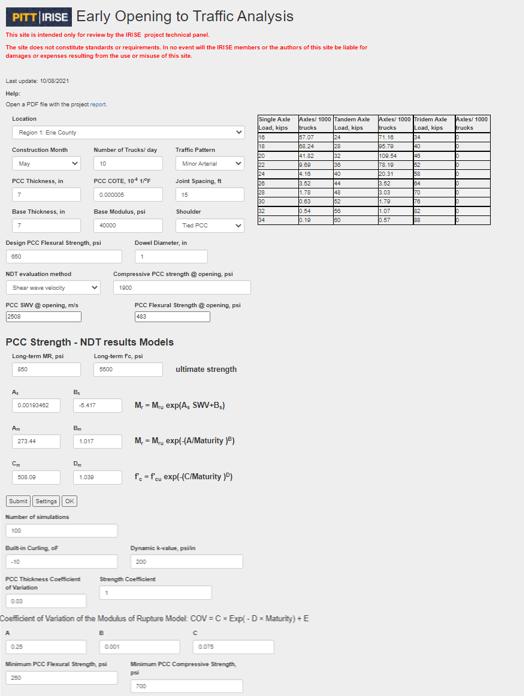
37 minute read
Figure 35: Total User Cost of Delay for Downtown Pittsburgh
[CATEGORY NAME], 2,674.7, [PERCENTAGE ]
[CATEGORY NAME], 4,688.4, Total User Cost of Delay for Arterial Network [PERCENTAGE ]
[CATEGORY NAME], 116,281.9, [PERCENTAGE ]
Figure 35: Total User Cost of Delay for Downtown Pittsburgh
In the arterial analysis, a few scenarios with different volumes show that a work-zone street closure in Pittsburgh Downtown area can cause user delay costs in the range of $102,779 to $123,645. The difference in models' delays, with and without work-zone, shows that such a street-closure work zone can increase the network's total delay by around 11%. It is essential to mention that the user delay costs incurred during 1.5 days of closure would be much lower if the extra half of the day is time overnight when traffic volumes are their lowest point.
This demonstrates the importance of short construction time in urban areas. A significant amount of user delay cost can be saved by cutting down the length of time a roadway must be closed for construction. Even a few hours saved can lead to tens of thousands of user cost saved for the project. Early opening is especially crucial to urban areas because of the effect on neighboring streets. The passenger cars that typically take the roadway under construction are forced onto other routes creating significant delays, as shown in the 11% network delay from the example. Departments of Transportations and drivers can see the benefits of reducing the road closure time to significantly reduce user costs and congestion. This allows concrete construction in an urban environment while limiting the closure time but retaining the long-term performance concrete pavements are known for.
However, this study presents several limitations. First, traffic volumes were available only for PM peak hours. Thus, estimation of volumes for other hours was done based on adjustment factors. Second, traffic in the VISSIM model routed through a set of static routes, and the rerouting (due to the work-zone street closure) needed to be done manually. Such an approach reduced the stochastic nature of the traffic and could have a minor-to-moderate impact on the model’s outputs. In the future, the model should use dynamic traffic assignment (DTA). Finally, various elements of modeled network should be calibrated and validated.
8 Conclusions
Early opening is a simple concept to reduce construction time with minimal cost to the project. The idea is to open a pavement to traffic as soon as the concrete strength can carry the expected traffic load without risking long term performance. It is critical that the strength of concrete be higher than the stress imposed by the expected traffic load. Traditionally, strength criteria are determined through destructive testing such as compressive or flexural strength. Departments of Transportation use either or both destructive tests to determine criteria for opening, however, the criteria differ between states with no clear guideline. Nondestructive methods are also being accepted in the industry to provide immediate results along with other benefits. Maturity and ultrasonic tomography are two nondestructive tests that are able to reliably estimate concrete strength and will be used in this project to improve early opening strength prediction.
The field study in this report evaluated the in-situ estimation of concrete strength of newly constructed concrete pavements using traditional maturity and emerging linear array ultrasound testing. Both methods estimate concrete strength indirectly and require calibration with laboratory measured strength development over time. Both methods permitted establishing a good correlation between the laboratory-measured strength properties (compressive or flexural strength) and fundamental PCC concrete properties: the heat of hydration for the maturity method and shear wave velocity for the ultrasound method. Both methods predicted reasonable concrete strength gain trends for in-situ pavement using two different mixtures: High-Early Strength and Long-Life Concrete pavement. Nevertheless, the linear array method was found to have the following advantages:
The same device can be used for taking measurements at the in-situ pavement and at concrete beams required to calibrate the method in the field; The shear wave velocity was found to correlate slightly better with concrete compressive and flexural strength for both mixes, maturity tended to provide a more conservative strength estimate; It does not require installation of sensors prior to pavement paving; The measurements can be taken practically at any point of the pavements system surface; It permits evaluation of variability of strength development in the in-situ pavement.
Shear wave velocities reported by the ultrasound tomography linear array system MIRA for beams and for slabs presented a systematic difference for the same maturity level. A possible explanation for this phenomenon is the influence of the concrete beam sides on the velocity calculation. A procedure for adjustment of beam velocity was proposed in this study, but a more rigorous procedure for computing shear wave velocity from the signals time histories for individual sensor pairs will improve accuracy and reliability of this technology.
Previous studies show that the current criteria for strength to open to traffic are conservative. Minnesota Department of Transportation were able to successfully load a concrete pavement after three hours with a flexural strength of 73 psi with no significant damage in the short- or long-term. The same study farther determined a mechanistic-based early opening damage analysis that is able to predict cracking and dowel bar performance reliability based on the maturity or strength at opening. This allows for proper risk assessment when determining when to open a pavement to traffic by accounting for rate of concrete strength gain, traffic volume, climate, load characteristics, and pavement structure parameters. This damage analysis model was expanded to apply to Pennsylvania conditions and to include the relation between maturity and shear wave velocity so that additional nondestructive testing can be used to predict strength gain. The addition of shear wave velocity allowed the pavement to be monitored at any location desired instead of being restricted to the temperature sensor locations for maturity. Critical or additional locations can be scanned quickly using ultrasonic tomography and still be used for strength predictions.
Concerning the possibility of opening pavements to traffic at earlier times, results from the traffic simulations indicate that for arterial roads, faster construction does have a significant impact on user delay costs. Since simulation involved a 24-hour road closure due to an active work zone, any reduction in closure time will yield substantial reduction in costs especially if the closure time is reduced during daytime. However, cases should be studied and modelled with a higher level of detail regarding the particular circumstances of each closure area and work zone.
References
―A1040 MIRA.‖ ACS, https://acs-international.com/product/a1040-mira/. Date Accessed Nov. 17, 2021.
An, J.-H., J. Nam, S.-H. Joh, and S.-A. Kwon, ―Estimation of the Compressive Strength of Concrete Using Shear Wave Velocity,‖ in New Technologies in Construction and Rehabilitation of Portland Cement Concrete Pavement and Bridge Deck Pavement, 2009, pp. 154–164.
ASTM, ASTM C39-Compressive Strength of Cylindrical Concrete Specimens, ASTM Stand., 2015. pp. 1–7.
ASTM, ASTM C78- Flexural Strength of Concrete (Using Simple Beam with Third-Point Loading), Annu. B. ASTM Stand., 2008. 4, no. Reapproved, pp. 1–7.
