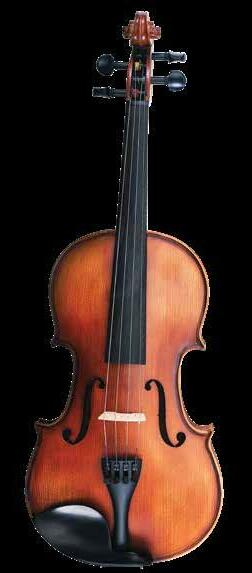
4 minute read
How does the violin saw its tooth?
Named in honour of Australia’s first Nobel Laureates William Henry Bragg and his son William Lawrence Bragg, the UNSW Press Bragg Prize for Science Writing recognises outstanding non-fiction writing on science.
High school students were invited to submit 800-word essays in response to the 2021 theme: The STEM in Everyday Life.
Stephanie Chew has been awarded as Runner-up the 2021 UNSW Bragg Student Prize for Science Writing. In her essay “How does the violin saw its tooth?” Stephanie explores the complicated science behind how a violin produces sound. We share Stephanie’s impressive essay below: It has been said by Isserlis, a British cellist, that Bach’s music shows us God’s view of the world, Mozart’s music is like a part of nature and Beethoven’s music speaks for all mankind. And the heart of it all is the amazing sound players release from their instruments. When I play Bach’s Sonata No. 2 on my violin, I’m whisked into a whirlpool of emotions, with excitement, sorrow and joy combining into one. The calming melody in the third movement flows over a steady baseline, and the sound from the violin soulfully cries out to the listener. I marvel at my violin’s ability to create polyphonic voices, voices that would otherwise take multiple singers to produce. Where does the violin’s mysterious ability to create sounds that strongly connect with human emotions come from? The answer lies in the combination of the player and the carefully calibrated design factors in the body of the violin that together create the sublime sound. The process starts when the bow is drawn across the strings. Friction causes the string to move. Helmholtz, a German physicist, showed that this is a ‘slip-stick’ motion that occurs hundreds of times per second. The string travels with the bow for some time (the stick), then slips in the other direction, before sticking to the bow again. The resulting wave looks like the teeth on a saw, so it’s called the ‘sawtooth wave’! The vibrational energy from the strings is then transferred to the body of the violin and the vibrations are transformed into sound. This is where the magic occurs. The body acts like a loudspeaker cone, with the greatest influence coming from its top and back plates. In the twelfth century, Fibonacci, an Italian mathematician, described the Fibonacci series. By taking one number in the series and dividing it by the two preceding numbers, we get a ratio of approximately 1.6. This is called the ‘Golden Ratio’. In the sixteenth and seventeenth centuries, master luthiers, such as Amati and Stradivarius, applied the Golden Ratio to perfect the geometrical proportions and the form of the violin. As deviations from these proportions can reduce the acoustical properties of the violin, they have continued relatively unchanged to this day. The masters further perfected the shape, length and location of the two sound holes on the violin’s top plate. These help the violin to optimally produce sound. Although we call these holes the ‘f-holes’, they are the long and descending ‘s’ used in Latin and Italian! How do these f-holes work? Well, they act as air-filled cavities that have a neck. This is called a ‘Helmholtz resonator’. Here, the volume of air in and near the open holes vibrates because of the springiness of the air inside. When the body vibrates, the volume of air inside also vibrates. This produces pressure fluctuations in the air which eventually leaves through the f-holes. If the f-holes are placed too far apart, the sound becomes rough. If placed too close together, the plate cannot vibrate properly, and the sound becomes ‘pinched’. If the f-hole is too wide, then the violin lacks the ability to project sound. Makris, a professor at MIT, showed that the f-hole length used by the masters increased the air-resonance power from their predecessors by approximately 60 per cent! The top and back plates of the violin’s body perfectly encapsulate the physics behind sound production. The masters established the ideal thickness and arching of these plates for optimal vibration and sound production. By tapping on the plate and listening to the resulting sound, they were able to tune the plates and scrape them to the desired thickness for easy vibration. This practice survives today. Thanks to research in 1787 by Chladni, a German physicist and musician, modern luthiers can better understand how these plates resonate by using Chladni patterns to visualise the vibrations on these plates. Symmetrical plates produce symmetrical Chladni patterns. These patterns help modern luthiers adjust the symmetry of the plate to optimise sound production and propagation. Finally, resonance features heavily in sound production in a violin. This sound is comprised of its fundamental frequency (measured in Hertz) and associated harmonics (i.e., multiples of the fundamental frequency). When the open A string (with a fundamental frequency of 440Hz) is played on the violin, it also produces sound at the harmonics (i.e., 880Hz, 1320Hz etc.). The E string (with a fundamental frequency of 660Hz) also vibrates, as 1320Hz is a common multiple. This is called resonance. Generally, the more intense and balanced the combination of fundamental frequencies and harmonics, the better the sound. Understanding these scientific fundamentals helps us appreciate the complicated process behind the way a violin produces sound. For the modern luthiers, this helps them replicate old master violins and to create acoustically better and more powerful violins. Stephanie Chew, Year 8