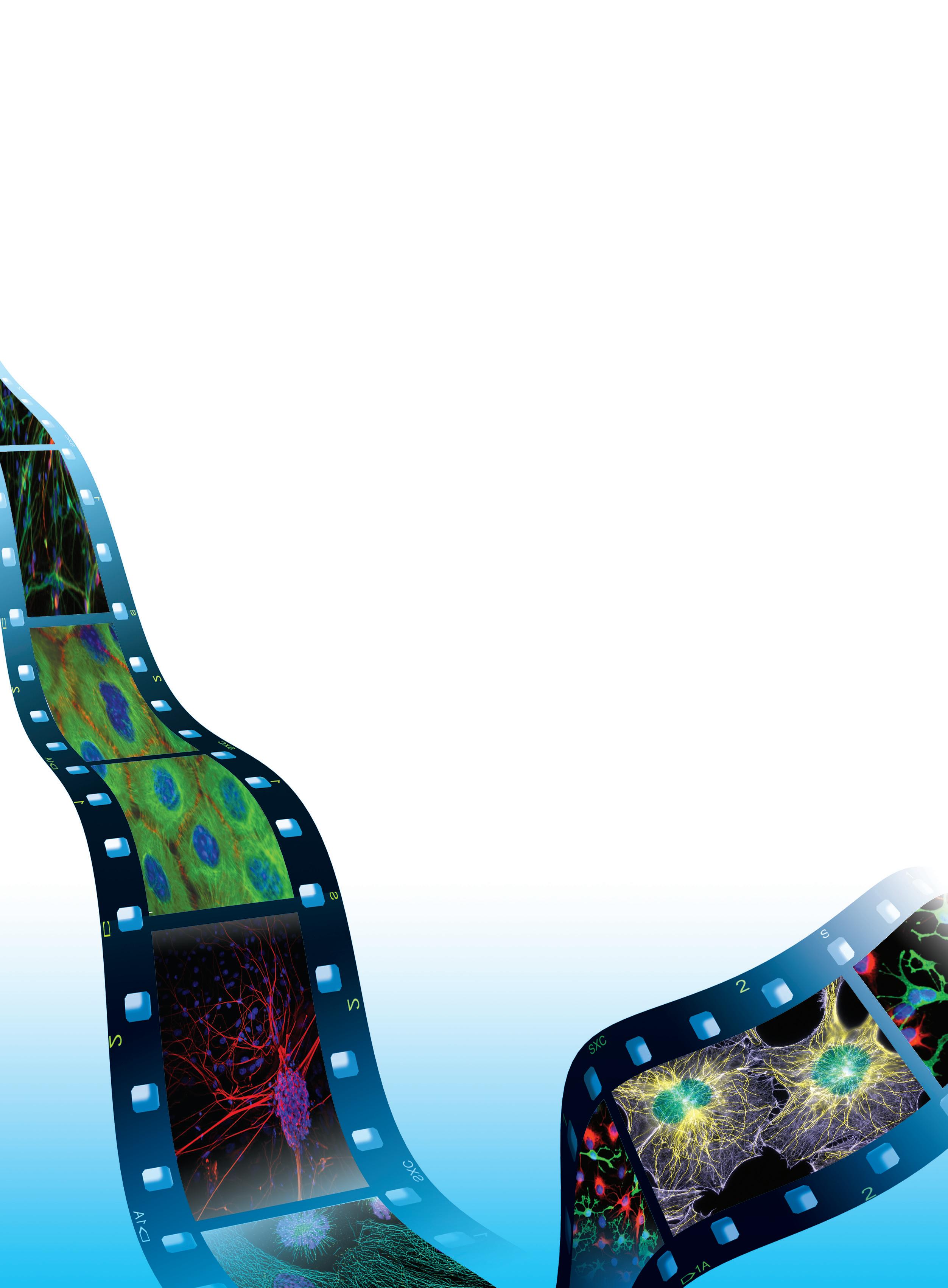
8 minute read
FLUORESCENCE IMAGING
AND AUTOMATED MICROSCOPY FOR CANCER MANAGEMENT IN THE DEVELOPED AND DEVELOPING WORLD
Cancer is a common, tragic and expensive disease. While more than 1/3 of the population of North American will be diagnosed with cancer at some time in their lives, and the cost of cancer in the US exceeded $219 billion in 20081, the situation is even worse in the developing world where even less resources are available and the human costs of cancer can be even higher.