ASTM, ASTM 1074-Standard Practice for Estimating Concrete Strength by the Maturity Method 1, ASTM Stand. 2013, pp. 1–10
Bauer, Jocelyn, et al. ―Integrating Travel Time Reliability into Transportation System Management.‖ U.S. Department of Transportation, FHWA, 2019.
―Best Practices In Work Zone Assessment, Data Collection, And Performance Evaluation.‖ Lawrenceville, NJ : National Cooperative Highway Research Program, 2010. NCHRP Project 20-68A.
Carino, N. J. and H. S. Lew, “The Maturity Method: From Theory to Application,” Structures Congress & Exposition, 2001, p. 19.
Cho, M., S. Joh, S. A. Kwon, and T. Kang, ―Nondestructive In-Place Strength Profiling of Concrete Pavements by Resonance Search Technique, ‖ Proceedings of the 86th Annual Meeting of the Transportation Research Board, Washington D.C., 2007.
Choi, P., D.H. Kim, B.H. Lee, and M.C. Won, ―Application of Ultrasonic Shear-Wave Tomography to Identify Horizontal Crack or Delamination in Concrete Pavement and Bridge,‖ Constr. Build. Mater., 121, 2016, 81–91. https://doi.org/10.1016/j.conbuildmat.2016.05.126
Choubane, B. and Tia, M., ―Nonlinear temperature gradient effect on maximum warping stresses in rigid pavements.‖ Transportation Research Record, 1370, 1992, 11–19.
―Commonwealth of Pennsylvania Department of Transportation-Publication 408,‖ 2021.
Copeland, LuAnn. ―User's Manual for QUEWZ-98.‖ Texas Transportation Institute, 1998.
Crawford, G. I., ―Guide to Nondestructive Testing of Concrete,‖ FHWA-SA-97-105. 1997.
Crovetti, J. and L. Khazanovich, ―Early Opening of Portland Cement Concrete (PCC) Pavements to Traffic,‖ WHRP 05-13. Wisconsin Department of Transportation, Milwaukee, Wisconsin, 2005.
DeVries, Lyle, et al. ―Full Closure Strategic Analysis.‖ Colorado Department of Transportation, 2014.
Edara, Praveen K. and Cottrell, Benjamin H.,
―Estimation of Traffic Mobility Impacts at Work Zones: State of the Practice.‖ Transportation Research Board Annual Meeting, 2006.
Edara, Praveen. ―Evaluation of Work Zone Enhancement Software Programs.‖ Missouri Department of Transportation, 2009.
Edara, Praveen, et al. ―Understanding the Impacts of Work Zone Activities on Traffic Flow Characteristics.‖ Smart Work Zone Deployment Initiative, FHWA, 2018.
Elefteriadou, Lily, Jain, Mayank and Heaslip, Kevin. ―Impact of Lane Closures on Roadway Capacity Part B: Arterial Work Zone Capacity.‖ Florida Department of Transportation, 2008.
Freeseman, K., K. Hoegh, and L. Khazanovich, ―Concrete Strength Required to Open to Traffic,‖ MN/RC 2016-01. Saint Paul, MN, 2016.
―FREEVAL-PA: An Innovation in Transportation & Software Engineering.‖ Kittelson & Associates. [Online] 2020. https://www.kittelson.com/ideas/freeval-pa-an-innovation-intransportation-software-engineering/.
Hardy, Matthew and Wunderlich, Karl. ―Traffic Analysis Tools Volume IX: Work Zone Modeling and Simulation—A Guide for Analysts.‖ Federal Highway Administration Office of Operations, 2009.
Hardy, Matthew and Wunderlich, Karl. ―Work Zone Analysis—A Guide for Decision-Makers.‖ Federal Highway Administration Office of Operations, 2008.
Heaslip, Kevin, et al. ―Estimation of Freeway Work Zone Capacity Through Simulation and Field Data.‖ Transportation Research Record, 2009.
Helal, J., M. Sofi, and P. Mendis, ―Non-destructive testing of concrete: A review of methods,‖ Electron. J. Struct. Eng., vol. 14, no. 1, pp. 97–105, 2015.
Hiller J. and J. Roesler, ―Simplified nonlinear temperature curling analysis for jointed concrete pavements.‖ ASCE Journal of Transportation Engineering, 136(10), 2010, 654-663
Hoegh, K., L. Khazanovich, and H. T. Yu, ―Ultrasonic tomography for evaluation of concrete pavements,‖ Transp. Res. Rec., 2232, 2011, 85–94. https://doi.org/10.3141/2232-09
Hoegh, K., L. Khazanovich, and H.T. Yu, “Concrete pavement joint diagnostics with ultrasonic tomography,” Trans. Res. Rec., 2305, 54-61, 2012.
Hoegh, K., ―Ultrasonic Linear Array Evaluation of Concrete Pavements‖. Dissertation. University of Minnesota, 2013.
Ioannides, A. and L. Khazanovich, ―Nonlinear Temperature Effects in Multi-Layered Concrete Pavements.‖ ASCE Journal of Transportation Engineering, 124(2), 1998, 128–136.
Jacoby, Ken, et al. ―A Tool for Integrated Schedule-Traffic-Cost Analysis for Highway Projects.‖ United States Department of Transportation, FHWA. [Online] 2008. https://www.fhwa.dot.gov/construction/ca4prsbroc.cfm.
Jeannotte, Krista and Chandra, Andre. ―Developing and Implementing Transportation Management Plans for Work Zones.‖ United States Department of Transportation, Federal Highway Administration, 2005.
Jiang, Xiaomo and Adeli, Hojjat. ―Freeway Work Zone Traffic Delay and Cost Optimization Model,‖ Journal of Transportation Engineering, 2003.
Jiang, Yi. ―Estimation of Traffic Delays and Vehicle Queues at Freeway Work Zones,‖ 80th Annual Meeting of the Transportation Research Board. 2001.
Kan, Xingan David, Ramezani, Hani and Benekohal, Rahim F., ―Calibration of VISSIM for Freeway Work Zones with Time-Varying Capacity.‖ Transportation Research Board 93rd Annual Meeting, 2014.
Khanta, Pothu Raju. ―Evaluation of Traffic Simulation Models for Work Zones in the New England Area.‖ University of Massachusetts Amherst, 2008.
Khazanovich, L., ―Structural Analysis of Multi-Layered Concrete Pavement Systems.‖ Ph.D. Thesis, University of Illinois, Urbana, IL, 1994.
Khazanovich, L., O. I. Selezneva, H. T. Yu, and M. I. Darter. ―Development of Rapid Solutions for Prediction of Critical Continuously Reinforced Concrete Pavement Stresses.‖ In Transportation Research Record: Journal of the Transportation Research Board, No. 1778, TRB, National Research Council, Washington, D.C., 2001, pp. 64–72.
Khazanovich, L., K. Kosar, and H. Li, ―Evaluation of Long-Term Impacts of Early Opening of Concrete Pavements.‖ Minnesota Department of Transportation. NRRA202111. 2021
Khazanovich, L. and D. Tompkins, ―Incorporating Slab/Underlying Layer Interaction into the Concrete Pavement Analysis Procedures.‖ The National Academies Press, Washington, D.C., 2017.
Khazanovich, L., J. Vandenbossche, J. DeSantis, S. Sachs. ―Development of an Improved Design Procedure for Unbonded Concrete Overlays.‖ Report No. MN 2020-08. Minnesota Department of Transportation, 2020.
