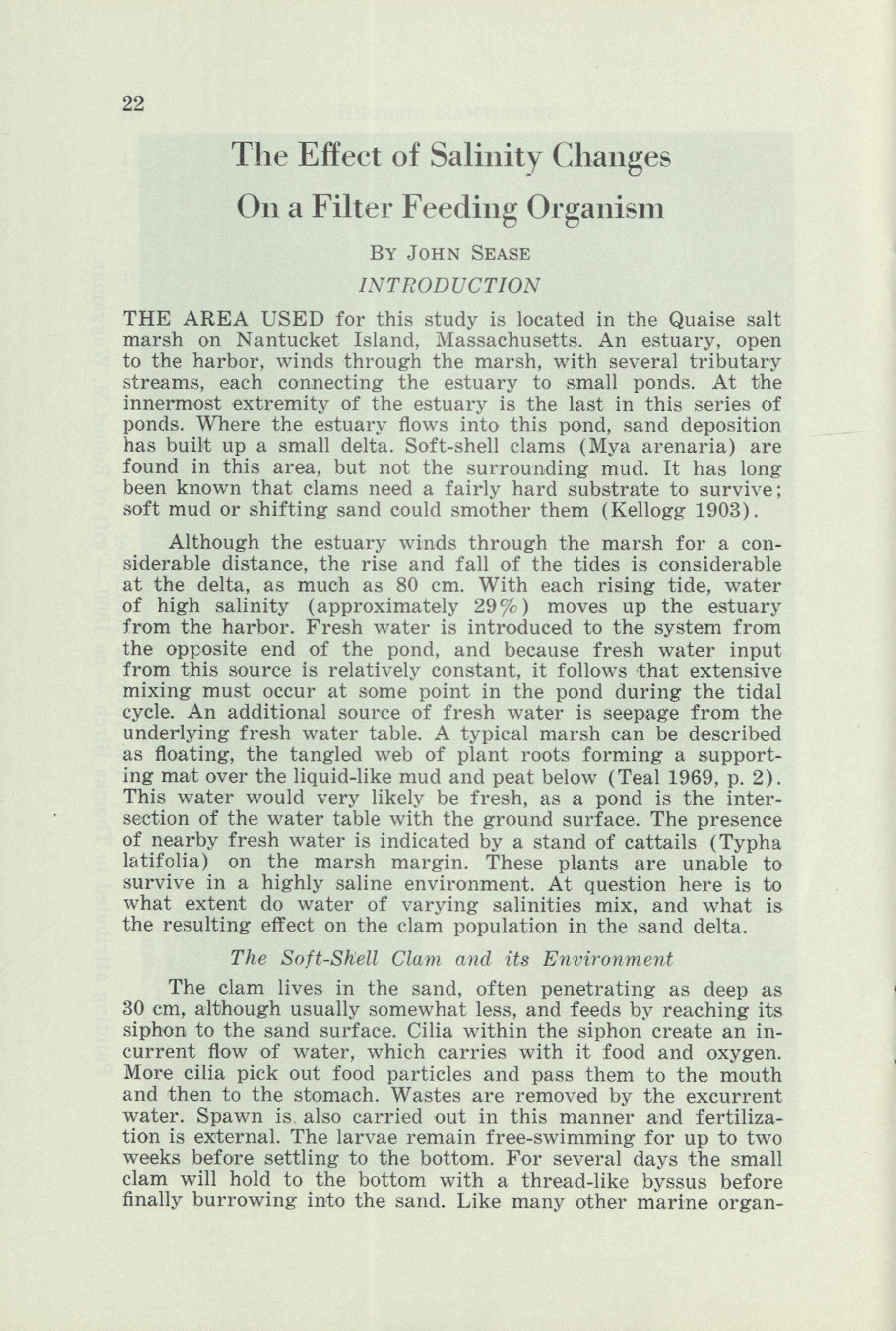
12 minute read
The Effect of Salinity Changes on a Filter Feeding Organism
22
BY JOHN SEASE INTRODUCTION THE AREA USED for this study is located in the Quaise salt marsh on Nantucket Island, Massachusetts. An estuary, open to the harbor, winds through the marsh, with several tributary streams, each connecting the estuary to small ponds. At the innermost extremity of the estuary is the last in this series of ponds. Where the estuary flows into this pond, sand deposition has built up a small delta. Soft-shell clams (Mya arenaria) are found in this area, but not the surrounding mud. It has long been known that clams need a fairly hard substrate to survive; soft mud or shifting sand could smother them (Kellogg 1903).
