cortex; it is found deep inside the front part of the brain, close to structures associated with the limbic system (which handles emotions; see figure 1.1, page 9). This makes sense, given Michael I. Posner and Mary K. Rothbart’s (2007) description of the executive control network as responsible for “monitoring and resolving conflict among thoughts, feelings, and responses” (p. 7). However, other brain areas are also involved in executive control, including the prefrontal cortex in the frontal lobe (behind your forehead). The executive control network has been studied more than the alerting network but not as much as the orienting network.
Measurement of Attention
With a model of attentional networks in place, it becomes possible to measure the efficiency of those networks and explore the relationships between them. In 2002, Jin Fan, Bruce D. McCandliss, Tobias Sommer, Amir Raz, and Michael I. Posner developed the attention networks test. It is a thirty-minute test that can be used with children, adults, and even nonhuman primates. As might be supposed, it consists of three measures: (1) alerting, (2) orienting, and (3) executive control.
1. Alerting measure: The participant sees a cue that a target is about to appear but doesn’t indicate where the target will appear. When the target appears, the participant responds as quickly as possible to indicate its location.
2. Orienting measure: The participant sees a cue that indicates both the imminent appearance of a target and where the target will appear. When the target appears, the participant responds as quickly as possible to indicate its location.
3. Executive control measure: The participant sees three subsequent conditions—the first presents two congruent stimuli (for example, the word red printed in red ink), the second presents two neutral stimuli (for example, the word knife printed in blue ink), and the third presents two incongruent stimuli (the word red printed in green ink). The participant names one of the stimuli (such as the ink color) in all three conditions as quickly as possible.
In 2014, Joan P. Pozuelos, Pedro M. Paz-Alonso, Alejandro Castillo, Luis J. Fuentes, and M. Rosario Rueda used the attention networks test to investigate how each of the
attentional networks develop across childhood and whether there were interactions between the networks. As they explained, “although the attention networks are associated with distinct brain circuits, there is evidence suggesting that they are not functionally independent” (p. 2406). Indeed, they found that children between six and twelve years old exhibited interactions between the alerting and orienting networks and between the orienting and executive control networks. This suggests that building up a child’s alerting network could strengthen the orienting network, and improving the orienting network’s function could have positive impacts on the executive control network.
In the classroom, strengthen your students’ alerting networks by maintaining consistent routines and by using schedules and timers to help students understand and track the structure of the day. Ensuring that the classroom is well-lit with calming colors—and avoiding over-decorating—also supports positive functioning in the alerting network. Relative to the alerting network, Pozuelos and colleagues (2014) found that younger children (six to seven years old) benefited more than older children (eight to twelve years old) from warning cues before targets were presented. They explained:
Larger alerting scores indicate that young children benefit more than older children from having a warning auditory signal prior to the presentation of the target. This is due to their slower responses when no auditory tone is presented, which is indicative of young children’s greater difficulties in maintaining an optimal level of tonic alertness in absence of warning cues. (p. 2411)
Essentially, younger children benefit from being told that important information is about to be presented more than older children do. As many primary teachers already know, using auditory signals, such as a chime, helps students prepare for transitions, which require them to reorient their attention.
To support your students’ orienting networks, maintain an organized classroom with clearly defined areas for different activities; labels and visual cues help students quickly focus on the important materials and information needed for a task. When using instructional tools such as worksheets, manipulatives, or technology, avoid overloading students by asking them to use multiple tools at once. Their orienting networks will function most productively if they are able to maintain focus on a single information source or recording location.
The Major Types of Attention
Although the introduction opened with some psychological perspectives on attention, we have thus far focused mainly on neuroscientific conceptualizations of attention. To conclude this chapter, we will address psychological perspectives on attention. Whereas neuroscientific perspectives offer a detailed understanding of the brain structures and neural pathways involved in attention, cognitive psychology focuses on how attention manifests in students’ thinking and behavior. Because of this, cognitive psychology
perspectives are often more readily applicable to K–12 education and classroom practices. However, as we will see in the subsequent chapters, neuroscientific evidence supports and reinforces the hypotheses of cognitive psychology and vice versa. For example, one of the topics we will discuss in depth in chapter 4 (page 69) is working memory. Briefly, working memory is where information is temporarily stored and manipulated during learning. Neuroscientific evidence supports cognitive perspectives on working memory by identifying brain regions associated with working memory processes (such as the hippocampus, which is part of the limbic system, and portions of the prefrontal cortex; see figure 1.1, page 9). These findings, in turn, are used by cognitive psychologists to nuance their understanding of how learned information proceeds from working memory to long-term memory and is stored for later use.