Khazanovich, L., K. Kosar, and H. Li, ―Evaluation of Long-Term Impacts of Early Opening of Concrete Pavements,‖ National Road Research Alliance, Minnesota Department of Transportation, 2021. Report Number: NRRA202111
Kite, Steve. Work Zone ITS- ―North Carolina’s Experience‖. North Carolina DOT, 2013.
Mechanistic–Empirical Pavement Design Guide, Interim Edition: A Manual of Practice. AASHTO, Washington, D.C., 2015.
Memmott, Jeffery L. and Dudek, Conrad L.,
―Queue and User Cost Evaluation of Work Zones.‖ Transportation Research Board, 1984.
Michigan Department of Transportation, ―Special Provision for Quality Control and Acceptance of Portland Cement Concrete,‖ Michigan Dep. Transp., vol. 604. August 2013.
Minnesota Department of Transportation, ―Estimating concrete strength by the maturity method.‖ MnDOT Specifications 2461.3.G.6, 2020. Concrete Maturity (state.mn.us)
Mudge, Richard, et al. ―Work Zone Performance Measurement Using Probe Data.‖ U.S. Department of Transportation, Federal Highway Administration, 2013.
NCHRP. ―Guide for mechanistic-empirical design of new and rehabilitated pavement structures.‖ Final report. Project 1-37A. National Cooperative Highway Research Program, Transportation Research Board, Washington, D.C., 2004.
NJDOT, New Jersey Department of Transportation Standard Specifications for Road and Bridge Construction, New Jersey Dep. Transp. 2015. pp. 1–37
―Office of Operations‖. FHWA Office of Operations. U.S. Department of Transportation, FHWA. [Online] April 2020. https://ops.fhwa.dot.gov/aboutus/opstory.htm.
―Regional Offices.‖ Pennsylvania Department of Transportation, [Online] 2021. https://www.penndot.gov/RegionalOffices/Pages/default.aspx.
Pennsylvania DOT. ―Publication 213- Temporary Traffic Control Guidelines.‖ Pennsylvania DOT, 2006-2007.
Pennsylvania Department of Transportation. ―Pennsylvania Work Zone Pocket Guide for Municipalities & Utilities.‖ Pennsylvania Department of Transportation, 2010.
PennDOT, PTC, and PSP. ―Pennsylvania's Automated Work Zone Speed Enforcement.‖ [Online] September 2020. https://workzonecameras.penndot.gov/locations/.
Salles, L., L. Khazanovich, L., and J. T. Balbo. Non-Destructive Evaluation of Crack Initiation and Propagation in Continuously Reinforced Concrete Pavements. Transportation Research Record, 2673 (2019), 375–385. https://doi.org/10.1177/0361198119833672
Sankar, Param, et al. ―Work Zone Impacts Assessment – An Approach to Assess and Manage Work Zone Safety and Mobility Impacts of Road Projects.‖ Washington, DC : Federal Highway Administration (FHWA), 2006.
Schnell, Thomas, Mohror, Jeffrey S. and Aktan, Fuat. ―Evaluation of Traffic Flow Analysis Tools Applied to Work Zones Based on Flow Data Collected in the Field.‖ Transportation Research Record, 2002.
Scriba, Tracy and Feast, Laura. ―Using Modeling and Simulation Tools for Work Zone Analysis. Work Zone and Traffic Analysis.‖ [Online] May 2009. https://ops.fhwa.dot.gov/wz/traffic_analysis/wza_leaflet/wza_leaflet.htm.
Song, Ziqi and Yin, Yafeng. ―Impact of Lane Closures on Roadway Capacity Part C: Modeling Diversion Propensity at Work Zones.‖ Florida Department of Transportation, 2008
State of Ohio Department of Transportation. ―Ohio Construction and Material Specifications,‖ Harrisburg, PA, 2019.
Tennessee Department of Transportation. ―Work Zone Safety and Mobility Manual.‖ Tennessee Department of Transportation, 2017.
Thomlinson, J.,
―Temperature Variations and Consequent Stresses Produced by Daily and Seasonal Temperature Cycles in Concrete Slabs.‖ Concrete and Construction Engineering, 36 (6), 1940, 298-307.
―Traffic Bottlenecks.‖ U.S. Department of Transportation, FHWA. [Online] March 2020. https://ops.fhwa.dot.gov/bn/bnchart.htm.
Trask, Lake, et al. ―FREEVAL-WZ Users Guide.‖ NC State University, 2016.
Trask, Lake and Aghdashi, Behzad. ―FREEVAL NC User Guide.‖ Kittelson & Associates, ITRE, 2019.
TxDOT, ―Standard Specifications for Construction and Maintenance of Highways, Streets, and Bridges,‖ Texas Dep. Transp., November 2014.
Ullman, Gerald L., Porter, Richard J. and Karkee, Ganesh J. ―Monitoring Work Zone Safety and Mobility Impact in Texas.‖ Texas Department of Transportation, 2009.
―Urban Street Reliability Engine User Guide.‖ Kittelson & Associates, Inc. 2014.
Vancura, M., L. Khazanovich, and R. Barnes, Concrete pavement thickness variation assessment with cores and nondestructive testing measurements, Transp. Res. Rec., 2347 (2013) 61–68. https://doi.org/10.3141/2347-07
Van Dam, T. J., Peterson, K. R., Sutter, L. L., Panguluri, A., Sytsma, J., Buch, N., Kowli, R., & Desaraju, R., ―Guidelines for Early-Opening-to-Traffic Portland Cement Concrete for Pavement Rehabilitation (NCHRP Report 540).‖ Washington, DC: National Highway Cooperative Research Program, Transportation Research Board, National Academies. 2005
Washburn, Scott S., Hiles, Thomas and Heaslip, Kevin. ―Impact of Lane Closures on Roadway Capacity,‖ Florida Department of Transportation, 2008.
Westergaard, H. M. ―Stresses in Concrete Pavements Computed by Theoretical Analysis. Public Roads,‖ Vol. 7, No. 2, 1926, pp. 25–35. Also appeared as Computation of Stresses in Concrete Roads. HRB Proc., 5th Annual Meeting, Part I, National Research Council, Washington, D.C., 1926, pp. 90–112.
―Work Zone and Traffic Analysis Tools.‖ U.S. Department of Transportation, FHWA. [Online] Work Zone Management Program, February 2019. https://ops.fhwa.dot.gov/wz/traffic_analysis/tools.htm.
Ye , Zhirui and Al-kaisy, Ahmed. ―Explore ITS Technologies for Work Zones and Work Zone Impact Areas.‖ Western Federal Lands Highway Division, 2010.
Yu, H.T., L. Khazanovich, M.I. Darter, and A. Ardani, ―Analysis of Concrete Pavement Responses to Temperature and Wheel Loads Measured from Instrumented Slabs.‖ Transportation Research Record, 1639, 1998, 94–101.
Zayed, A., ―Performance Improvement of High Early Strength (HES) Concrete for Pavement Replacement,‖ 2018.
Appendix A
Appendix A presents the average shear wave velocity (SWC) collected in slabs HES1 and C1.1 during the experiment.