Although the estuary winds through the marsh for a considerable distance, the rise and fall of the tides is considerable at the delta, as much as 80 cm. With each rising tide, water of high salinity (approximately 29%) moves up the estuary from the harbor. Fresh water is introduced to the system from the opposite end of the pond, and because fresh water input from this source is relatively constant, it follows that extensive mixing must occur at some point in the pond during the tidal cycle. An additional source of fresh water is seepage from the underlying fresh water table. A typical marsh can be described as floating, the tangled web of plant roots forming a supporting mat over the liquid-like mud and peat below (Teal 1969, p. 2). This water would very likely be fresh, as a pond is the intersection of the water table with the ground surface. The presence of nearby fresh water is indicated by a stand of cattails (Typha latifolia) on the marsh margin. These plants are unable to survive in a highly saline environment. At question here is to what extent do water of varying salinities mix, and what is the resulting effect on the clam population in the sand delta. The Soft-Shell Clam and its Environment
The clam lives in the sand, often penetrating as deep as 30 cm, although usually somewhat less, and feeds by reaching its siphon to the sand surface. Cilia within the siphon create an incurrent flow of water, which carries with it food and oxygen. More cilia pick out food particles and pass them to the mouth and then to the stomach. Wastes are removed by the excurrent water. Spawn is also carried out in this manner and fertilization is external. The larvae remain free-swimming for up to two weeks before settling to the bottom. For several days the small clam will hold to the bottom with a thread-like byssus before finally burrowing into the sand. Like many other marine organ-
EFFECT OF SALINITY CHANGES 23
isms, the clam population survives by producing tremendous numbers of offspring. The mortality rate is quite high for the free-swimming larvae, and numbers are further reduced as many spat are likely to settle on hostile terrain. A wide variety of conditions may regulate the growth of clams. First, the substrate must be hard enough for the clam to dig without having its siphon smothered by mud or loose sand. The soft muddy bottom so characteristic of the great majority of the salt marsh is unsuitable for the clam, as the siphon could not be kept clear of the shifting mud.
Most significant of the influencing factors is the current. Current is important as the clam is stationary and it must depend on water movement to deliver food, oxygen, lime salts for shell formation, etc., as well as remove spawn, waste, and dangerous decaying material. Experiments have shown that there is an optimal current flow at which maximum growth of clams will take place. At lower flow rates they grow less well and in very rapid currents their survival rate rapidly diminishes (Belding 1914; Kellogg 1905). An additional factor influencing clam growth is salinity although there is conflicting evidence concerning its effect on their feeding activity. Matthiessen (1960) found growth rate to vary directly with salinity, while Blake (1961) reported no significant influence of salinity on feeding activity (in both cases feeding activity and rate of growth were considered directly related). My investigations at the Quaise marsh are an attempt to resolve this uncertainty. II Methods
Six sites for salinity measurements were chosen that would cover the delta and give representative sampling. Readings were taken using an induction salinometer (G.M. mfg. and Instrument Corp. RS5-3), which measured conductivity, salinity, and temperature, salinity being directly proportional to the salinity of water to conduct an electrical current. Stakes with crossbars at intervals of approximately 15 cm were placed at each of the six locations and the sensing unit was lashed at the end of a long fiberglass pole. The sensor was hung from the appropriate crossbar, depending on water level, and measurements were made without disturbing the water.
To monitor salinity fluctuations, readings were made at four phases of the tidal cycle: slack high and low tides, and mid-flooding and mid-ebbing tides. Salinity was checked at the surface and on the bottom at all stations, except at low tide. In this case, the water was so shallow at five of the six stations only one measurement could be made. Measurements were compiled over a period of seven days, and the range of the tides varied during that time (the seven sample days covered approximately half of the lunar tide cycle from spring tide to neap tide). The time of high and low water varied with the range of the tides.