Another instance in which neuroscientific findings have helped clarify cognitive psychological perspectives relates to defining different types of attention and their accompanying behaviors. Eadaoin J. Slattery, Eoin O’Callaghan, Patrick Ryan, Donal G. Fortune, and Laura P. McAvinue (2022) assert that “the term ‘attention’ is a broad category and has limited descriptive value. This is because attention is not a unitary construct but rather comprises several distinct components” (p. 3). Indeed, psychologists flexibly use the word attention to describe various attention-related concepts:
1. A limited resource used to process information (for example, Wickens, 1980)
2. The process of selecting information to focus on (for example, Desimone & Duncan, 1995)
3. The mechanism that determines how information will be processed (for example, Carrasco, Ling, & Read, 2004)
4. The underlying brain structures that give rise to it (Lindsay, 2020)
To help clarify the issue for our K–12 education purposes, figure 1.2 presents some of the major types of attention found in psychological attention literature and how they generally relate to each other.
Figure 1.2: Continua of
The first continuum describes the sustained versus transient aspect of attention (Chevet, Baccino, Marlot, Vinter, & Drai-Zerbib, 2022; Chun et al., 2011; Gallen et al., 2023; Lindsay, 2020; McDowd, 2007; Posner & Petersen, 1990; Slattery et al., 2022). Sustained attention involves focusing on a specific stimulus or activity for an extended period of time; it is sometimes called vigilance. In contrast, transient attention lasts for shorter periods (generally about several seconds). In the classroom, students might exhibit behaviors anywhere along the continuum of sustained and transient attention while, for example, watching an instructional video. Elias, displaying sustained attention, watches the video carefully, listening to the narration and noticing subtle changes and details portrayed in the visuals. Sakura, displaying transient attention, briefly focuses on the video when bright colors, large-scale movements, or loud sound effects occur, but otherwise pays attention to other classroom stimuli such as friends and noises from the hallway.
The second continuum describes the selective versus divided aspect of attention (Broadbent, 2013; Chevet et al., 2022; Kahneman, 1973; McDowd, 2007; Treisman, 1964; Wickens, 2002, 2008). Selective attention is the ability to focus on a specific stimulus or activity while simultaneously ignoring other information. Divided attention describes situations in which we attempt to split our focus between two or more stimuli or activities at the same time. For example, during an art class, Aisha exhibits selective attention by focusing solely on her painting, while Javier exhibits divided attention, chatting with his neighbor, reading a notification on his smartwatch, working on his painting, and then chatting with his neighbor again.
The third continuum describes the external versus internal aspect of attention (Chun et al., 2011; Johnson, 1983; Kane & Engle, 2003; Monsell, 2003; Keller et al., 2020; Miller & Cohen, 2001; Oberauer, 2019; Pashler, 1994; Yeung, Botvinick, & Cohen, 2004). External attention focuses on information from the outside world that is perceived through our senses; it is also referred to as perceptual attention. Internal attention focuses inward and may include the contents of memory, emotions, tasks, or plans; it is sometimes called central or reflective attention. In the classroom, both internal and external attention can be productive. For example, during a direct instruction lesson, Ravi exhibits external attention by looking at the teacher and listening carefully to her description of a mathematical algorithm, jotting notes in his notebook as the teacher explains the process. Keith, on the other hand, exhibits internal attention; he closes his eyes and mentally rehearses the algorithmic process in his head, noting steps that he would like to tweak and ways to make the process faster and more accurate.
To connect these psychological descriptions of attention back to the neuroscientific literature, notice the role of each of Petersen and Posner’s (2012) neural networks in each continuum of attention. The alerting network detects distractions that have the potential to disrupt sustained and selective attention. If the orienting network prioritizes a distraction over the current focus of attention, a shift occurs, which can result in transient or divided attention. The executive control network maintains the larger point of view, helping to determine if the orienting network should prioritize a distraction, depending on
current goals and plans. As mentioned previously, understanding this interplay between types of attention and the neural networks that direct them is helpful for both educators and students. For students, this understanding can make them better able to regulate their attention, manage distractions, and optimize their learning experiences. For educators, these insights can help them teach more effectively, support neurodiverse learners, promote student engagement, and address attention-related challenges in the classroom.
Summary
In this chapter, we explored the research on attention from the perspectives of both neuroscience and cognitive psychology. From neuroscience, we discussed neuroimaging, eye tracking, and Petersen and Posner’s model of neural networks; from cognitive psychology, we articulated definitions of some key attentional concepts. Combining these two threads is important to create a nuanced and strategic picture of how student attention functions in K–12 classrooms. Educators who grasp the inner workings of attention networks in the brain and understand how the brain’s attention processes manifest in students’ behavior and actions can design instruction that aligns with how students’ brains focus on and process information. From a classroom management perspective, educators who understand attentional mechanisms can leverage those mechanisms to capture and sustain students’ interest and motivation for learning. Finally, educators who understand attention can help students understand how their brains work and empower them to consciously manipulate their attention to better achieve their own goals and desired outcomes.