Table A. 1: Shear Wave Velocity of slab C1.1 (Day 1)
Date Time Time from construction (h)
Section Position Avg. SWV (m/s) Cycle 7/13/20 15:27 5.13 C-1.1 TE 1445 1 7/13/20 15:28 5.14 C-1.1 TC 1475 1 7/13/20 15:30 5.17 C-1.1 CRS 1520 1 7/13/20 15:31 5.18 C-1.1 CRN 1505 1 7/13/20 15:31 5.19 C-1.1 CLS 1495 1 7/13/20 15:32 5.20 C-1.1 CLN 1520 1 7/13/20 15:45 5.42 C-1.1 TE 1565 2 7/13/20 15:46 5.43 C-1.1 TC 1590 2 7/13/20 15:47 5.45 C-1.1 CLS 1575 2 7/13/20 15:48 5.48 C-1.1 CRS 1610 2 7/13/20 15:49 5.48 C-1.1 CLN 1680 2 7/13/20 15:50 5.50 C-1.1 CRN 1735 2 7/13/20 15:59 5.66 C-1.1 TE 1650 3 7/13/20 16:00 5.68 C-1.1 TC 1685 3 7/13/20 16:01 5.68 C-1.1 CLS 1675 3 7/13/20 16:02 5.70 C-1.1 CRS 1680 3 7/13/20 16:03 5.72 C-1.1 CLN 1700 3 7/13/20 16:04 5.73 C-1.1 CRN 1740 3 7/13/20 16:15 5.92 C-1.1 TE 1745 4 7/13/20 16:15 5.92 C-1.1 TC 1790 4 7/13/20 16:17 5.95 C-1.1 CLS 1785 4 7/13/20 16:18 5.97 C-1.1 CRS 1780 4 7/13/20 16:19 5.98 C-1.1 CLN 1800 4 7/13/20 16:19 5.99 C-1.1 CRN 1830 4 7/13/20 16:44 6.40 C-1.1 TE 1925 5 7/13/20 16:44 6.41 C-1.1 TC 1940 5 7/13/20 16:45 6.42 C-1.1 CLS 1950 5 7/13/20 16:45 6.42 C-1.1 CRS 1965 5 7/13/20 16:47 6.46 C-1.1 CLN 1970 5 7/13/20 16:48 6.47 C-1.1 CRN 1975 5
Table A. 2: Shear Wave Velocity of slab C1.1 (Subsequent days)
Date Time Time from construction (h)
Section Position Avg. SWV (m/s) Cycle 7/14/20 8:32 22.21 C-1.1 TE 2520 6 7/14/20 8:35 22.25 C-1.1 TC 2500 6 7/14/20 8:36 22.28 C-1.1 CRS 2500 6 7/14/20 8:37 22.29 C-1.1 CLS 2530 6 7/14/20 8:38 22.31 C-1.1 CRN 2500 6 7/14/20 8:39 22.33 C-1.1 CLN 2530 6 7/14/20 10:50 24.50 C-1.1 TE 2530 7 7/14/20 10:51 24.52 C-1.1 TC 2530 7 7/14/20 10:52 24.53 C-1.1 CLS 2530 7 7/14/20 10:53 24.55 C-1.1 CLN 2530 7 7/14/20 10:53 24.56 C-1.1 CRN 2530 7 7/14/20 10:54 24.57 C-1.1 CRS 2530 7 7/15/20 10:37 48.29 C-1.1 TE 2560 8 7/15/20 10:38 48.30 C-1.1 TC 2605 8 7/15/20 10:39 48.32 C-1.1 CLS 2580 8 7/15/20 10:39 48.33 C-1.1 CLN 2580 8 7/15/20 10:40 48.33 C-1.1 CRN 2580 8 7/15/20 10:41 48.35 C-1.1 CRS 2605 8 7/16/20 12:26 74.11 C-1.1 TE 2600 9 7/16/20 12:27 74.13 C-1.1 TC 2530 9 7/16/20 12:28 74.14 C-1.1 CLS 2615 9 7/16/20 12:29 74.15 C-1.1 CLN 2610 9 7/16/20 12:30 74.17 C-1.1 CRN 2625 9 7/16/20 12:31 74.18 C-1.1 CRS 2610 9 7/18/20 8:06 117.77 C-1.1 TE 2645 10 7/18/20 8:06 117.77 C-1.1 TC 2660 10 7/18/20 8:07 117.78 C-1.1 CRN 2665 10 7/18/20 8:07 117.79 C-1.1 CRS 2650 10 7/18/20 8:08 117.80 C-1.1 CLS 2660 10 7/18/20 8:08 117.81 C-1.1 CNS 2660 10
Table A. 3: Shear Wave Velocity of slab C1.1 (Subsequent days) Part II
Date Time Time from construction Section Position Avg. SWV (m/s) Cycle
(h) 7/20/20 10:43 168.38 C-1.1 TE 2650 11 7/20/20 10:44 168.40 C-1.1 TC 2635 11 7/20/20 10:44 168.41 C-1.1 CRS 2655 11 7/20/20 10:45 168.43 C-1.1 CLS 2665 11 7/20/20 10:46 168.44 C-1.1 CRN 2685 11 7/20/20 10:47 168.45 C-1.1 CLN 2665 11 7/27/20 11:46 337.43 C-1.1 TE 2695 12 7/27/20 11:47 337.45 C-1.1 TC 2700 12 7/27/20 11:47 337.46 C-1.1 CRN 2715 12 7/27/20 11:48 337.48 C-1.1 CRS 2695 12 7/27/20 11:49 337.48 C-1.1 CLS 2710 12 7/27/20 11:49 337.49 C-1.1 CLN 2680 12
Table A. 4: Shear Wave Velocity of slab HES1 (Day 1)
Date Time Time from construction (h)
Section Position Avg. SWV (m/s) Cycle 7/13/20 10:22 2.78 HES-1.1 TE 3537 1 7/13/20 10:25 2.84 HES-1.1 TC 3930 1 7/13/20 10:29 2.91 HES-1.1 CRS 3387 1 7/13/20 10:30 2.92 HES-1.1 CLS 1997 1 7/13/20 10:32 2.95 HES-1.1 CLN 2453 1 7/13/20 10:34 2.98 HES-1.1 CRN 2657 1 7/13/20 10:37 3.03 HES-1.1 TE 3230 2 7/13/20 10:39 3.07 HES-1.1 TC 1010 2 7/13/20 10:41 3.11 HES-1.1 CRS 2980 2 7/13/20 10:42 3.13 HES-1.1 CLS 1010 2 7/13/20 10:44 3.16 HES-1.1 CLN 1010 2 7/13/20 10:45 3.18 HES-1.1 CRN 1010 2 7/13/20 10:51 3.28 HES-1.1 TE 1010 3 7/13/20 10:53 3.31 HES-1.1 TC 1050 3 7/13/20 10:54 3.33 HES-1.1 CRS 1010 3 7/13/20 10:56 3.35 HES-1.1 CLS 1153 3 7/13/20 10:58 3.38 HES-1.1 CLN 1190 3 7/13/20 10:59 3.41 HES-1.1 CRN 1083 3
Table A. 5: Shear Wave Velocity of slab HES1 (Day 1) Part II
Date Time Time from construction Section Position Avg. SWV (m/s) Cycle