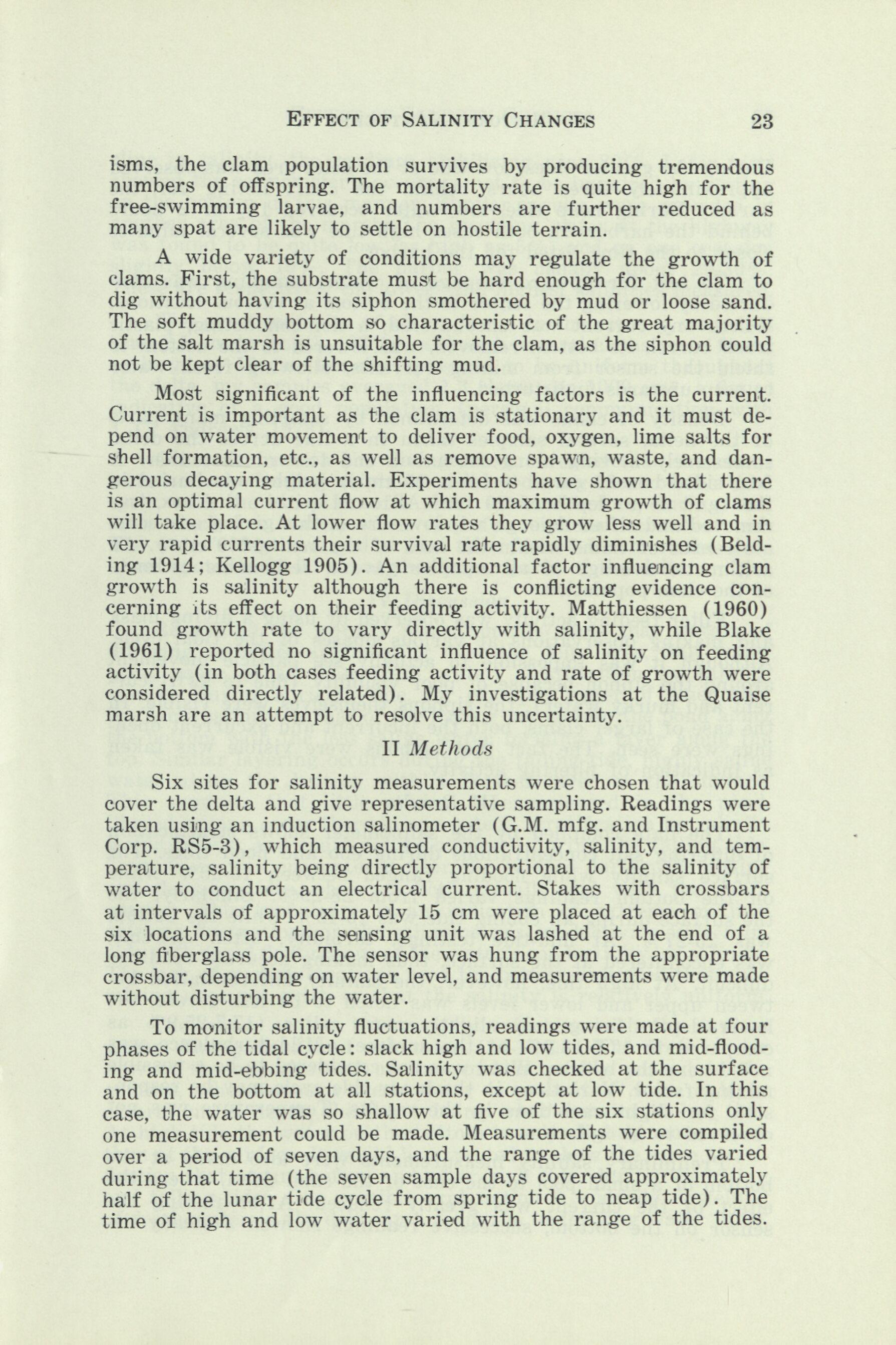
24
HISTORIC NANTUCKET
During the greater tides, low tide at the delta area was observed approximately three hours behind the chart listing for the harbor. During neap tide, low water occurred l1/? or 2 hours behind the harbor. In both cases high tide was several minutes closer to the time listed for the harbor.
To test for upward seepage of water, sampling was conducted by lowering the sensor to the bottom at various locations. Where any current was encountered, a non-conducting, plastic trash barrel, its bottom removed, was lowered to the bottom to shield the sensor from mixing currents, allowing fresh water to seep upwards undisturbed.
Density of clams was determined by sampling many parts of the delta, several samples taken in a line across the delta, successively moving along from one end toward the other. A four-sided box 25 cm x 40 cm x 25 cm in height was buried in the sand to a depth of 20 cm. All sand within the box was removed and sifted through window screen, this giving an accurate census of clams within the area of the box (1000 cm2). An arithmetic average of all samples gave a reasonable estimate of the clam population.