To that end, in chapters 2 and 3 (page 19 and page 45), we will focus on the two major categories of attention that are pertinent to helping students manage their own attention: (1) sustained attention and (2) selective attention. We will save our discussion of how teachers can design instruction that aligns with how students’ brains focus on and process information for chapters 4 and 5 (page 69 and page 95). There, we will switch our focus to the internal-external attention continuum, examine working memory as the place where internal and external stimuli meet, and discuss how teachers can manage working memory to enhance learning.
Questions for Discussion and Reflection
1. What aspects of attention have you previously studied or learned about as an educator?
2. Are you more familiar with neuroscientific or cognitive psychology perspectives on attention?
3. Where do you typically focus your eyes when your attention is turned inward or when you are thinking?
4. What are some things you do in your classroom to help students maintain alertness?
5. What are some things you do in your classroom to help students orient their attention appropriately?
6. What are some questions you might ask students to help them become more aware of their executive control network?
7. What is a recent example of a student displaying sustained attention? Transient attention? Selective attention? Divided attention?
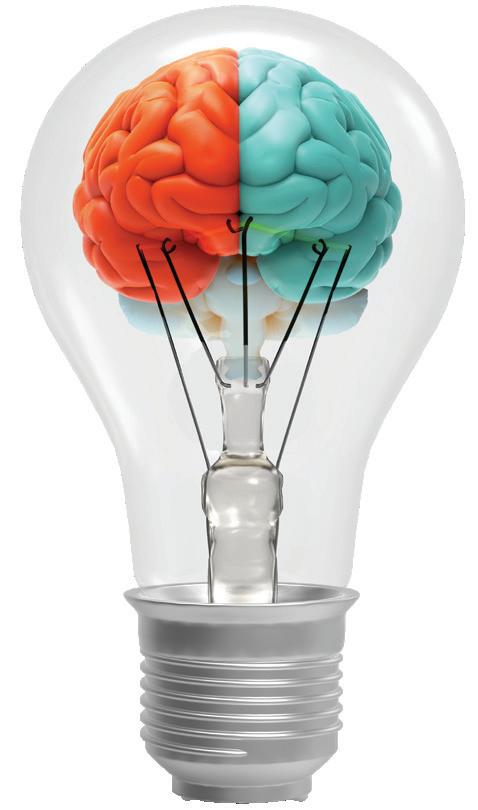
With many distractions competing for students’ focus in today’s highly stimulating world, capturing their attention is paramount to learning. So what do psychology and neuroscience tell us about how educators can craft attention-sensitive instruction? In Where Learning Happens, Julia A. Simms thoroughly explores the research on types of attention, cognitive load theory, information-processing principles, and other key concepts and offers evidence-affirmed strategies to maximize student engagement. By understanding working memory as the locus of learning, classroom teachers can leverage their knowledge of attention to help students achieve their academic goals.
K–12 TEACHERS WILL:
z Understand how to create a classroom atmosphere conducive to attention
z Learn the evolution of attention-related research and its practical applications
z Bolster students’ working memory by considering cognitive load theory, informationprocessing principles, and instructional design
z Gain knowledge of cognitive attention training, state training, and effective attention strategies
z Leverage an attention-centered instructional approach to help students achieve goals
“Julia A. Simms has masterfully synthesized decades of research on attention, distraction, and the brain into a compelling and practical guide for educators. Each chapter is packed with actionable insights that will enhance student learning and help educators create an atmosphere of focused attention. This book is not just relevant; it’s essential for anyone dedicated to the craft of teaching and the pursuit of learning.”
—BRET HELLER
Superintendent, School Town of Munster, Indiana
“Where Learning Happens deconstructs what it is we actually mean when we invite students to ‘pay attention.’ After reading this book, I have a much deeper understanding of how my instructional choices do (and don’t) foster the kind of attention that actually facilitates learning.”
—MARK GARDNER
English Language Arts Teacher, Hayes Freedom High School, Camas, Washington
“Simms’s focus on cultivating a culture of attention and managing distractions is incredibly timely given our tech-driven, post-pandemic classrooms. As a principal, I’m eager to implement these ideas to support my teachers and enhance student success.”
—NANCY MILLS Principal, Harris Park Elementary School, Westminster, Colorado