(h) 7/13/20 11:05 3.51 HES-1.1 TE 1073 4 7/13/20 11:07 3.53 HES-1.1 TC 1187 4 7/13/20 11:08 3.56 HES-1.1 CRS 1047 4 7/13/20 11:10 3.58 HES-1.1 CLS 1243 4 7/13/20 11:11 3.61 HES-1.1 CLN 1267 4 7/13/20 11:12 3.62 HES-1.1 CRN 1220 4 7/13/20 11:20 3.76 HES-1.1 TE 1210 5 7/13/20 11:21 3.78 HES-1.1 TC 1300 5 7/13/20 11:23 3.80 HES-1.1 CRS 1203 5 7/13/20 11:24 3.82 HES-1.1 CLS 1387 5 7/13/20 11:25 3.84 HES-1.1 CLN 1410 5 7/13/20 11:27 3.87 HES-1.1 CRN 1337 5 7/13/20 12:00 4.42 HES-1.1 TE 1555 7 7/13/20 12:00 4.43 HES-1.1 TC 1610 7 7/13/20 12:01 4.43 HES-1.1 CRS 1530 7 7/13/20 12:02 4.45 HES-1.1 CLS 1670 7 7/13/20 12:03 4.47 HES-1.1 CLN 1675 7 7/13/20 12:03 4.48 HES-1.1 CRN 1655 7 7/13/20 12:34 4.99 HES-1.1 TE 1770 8 7/13/20 12:36 5.03 HES-1.1 TC 1835 8 7/13/20 12:37 5.04 HES-1.1 CRS 1755 8 7/13/20 12:38 5.06 HES-1.1 CLS 1830 8 7/13/20 12:39 5.08 HES-1.1 CLN 1950 8 7/13/20 12:40 5.09 HES-1.1 CRN 1920 8 7/13/20 13:00 5.42 HES-1.1 TE 1935 9 7/13/20 13:01 5.43 HES-1.1 TC 2010 9 7/13/20 13:02 5.45 HES-1.1 CRS 1925 9 7/13/20 13:03 5.47 HES-1.1 CLS 2055 9 7/13/20 13:04 5.49 HES-1.1 CLN 2015 9 7/13/20 13:05 5.51 HES-1.1 CRN 2030 9
Table A. 6: Shear Wave Velocity of slab HES1 (Day 1) Part III
Date Time Time from construction (h)
Section Position Avg. SWV (m/s) Cycle 7/13/20 13:26 5.86 HES-1.1 TE 2075 10 7/13/20 13:27 5.88 HES-1.1 TC 2095 10 7/13/20 13:28 5.89 HES-1.1 CRS 2060 10
7/13/20 13:29 5.91 HES-1.1 CLS 2100 10 7/13/20 13:30 5.93 HES-1.1 CLN 2100 10 7/13/20 13:31 5.93 HES-1.1 CRN 2125 10 7/13/20 14:00 6.42 HES-1.1 TE 2140 11 7/13/20 14:00 6.43 HES-1.1 TC 2135 11 7/13/20 14:01 6.44 HES-1.1 CRS 2110 11 7/13/20 14:02 6.45 HES-1.1 CLS 2135 11 7/13/20 14:03 6.47 HES-1.1 CLN 2130 11 7/13/20 14:04 6.48 HES-1.1 CRN 2160 11 7/13/20 14:22 6.78 HES-1.1 TE 2170 12 7/13/20 14:23 6.81 HES-1.1 TC 2155 12 7/13/20 14:24 6.83 HES-1.1 CRS 2115 12 7/13/20 14:25 6.83 HES-1.1 CLS 2150 12 7/13/20 14:26 6.85 HES-1.1 CLN 2140 12 7/13/20 14:27 6.87 HES-1.1 CRN 2160 12 7/13/20 15:21 7.77 HES-1.1 TE 2170 13 7/13/20 15:22 7.78 HES-1.1 TC 2195 13 7/13/20 15:23 7.80 HES-1.1 CLS 2160 13 7/13/20 15:23 7.81 HES-1.1 CRS 2135 13 7/13/20 15:24 7.83 HES-1.1 CLN 2150 13 7/13/20 15:25 7.83 HES-1.1 CRN 2240 13 7/13/20 16:21 8.78 HES-1.1 TE 2210 14 7/13/20 16:23 8.80 HES-1.1 TC 2175 14 7/13/20 16:24 8.82 HES-1.1 CLS 2210 14 7/13/20 16:25 8.83 HES-1.1 CRS 2170 14 7/13/20 16:25 8.83 HES-1.1 CLN 2170 14 7/13/20 16:26 8.86 HES-1.1 CRN 2250 14
Table A. 7: Shear Wave Velocity of slab HES1 (Subsequent Days)
Date Time Time from construction (h)
Section Position Avg. SWV (m/s) Cycle 7/14/20 8:16 24.69 HES-1.1 TE 2310 15 7/14/20 8:17 24.71 HES-1.1 TC 2300 15 7/14/20 8:19 24.73 HES-1.1 CRS 2310 15 7/14/20 8:20 24.75 HES-1.1 CRN 2320 15 7/14/20 8:21 24.77 HES-1.1 CLN 2310 15 7/14/20 8:22 24.79 HES-1.1 CLS 2305 15 7/14/20 10:45 27.17 HES-1.1 TE 2305 16
7/14/20 10:45 27.18 HES-1.1 TC 2310 16 7/14/20 10:46 27.19 HES-1.1 CRS 2285 16 7/14/20 10:47 27.20 HES-1.1 CLS 2300 16 7/14/20 10:48 27.22 HES-1.1 CLN 2310 16 7/14/20 10:49 27.23 HES-1.1 CRN 2310 16 7/15/20 10:32 50.96 HES-1.1 TE 2335 17 7/15/20 10:33 50.98 HES-1.1 TC 2310 17 7/15/20 10:34 50.98 HES-1.1 CLS 2340 17 7/15/20 10:35 51.00 HES-1.1 CRS 2310 17 7/15/20 10:36 51.02 HES-1.1 CRN 2345 17 7/15/20 10:36 51.03 HES-1.1 CLN 2330 17 7/16/20 13:04 77.49 HES-1.1 TE 2330 18 7/16/20 13:05 77.50 HES-1.1 TC 2300 18 7/16/20 13:06 77.53 HES-1.1 CLN 2360 18 7/16/20 13:07 77.54 HES-1.1 CRN 2310 18 7/16/20 13:08 77.56 HES-1.1 CRS 2270 18 7/16/20 13:09 77.58 HES-1.1 CLS 2310 18 7/18/20 7:49 120.23 HES-1.1 TE 2405 19 7/18/20 7:50 120.25 HES-1.1 TC 2310 19 7/18/20 7:51 120.27 HES-1.1 CRN 2405 19 7/18/20 7:52 120.28 HES-1.1 CRS 2395 19 7/18/20 7:53 120.30 HES-1.1 CLS 2400 19 7/18/20 7:54 120.32 HES-1.1 CLN 2400 19
Table A. 8: Shear Wave Velocity of slab HES1 (Subsequent Days) Part II
Date Time Time from construction (h)
Section Position Avg. SWV (m/s) Cycle 7/20/20 10:36 171.02 HES-1.1 TE 2310 20 7/20/20 10:37 171.04 HES-1.1 TC 2305 20 7/20/20 10:39 171.07 HES-1.1 CRS 2360 20 7/20/20 10:40 171.08 HES-1.1 CLS 2365 20 7/20/20 10:41 171.10 HES-1.1 CRN 2410 20 7/20/20 10:42 171.12 HES-1.1 CLN 2350 20 7/27/20 11:34 339.98 HES-1.1 TE 2405 21 7/27/20 11:35 340.00 HES-1.1 TC 2365 21 7/27/20 11:36 340.03 HES-1.1 CRN 2415 21 7/27/20 11:37 340.03 HES-1.1 CRS 2400 21 7/27/20 11:37 340.04 HES-1.1 CLS 2370 21
7/27/20 11:38 340.05 HES-1.1 CLN 2395 21
Appendix B
Appendix B presents the average shear wave velocity (SWV) collected in beams at the laboratory.