Filter feeding activity was observed by using a diving mask and making observations at various phases of the tidal cycle. Although it was impossible to actually see particle movement around the siphon because of poor visibility (due to swift current and murky water), the siphons themselves were visible. In the case of larger clams, both the incurrent and excurrent openings were seen. The fact that siphons were visible was taken as indication of filtering activity. Ill Results
The data compiled by the salinometer shows stratification of differing salt concentrations during ebb and flood tides. The surface salinity while flooding ranged between 16-20% while on the bottom readings varied from 28-29%. Similarly, the the surface during an ebbing tide was 16-19% while the bottom was 25-27%. At mid-tide testings, turbidity, often extreme, was encountered at the surface, thus making accurate readings difficult. At high tide, however, the salinity differentiation between upper and lower levels was noted to be less severe, the lower depths being only slightly more saline (17.7-25.9% as compared to 17.5-18.3% at the surface). Mild turbulance was noted near the bottom. Wind was not considered to be a possible mixing factor. Variations in the lunar tide cycle may be responsible for slight variations in the results. Temperature was not observed to be a factor.
Preliminary salinity measurements were also made at the bottom of a small pool to the east of the sand delta, where the effect of the current was greatly reduced. After resting the sensor on the bottom, successive readings showed a steady de-
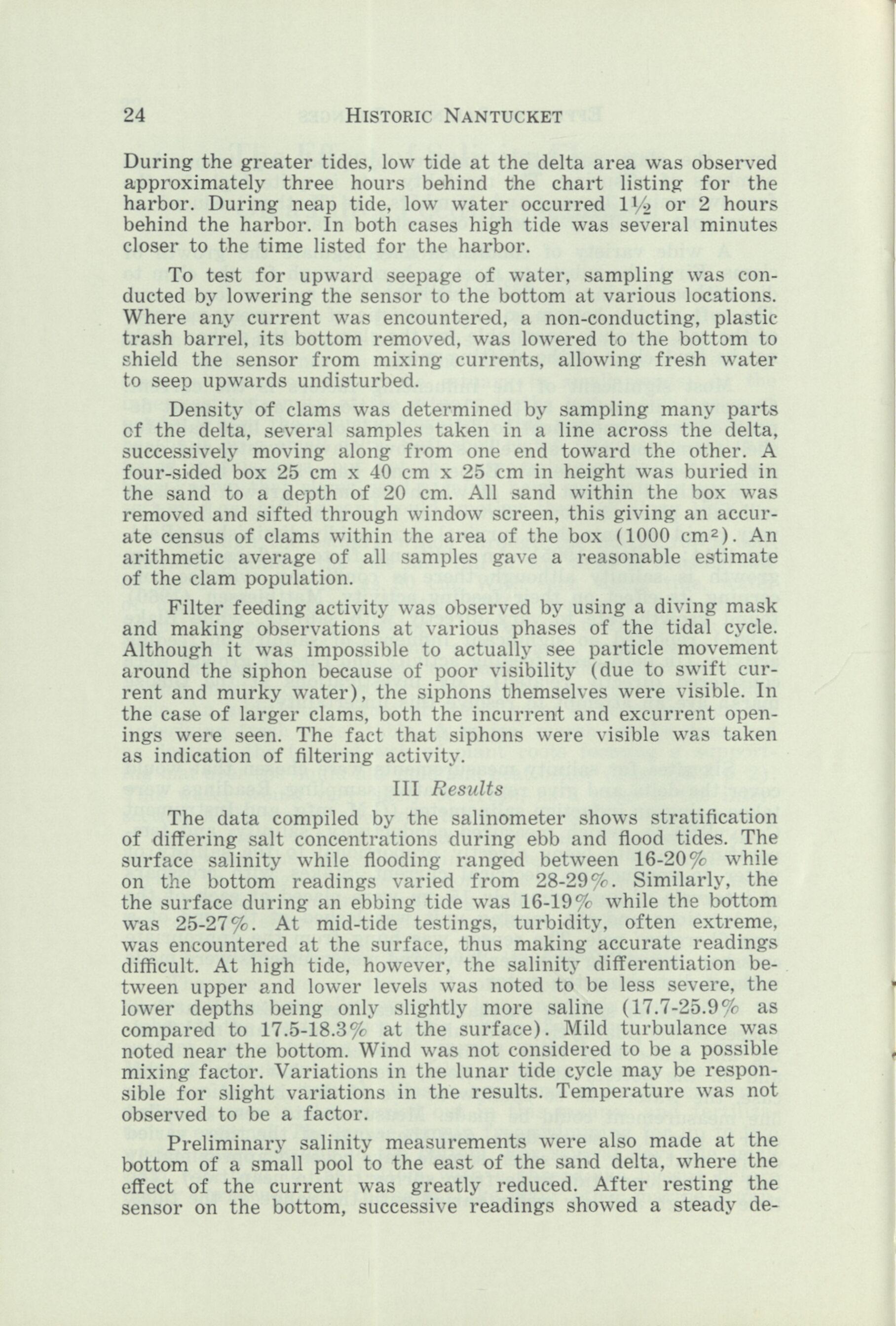
EFFECT OF SALINITY CHANGES 25
cline in salinity, the readings stabilizing near 80%. Later tests showed the salinometer's accuracy not to be impaired by resting on the bottom in this manner. Similar results were recorded in another area of the marsh. The incoming water at the highway was found to be quite low, as high as 5%, and on one occasion slightly below 10%. The harbor was usually about 29-30%. These are respectively the lowest and highest salinities measured.
