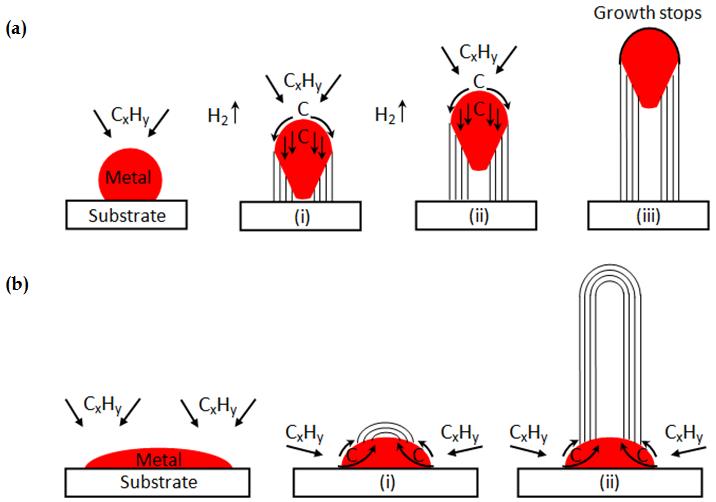
4 minute read
Carbon Nanotubes and how they grow
In chemistry lessons, most of us have learnt about structures formed by carbon that have different properties (allotropes), from diamond (one of the hardest materials in existence) to graphite (which can conduct electricity due to its carbon atoms only bonding with 3 other atoms, leaving mobile electrons that can carry charge). Carbon nanotubes (CNTs) are one of the structures carbon can take that we
do not learn much about, and so this article hopes to explore the properties and applications of carbon nanotubes as well as the process in which they are produced.
Advertisement
CNTs are extremely thin carbon structures and were first discovered by Iijima in 1993, and have similar properties to graphene in terms of electroconductivity and thermoconductivity, but are regarded as stronger - ‘the stiffest and strongest man-made material known to date’ ( Sattar, R., Kausar, A., & Siddiq, M. 2015) - than graphene due to their structure, easily explained using the analogy of how ‘a rolled piece of paper is stronger than the same paper as a flat sheet’ (Slomski. 2010). According to research conducted by the ACS, CNTs are ‘at least 117 times stronger than steel and 30 times stronger than Kevlar, the material used in bulletproof vests’ per unit of material (American Chemical Society.2010.). There may be some confusion caused as CNTs are referred to as ‘one-dimensional conductors’, but this just means that they are ‘materials whose electrical conductivity is high only along one direction’ (Kagoshima, S., Nagasawa, H.& Sambongi, T. 1988). This, in turn, proposes many possible applications of CNTs in our daily lives, including: Electron emitters (i.e. x-ray machines) (Baughman R.H. 2010) as CNTs are electron emitters with low chemical reactivity; microchips, as CNTs can act as semi-conductors and can cope with high temperatures as they can act as a ‘heat sink’; batteries, which can extremely lightweight and flexible, as well as highly-performing through a method of applying CNT ink onto paper (Hu, L. Et al. 2009); solar cells, whereby extremely thin CNTs are deposited onto a bacterial cellulose membrane template to create an electrically conductive and optically transparent layer (Jung, R. et al. 2008) that can replace transparent conducting films, etc.
As with most carbon-based structures, CNTs have high thermal conductivity and electrical conductivity, like metals, but achieve this via the vibration of covalent bonds between the carbon atoms, enhanced by the ‘stiffness’ of the carbon bond, as described in Nanotechnology for Dummies (Boyson, Muir et al. 2011, p. 76). However, not all CNTs are the same, and their individual properties depend on the type of CNT they are. CNTs are categorized into 2 main categories: single-wall carbon nanotubes (SWCNTs) and multi-walled carbon nanotubes (MWCNTs). SWCNTs are much smaller in diameter (typically ~1nm) (Faktri, N. et al. 2009) and also more curved compared to MWCNTs (typically ~50-150nm) (Nagai, H. Et al. 2011), which is rather self-explanatory as they are ‘single-
walled’. This means that they are more flexible and elastic, making them suitable in reinforcement of other materials such as plastics. As well as this, SWCNTs are useful for ‘exploiting the electronic properties which vary with their chirality’ (Jindal, V. 2012). Chirality indicates the structure of the CNT through the way by which the structure is ‘twisted’, and can be described as ‘chiral’, ‘zigzag’ (which can make the CNT a semi-conductor), or ‘armchair’ (which makes the CNT ‘metallic’ highly conducting). On the other hand, MWCNTs have, along with similar properties as SWCNTs, a higher tensile strength as it was found that ‘tensile strength of composite is proportional to the volume fraction of high modulus and low modulus fibres’ (Muzammil, S.B. et al. 2019). Other studies include the categories: double-walled carbon nanotubes (DWCNTs) and functional used carbon nanotubes (f-CNTs) (Mehra N.K. and Palakurthi S. 2016 ), which have ‘an open-end structure on both sides’ (Grumezescu, A.M. 2018).
Figure 1 (Kumar, M. 2010) a) tipgrowth model b) base-growth model
To be able to incorporate the properties of CNTs, the most important thing to do is to understand how to create them efficiently. There are 3 commonly used methods of synthesizing (i.e. growing) CNTs: the Arc Discharge Method, the Laser Ablation Method, and Chemical Vapor Deposition (CVD). This article will look specifically into the CVD method, which is currently the most popular method of ‘growing’ CNTs as it has high yields, can produce at relatively low temperatures and can ‘control size by varying the size of catalyst particles’ (Purohit, R. Et al. 2014). Unlike Arc Discharge and Laser Ablation, which are based on heating graphite/graphene to produce ‘tangled nanotubes mixed randomly with byproducts’ (Dai,H. 2002, Pg.2), the CVD method involves heating a catalytic transition-metal nano particle species supported on (typically) alumina in a patterned array and feeding a hydrocarbon gas through a tube reactor at between 700-900C, whereby the hydrocarbon gas decomposes into hydrogen and carbon (which are then deposited for CNTs to grow). According to the Ames Research Centre (Delzeit, L.D. 2004), the conditions of the formation of SWCNTs and MWCNTs differ, with SWCNTs preferring CH4 (which maintain thermal stability at night temperatures) at 900C and MWCNTs preferring C2H2/C2H4 at 750C. This demonstrates that SWCNTs have a higher energy of formation, which originates from their ‘characteristics of small diameter, large curvature, and high strain energy (Wang, X., Vinodgopal, K., Dai, G., 2019). The size of the CNTs depends on the size of the catalytic particle, which is shown in Figure 1.
The Vapour-Liquid-Solid (VLS) method by which the CNTs grow in CVD - as ‘traditionally it is widely accepted that the growth of SWCNTs from metal catalysts follows the VLS mechanism’ (Liu, C. and Cheng, H.