Table B. 1: Shear Wave Velocity for LLCP beams
Date Time Time from construction (h)
Section Position Avg. SWV (m/s) 7/14/20 10:23 23.89 D1B7 A 2617 7/14/20 10:25 23.92 D1B7 B 2590 7/14/20 10:27 23.96 D1B7 C 2620 7/14/20 10:29 23.99 D1B7 D 2617 7/14/20 10:31 24.02 D1B5 A 2670 7/14/20 10:33 24.05 D1B5 B 2587 7/14/20 10:34 24.07 D1B5 C 2660 7/14/20 10:35 24.09 D1B5 D 2553 7/14/20 10:37 24.12 D1B9 A 2670 7/14/20 10:38 24.14 D1B9 B 2617 7/14/20 10:40 24.17 D1B9 C 2647 7/14/20 10:41 24.19 D1B9 D 2613 7/16/20 11:04 72.57 D1B12 A 2727 7/16/20 11:06 72.61 D1B12 B 2730 7/16/20 11:08 72.63 D1B12 C 2730 7/16/20 11:09 72.65 D1B12 D 2730 7/16/20 11:14 72.74 D1B11 A 2730 7/16/20 11:17 72.79 D1B11 B 2670 7/16/20 11:20 72.84 D1B11 C 2730 7/16/20 11:24 72.90 D1B11 D 2730 7/16/20 11:28 72.97 D1B13 A 2730 7/16/20 11:31 73.02 D1B13 B 2670 7/16/20 11:33 73.06 D1B13 C 2730 7/16/20 11:36 73.10 D1B13 D 2730
Table B. 2: Shear Wave Velocity for LLCP beams Part II
Date Time Time from construction Section Position Avg. SWV (m/s)
(h) 7/18/20 7:20 116.84 D1B15 A 2730 7/18/20 7:21 116.86 D1B15 B 2720 7/18/20 7:23 116.88 D1B15 C 2733 7/18/20 7:24 116.90 D1B15 D 2660 7/18/20 7:26 116.94 D1B4 A 2730 7/18/20 7:28 116.98 D1B4 B 2730 7/18/20 7:30 117.01 D1B4 C 2773 7/18/20 7:32 117.03 D1B4 D 2730 7/18/20 7:36 117.10 D1B8 A 2733 7/18/20 7:38 117.14 D1B8 B 2673 7/18/20 7:40 117.17 D1B8 C 2730 7/18/20 7:42 117.20 D1B8 D 2703 7/20/20 10:13 167.72 D1B18 A 2747 7/20/20 10:14 167.73 D1B18 B 2737 7/20/20 10:16 167.77 D1B18 C 2833 7/20/20 10:17 167.79 D1B18 D 2747 7/20/20 10:20 167.84 D1B14 A 2733 7/20/20 10:22 167.87 D1B14 B 2720 7/20/20 10:24 167.91 D1B14 C 2747 7/20/20 10:26 167.94 D1B14 D 2730 7/20/20 10:28 167.98 D1B10 A 2870 7/20/20 10:30 168.01 D1B10 B 2737 7/20/20 10:32 168.03 D1B10 C 2857 7/20/20 10:33 168.06 D1B10 D 2740 7/27/20 10:51 336.35 D1B6 A 2770 7/27/20 10:52 336.37 D1B6 B 2730 7/27/20 10:53 336.38 D1B6 C 2730 7/27/20 10:54 336.40 D1B6 D 2693 7/27/20 10:59 336.49 D1B17 A 2880 7/27/20 11:01 336.52 D1B17 B 2740 7/27/20 11:02 336.53 D1B17 C 2883 7/27/20 11:03 336.55 D1B17 D 2760 7/27/20 11:11 336.68 D1B16 A 2890 7/27/20 11:12 336.71 D1B16 B 2737 7/27/20 11:13 336.72 D1B16 C 2890 7/27/20 11:14 336.74 D1B16 D 2870
Table B. 3: Shear Wave Velocity for HES beams
Date Time Time from construction Section Position Avg. SWV (m/s)
(h) 7/13/20 14:43 7.14 D2B3 D 2253 7/13/20 14:45 7.17 D2B3 A 2280 7/13/20 14:47 7.20 D2B3 B 2253 7/13/20 14:48 7.23 D2B3 C 2323 7/13/20 14:50 7.26 D2B1 A 2200 7/13/20 14:53 7.30 D2B1 B 2227 7/13/20 14:54 7.32 D2B1 C 2250 7/13/20 14:56 7.35 D2B1 D 2170 7/13/20 14:59 7.41 D2B4 A 2267 7/13/20 15:00 7.43 D2B4 B 2307 7/13/20 15:02 7.46 D2B4 C 2307 7/13/20 15:04 7.49 D2B4 D 2260 7/13/20 15:08 7.56 D2B2 A 2243 7/13/20 15:09 7.57 D2B2 B 2237 7/13/20 15:11 7.60 D2B2 C 2270 7/13/20 15:12 7.62 D2B2 D 2210
Appendix C
Appendix C provides a user manual to use the Early Opening to Traffic Analysis Webbased Tool created in this study. Figure C. 1 shows the default screen that appears when the user first accesses the website and highlights the different areas to be discussed in this user manual.
User Inputs
Traffic Spectrum
Parameters at Time of Opening
Nondestructive Models
Advanced Settings
Figure C. 1: Web-based tool opening screen
User Inputs
To begin, the user must choose the location of the pavement project from a drop-down menu. Location is broken into five districts based on similar climates. The options for pavement location are as follows:
Region 1: Erie County Region 2: PennDOT Districts D1, D10, D11, and D12 (except for Erie) Region 3: PennDOT Districts D2 and D9 Region 4: PennDOT Districts D3 and D4 Region 5: PennDOT Districts D5, D6, and D8
Use Error! Reference source not found. for reference if district is unknown.
Next, the time of construction by month must be chosen from the drop-down menu.
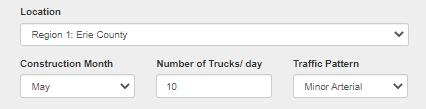
Figure C. 2: Construction and traffic parameters
Then the number of trucks per day must be entered by the user and a traffic pattern must be chosen from the drop-down menu. Choosing a traffic pattern will fill the traffic spectrum shown in Figure C. 3. Options for traffic patterns that automatically create a traffic spectrum are Minor Arterial, Residential, and Interstate. There is also a custom option if the exact traffic spectrum is known. The user can then input the custom traffic directly into the table.