The great majority of clams found in the estuary were less than 2.5 cm in length (83%), although several clams were found as large as 10 cm. Density appeared to be uniform throughout the delta, my samples averaged 1.3 clams per 1000 cm2 , a figure indicative of a good population (personal communication with J. C. Andrews, 1973).
Observation with the diving mask revealed that the clams were actively feeding (siphons visible) during most of the tidal cycle, with the exception of a short interval before, during, and after slack low water, when very few siphons were observed. The water's depth ranged at that time from 5-10 cm at the shallower spots, to 25-30 cm at the deeper parts of the delta. IV Discussion
Results of the salinity measurements show that waters of differing salinities are meeting over the delta area. Salt water being more dense than fresh water, stratification by salinity results. During a rising tide, for example, water of essentially the same salinity as that of the harbor moves into the pond along the bottom with the less saline water forming a layer along the top. Similar layering occurs during the falling tide, highly saline water along the bottom with a fresher layer at the surface. This suggests that little mixing is experienced in the pond. Should mixing occur there to any extent, stratification by salinity would be lost and could not be observed during the falling tide. Turbulence was encountered near the surface during the ebbing and flooding tides, observed both visually and with the salinometer. This turbulence is an indication of extreme mixing; extreme fluctuations on the salinometer's conductivity scale coincide with rapid fluctuations of the water's conductivity, or salinity. The lack of such fluctuations near the bottom indicated that such mixing does not exist, or that the salinity is relatively uniform.
The water at low tide roughly coincides with the surface water during flood and ebb tides (14-18%). The fresh water flow at the opposite end of the pond is quickly pushed to the surface, where enough fresh water is present to form a layer several cm in depth, this layer being what remains at low tide. At high tide the water on the bottom is only slightly more saline than at the surface. It would be expected to show the same stratification that occurs before and after high tide, during the rising and falling tides. One possible explanation is a steady upwelling of fresh water in the delta area from the underlying water table,
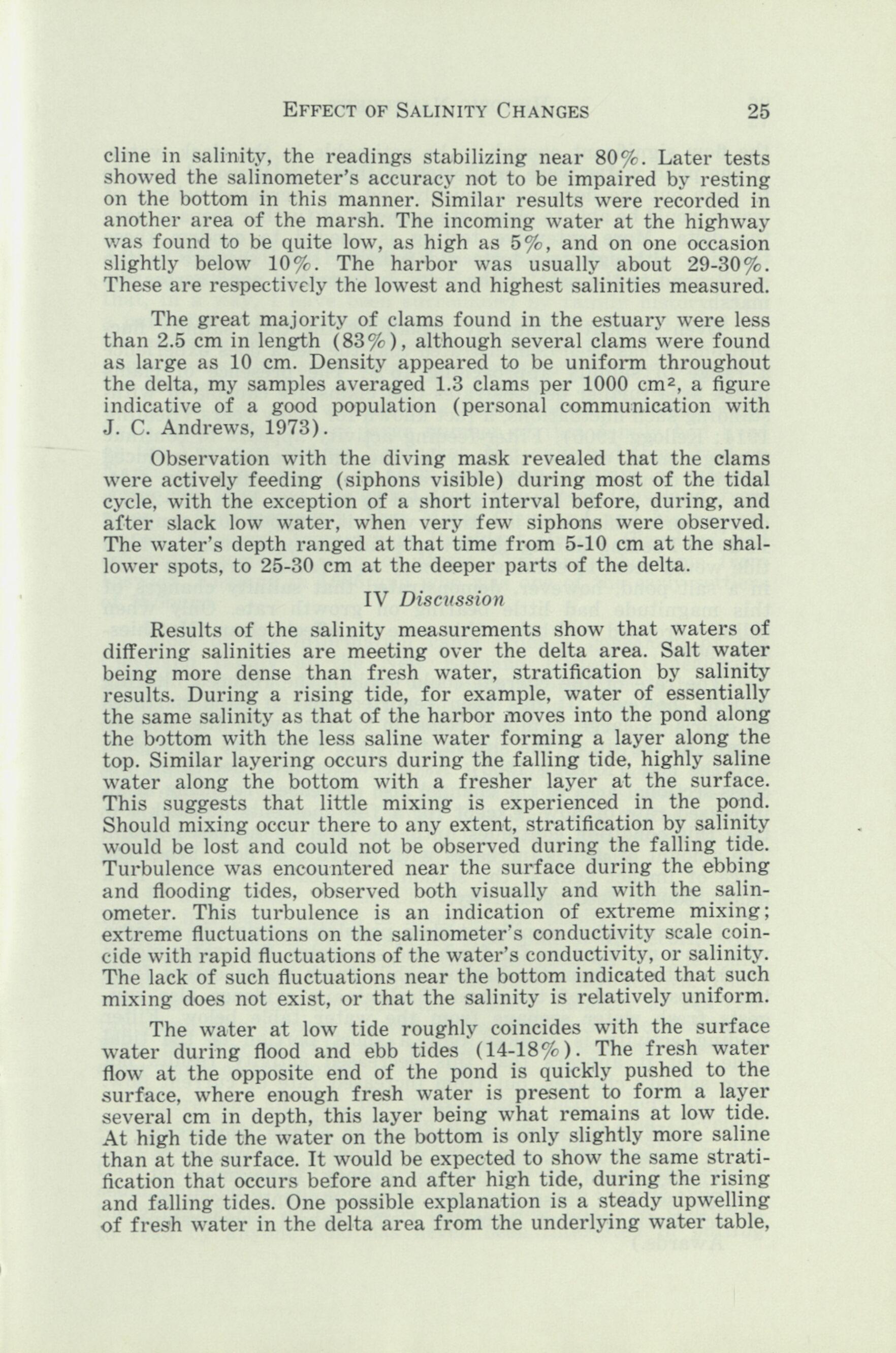
26 HISTORIC NANTUCKET
the flow significant enough to be noticeable in the slack water. If the pond water were unaffected in this way, it would be able to flush out the delta area quickly and the increased volume of water would be able to obliterate evidence of further seepage near the delta. More extensive testing is needed to confirm these theories, although two tests did indicate some seepage in the small pond to the east of the delta and another pond across the marsh.
Even without adequately explaining the tidal salinity fluctuations, it has been demonstrated that the delta does experience a range of salinity approaching 15%. The presence of clams in the area further demonstrates that clams are capable of withstanding such fluctuations, a fact known for many years (Belding 1914; Kellogg 1905). Filter-feeding activity appears to continue through most of the tidal cycle, the only period of reduced activity occurring shortly before, during and after low tide. The salinity on the bottom appeared to be quite high (25-29%) except during high and low tides, when it dropped to a range of 14-20%. Feeding during the lower salinities experienced at high tide would seem to eliminate salinity as a factor. Previous work in a salt pond, however, demonstrated that salinity changes of this magnitude had little bearing on growth rate. Only when salinity dropped well below this were the effects clear (Matthiessen 1960).
The rate of the current could explain the reduced filtering activity however. It has been reported that if sand particles or debris in large numbers come in contact with the sensory organs at the tip of the siphon, the clam withdraws the siphon into the hole (Kellogg 1905). As the water level decreases, the cross-sectional area of the channel also decreases, resulting in an increased rate of flow. Except for a few moments at slack low tide, this condition continues until the level is increased again. The amount of debris increases with the rate of flow, hence^ the force of impact against the siphon is increased, resulting in a much reduced feeding activity.