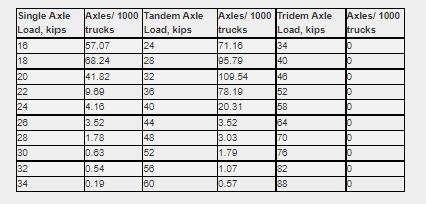
Figure C. 3: Provided traffic spectrum for minor arterial
Next, the user must input the PCC and base thickness in inches. Additional parameters include the PCC coefficient of thermal expansion and base modulus. The default options are typical values but for increased accuracy, it is recommended the user input the project values. Joint spacing must be entered in feet. The shoulder type must be chosen from the drop-down menu as a tied shoulder or asphalt/no shoulder. Dowel diameter in inches must be entered.
The design PCC flexural strength is the final input required in the user input section of the website. This is the required flexural strength for the project.
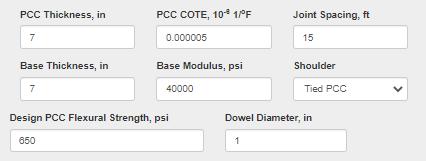
Figure C. 4: Pavement structure inputs
Parameters at Traffic Opening
This section of the website is about the pavement at the time of opening. The nondestructive test being used must be chosen from the drop-down menu between shear wave velocity and maturity. Depending on the nondestructive test chosen, the value below will change to represent the method.
The user then can enter a value for compressive PCC strength at opening. This can be a guess at first and will be reiterated as improved estimations are found. The minimum value is dictated by an advanced setting to be discussed later. The web tool will automatically change the PCC shear wave velocity or maturity and PCC flexural strength at opening.
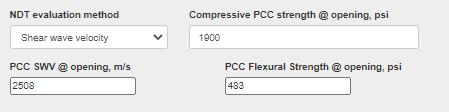
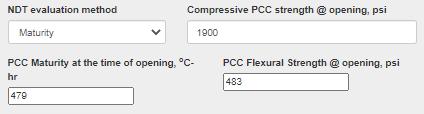
Figure C. 5: User inputs at time of opening for shear wave velocity or maturity.
Nondestructive Models
In this section of the tool the user has the option of modifying the model that relate the nondestructive tests to strength.
First the user must enter the ultimate flexural and compressive strength of the concrete mixture.
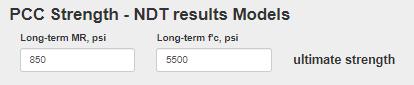
Figure C. 6: Ultimate strength inputs
Here the user can change the model coefficients. If shear wave velocity is the nondestructive test chosen, there will be an additional model to relate shear wave velocity to flexural strength. The remaining models are the same to create the strength predictions.
The user can change these coefficients from their typical default values to the values the user calculated based on their own project. These can be found using the procedure outlined in this project.
(a)
(b)
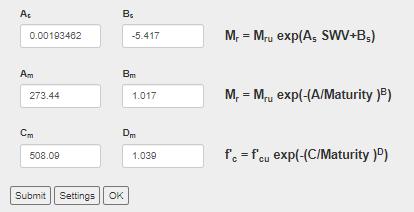
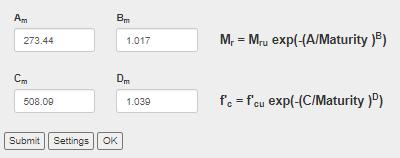
Figure C. 7: Location to change the nondestructive testing model coefficients for (a) shear wave velocity and (b) maturity.
Advanced Settings
The advanced settings can be opened be clicking the Settings button at the bottom of the screen. This opens the following options:
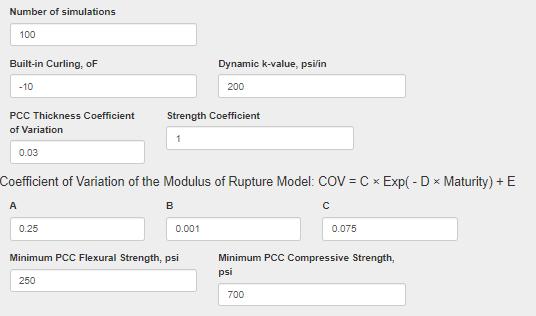
Figure C. 8: Advanced settings
These settings are not required to use the web tool but will increase the accuracy of the results. Increasing the number of simulations can increase the accuracy of the model but will also take longer. The built in curling and dynamic k-value can be changed but a standard value is the default. The expected PCC thickness variation can also be changed depending on past work in the area, but the standard default value is 0.03. The strength coefficient can also be changed here from the standard value of 1. The coefficient of variation in the modulus of rupture coefficients can also be modified for increased accuracy.
The key part of the advanced settings is the minimum strength values. It is highly recommended to enter these values for each project because the minimum opening strength suggestion will not go lower than this value. This is the lowest age that strength testing was done in the lab. The analysis will not go lower than this value so that it does not extrapolate a strength value that was not tested.
Click OK to exit the advanced settings.
Reading the Results
When the entered values best represent the project, click submit. The results will start with numerical values for the cracking performance reliability, dowel performance reliability, and ESALs repetitions to design strength at the opening strength chosen in the inputs (Figure C. 5).
Figure C. 9: Numerical results
If the reliabilities are not sufficient for the user, the simulation can be rerun using the graphed results to determine the next opening strength guess. In this example, the initial value for cracking performance is only about 89% which can be improved. Using the reliability graph created in the results, the user can find a strength with a higher reliability. In this example, using a flexural strength of 460 psi will provide a 97% cracking performance reliability.
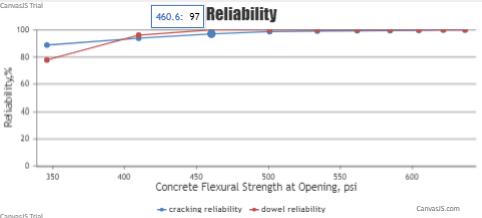
Figure C. 10: Reliability graphs
The user can then change the compressive PCC strength at opening to the updated value and rerun the simulation. It is not recommended to use the values from the result graphs without rerunning the simulation for that opening strength.
Once the reliability is acceptable for the user, that strength is the recommended value to open to traffic for that project.
Appendix D
In this appendix, based on the reviewed documents, webinars, and other resources, a brief summary is provided of what methods and tools are other states around the nation utilizing for analyzing the impacts of work zones. This information could be helpful in terms of decision making for the appropriate work zone software with various configurations.
Table E. 1: State DOT Survey Results
States Tools and Methods
California
Delaware
FREQ, HCS, CORSIM, and PASSER Spreadsheets are used for capacity addition projects on all roads except urban arterials and urban freeways. PASSER program is used for urban arterial roadways with signalized intersections, diamond interchanges, etc. High impact urban freeway construction or rehabilitation projects with capacity reductions: FREQ, HCS, or CORSIM. QuickZone and CA4PRS QuickZone as the main tool to estimate traffic impacts of work zones. A simpler version of the tool called the 'Lane Closure Analysis Program (LCAP)' is also used for simple work zone. configuration Work zones on arterials or interstates that do not involve any signalized intersections are analyzed using QuickZone or LCAP.
For two-lane roadways with flagging operations, the CORSIM simulation program with sufficient parameter calibration is used. SYNCHRO
Florida Uses a customized spreadsheet program.
Illinois
Use of a customized excel spreadsheet with input capacity values determined by the QUEWZ program. The QUEWZ program has produced good estimates of queue lengths in the past. However, since the software is no longer maintained Ohio DOT is looking for alternatives for the future.
Iowa
Maryland
Minnesota
Missouri
New Hampshire
New York State
Ohio
Oregon
Pennsylvania
Texas
A customized spreadsheet was occasionally used. Based on past experience and knowledge of the staff. One of the districts also mentioned using SYNCHRO software. QuickZone SYNCHRO A customized excel spreadsheet that performs HCM analysis. HCM Quickzone Quickzone AASHTO User Benefit Analysis CORSIM VISSIM SYNCHRO MoDOT Spreadsheet Quickzone
Quickzone when it is close to the work zone lane capacity threshold
Sketch planning tools SYNCHRO WZTA Tool Highway Capacity Manual (HCM) SYNCHRO Microsimulation A Spreadsheet developed by a consultant. FREEVAL SYNCHRO Quickzone HCS
Wisconsin Work Zone Traffic Analysis Tool (WZTAT)
Appendix E
In this appendix, different types of work zone tools are described briefly.
QuickZone
QuickZone program is an analytical model, developed by FHWA, to facilitate the estimation of work zone traffic impacts. QuickZone provides a platform to compare traffic impacts for work zone mitigation strategies including estimating the costs, time delays, and potential backups associated with these impacts in addition to evaluating traffic delays linked with work zone schedules in relation to peak and off-peak traffic periods and/or with the employment of diversion routes. It can also display the amount of delay in vehicle hours and the maximum length of the projected traffic queue associated with the work activity. QuickZone is capable of analyzing full lane closures as well. QuickZone requires detour routes to be defined as part of the analysis network. Although QuickZone grants a comprehensive and detailed analysis of delays occurring in work zones, the process of analysis requires more time and effort in comparison with spreadsheet models (Edara et al., 2018).
QUEWZ
QUEWZ is an analysis tool for Queue and User-Cost Evaluation of Work Zones (QUEWZ) developed by the Texas Transportation Institute that can be used for estimating the traffic impacts of work zone lane closures. This tool can calculate the work zone capacity based on various input data such as hourly traffic volumes, percentage of trucks, capacity values under normal conditions, lane closure hours, work zone configuration, etc. It can also provide a traffic adjustment for demand values based on the alternate routes. The equations are based on HCM, 2000. QUEWZ is capable of analyzing traffic conditions on freeway segments with and without lane closures and providing estimates of additional road user costs and queuing as a result of work zone lane closures (Copeland, 1998).
CA4PRS
Construction Analysis for Pavement Rehabilitation Strategies (CA4PRS) is an analysis software platform that facilitates the integrated study of project alternatives for multiple solutions for pavement planning, logistics of construction, and traffic operations. CA4PRS was developed by the University of California, Berkeley, Institute of Transportation Studies, with technological assistance from the Turner-Fairbank Highway Research Center of FHWA. For alternate rehabilitation methods, including pavement cross-sections and material styles, construction windows and lane-closing techniques, and contractor logistics and restrictions, CA4PRS users may analyze "what if" scenarios. Moreover, CA4PRS can estimate the maximum distance of
highway that can be rehabilitated or reconstructed within various resource constraints and closure timeframes (Jacoby et al. 2008).
HCM-Based Tools
Highway Capacity Manual (HCM) could be utilized for assessing the capacity and quality of service for current conditions of highway facilities, as well as the effects of temporary conditions such as work zones and future conditions such as proposed improvement projects. HCM is able to address the correct application and implications of micro-simulation analysis; offers basic methods and generalized volume tables of service provides an interactive multimodal approach to urban street analysis; and explores how demand and capability can be influenced by successful traffic management. Highway Capacity Software (HCS) contains some modules for the implementation of animation and simulation of both freeways and intersections (FHWA, 2019).
FREEVAL
Freeway evaluation (FREEVAL) is a computerized method intended to diligently execute computations for undersaturated and oversaturated directional highway installations for organizational study. FREEVAL is a powerful tool based on the Highway Capability Manual for macroscopic freeway research (HCM).
FREEVAL-WZ
FREEVAL-WZ is a version of FREEVAL-2015e which is specifically designed to have a user-friendly environment that applies to work zone study planning level approach. The program aims to have the ability to easily test the results of various work zone conditions and to calculate the effects over time and space of congested times (Trask, 2016).
FREEVAL-NC
FREEVAL-NC is the unique variant of FREEVAL for North Carolina intended to have a user-friendly environment that applies an approach to the planning stage for the work zone and reliability review of the freeway. Additionally, FREEVAL-NC has access to the segmentation index of the entire North Carolina highways. The program aims to have the opportunity to rapidly test the results of multiple work zone conditions and to measure the effects of congested cycles over time and space with the ability to expand the reliability study over the year (Trask et al., 2019).
FREEVAL-PA
FREEVAL-PA is a tool developed by Pennsylvania DOT that facilitates all forms of freeway research, helping PennDOT workers and contractors to easily gain meaningful
information on what traffic effects they will see on every limited-access highway facility in the state from various forms of construction and maintenance programs. The software tool, along with traffic volume and speed data, weather data, elevation data, and a computing engine operated by the HCM, is programmed with the roadway characteristics of each highway section in Pennsylvania to understand the effect of decision-making on capacity. The concept of this tool is from the FREEVAL which has been programmed in North Carolina, early 1990s (FREEVALPA, 2020).
STREETVAL
STREETVAL, a similar tech application, permits analysis such in FREEVAl for arterial corridors. In order to create a travel time distribution from which reliability metrics can be derived, STREETVAL utilizes a scenario generator that feeds the Urban Streets Highway Power Analysis methodology. The technique of reliability can be used to determine the following causes of unreliable travel time on urban road services, including traffic accidents, work zones, variations in demand, special events, inadequate base capacity, etc. The input data expected to be analyzed using the HCM technique for an urban street facility (―Urban Street Reliability Engine User Guide‖, 2014).
Spreadsheets
Based on the literature, most of the state DOTs nationwide are using spreadsheet-based tools to evaluate the impacts of work zones. Using the graphical procedure outlined in HCM, along with analytical equations, most of the tools estimate the output (delay and queue lengths). Inputs are usually containing vehicle demand for every time interval, number of open lanes, roadway capacity, percentage of trucks, etc.
CORSIM
CORSIM is a software package for microscopic traffic modeling for signal systems, highway systems, freeway systems, or the combination of all. It is originally developed by FHWA and has been used for around 30 years. CORSIM is capable of adapting to simulate traffic operations around a work zone on the arterials in urban areas, suburbs, and towns. It can also simulate the work zones through a prolonged incident blockage, the program assumes that drivers have no knowledge of the approaching blockage and there is no taper. CORSIM results are not sensitive to the work zone length or the differentiating between the effects of right or left lane closures. In addition, CORSIM is not able to consider some of the geometric elements such as lane width and shoulder width (Heaslip et al., 2009).
VISSIM
VISSIM as one of the most popular microsimulation tools could be used for freeway analysis especially conducting research for work zone study. VISSIM as one of the most popular microsimulation tools could be used for freeway analysis especially conducting research for work zone study. VISSIM requires calibration and validation processes before providing meaningful simulation results. With a systematic procedure for calibration of a VISSIM model capacity, speed, and queue length could be obtained for work zone analysis. One study regarding the calibration of VISSIM for freeway work zones has concluded that if field speed data is input for desired speed distribution, VISSIM does not reproduce the data for single-lane freeway work zones (Kan et al., 2014).