In summary, a significant clam population exists in an area which is a mixing zone for fresh and sea water. Sea water moves up the estuary from the harbor, while fresh water enters from the opposite end of the pond. Preliminary evidence also suggests fresh water seepage from the water table below. Filter feeding activity is reduced only a short portion of the tidal cycle, but it appears that this decreased activity results not from salinity changes, but from increased rate of current flow.
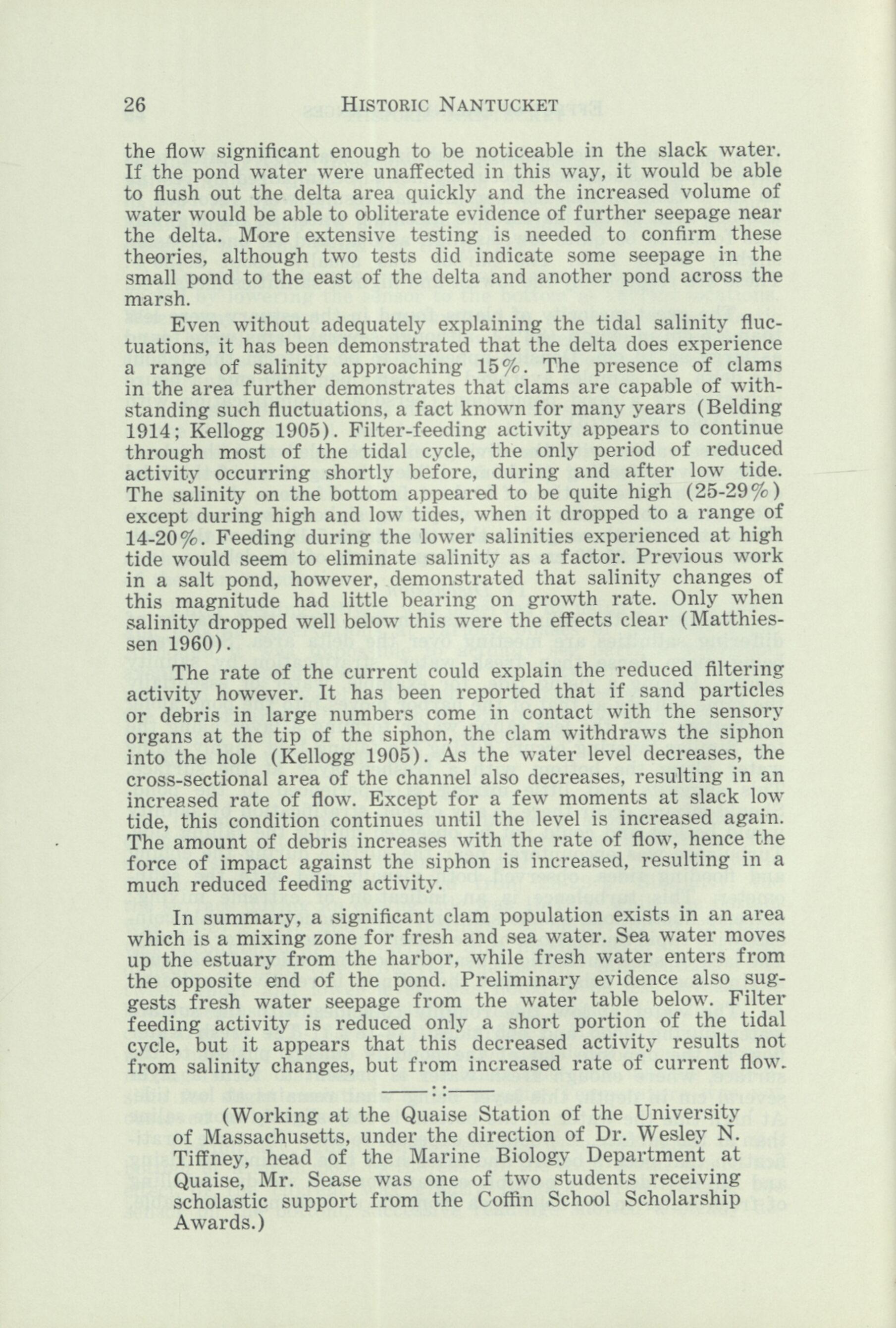
(Working at the Quaise Station of the University of Massachusetts, under the direction of Dr. Wesley N. Tiffney, head of the Marine Biology Department at Quaise, Mr. Sease was one of two students receiving scholastic support from the Coffin School Scholarship Awards.)