Advertisement
Most cancers arise from precancerous lesions, and effective detection and removal of precancerous tissue may be the best, most cost-effective means to improve cancer survival and reduce cancer incidence. Early detection of cancer dramatically improves survival; when detected late, treatment is less effective, has greater morbidity, and is more expensive. One of the greatest success stories in cancer management is based upon early cancer detection and is that of cervical cancer screening. Cervical cancer screening is based upon the visual analysis of cytological samples (cells removed by brushing or scrapping and deposited on a slide) to identify patients requiring observation of the cervix using a magnified view (colposcopy). Areas suspect for precancerous or cancerous lesions are biopsied and if pathologically confirmed treatment is applied. Done effectively on a population basis this can reduce cervical cancer incidence and mortality by a factor of 42. Any drug with these characteristics would be considered to be an almost unbelievable blockbuster. While such screening programs have been widely implemented in the developed world, they are not available in much of the developing world due to infrastructure and training costs of such programs. Thus while cervical cancer is not a large killer in the developed world in the developing world it is one of the most common forms of cancer in women and a leading cancer killer3 .
There have been many efforts to develop clinical systems for the automated or semi- automated interpretation of cervical cytology, while most were technically successful wide spread adoption of these technologies has not occurred in the developed world where they must compete with existing conventional screening laboratories. Rapid advances in affordable computational power over the last several decades and improved understanding of the performance requirements needed for an effective cervical cancer screening program have made it possible to construct automated cervical cytology devices which can be effective components of cervical cancer screening programs in the developing world. An example of this is the implementation of the automated quantitative cytology system initially developed in at the BCCA for the cervical cancer screening4. In partnership with a Chinese company this technology has been clinically implemented in economically successful private clinics and public sponsored clinics in China which have screened over 350,000 women to date. Further this approach is undergoing controlled studies to determine its feasibility as part of a publically funded program. In collaboration with colleagues at Rice University, MD Anderson Cancer Center and Drexel University we have also set up two of these automated quantitative cytology systems in Nigeria to assess their
utility in the developing world for cervical screening.
The vast majority of cancers (~85per cent) arise in the epithelium (tissue connected in one way or another to the world outside the body). Most of these tissues are accessible to investigation by optical technologies. Electromagnet radiation in the near visible and visible part of the spectrum (380nm to 700nm) is strongly modified (scattered, absorbed, reemitted) by biological tissue. These strong interactions limit the depth into tissue which light can penetrate, but conversely these strong interactions make light based systems sensitive to the subtle changes associated with the development of pre-cancers in epithelial tissue. This is both a challenge and opportunity for optical based disease detection and delineation technologies.
One optically based detection technology currently used clinically is in vivo autofluorescence imaging. Some of the molecules in tissue which absorb light can re-emit the absorbed energy as slightly less energetic light i.e. as fluorescence light. The key molecules involved in tissue fluorescence are part of cellular metabolism (such as FAD and NADH) and make up the stroma immediately underlying the epithelium (elastin and collagen). The growth of pre-cancers in the epithelium alters cellular metabolism and can cause tissue remodeling within the stroma, both effects which are detectable by tissue autofluorescence. The first FDA approved commercial application of tissue autofluorescence was for detection of pre-cancer and cancers in the lung. This light induced fluorescence endoscopy (LIFE) device5 developed at the BCCA was found to be more than six times more sensitive for the detection of pre-cancers at high risk of becoming cancer and preinvasive cancers than conventional white light endoscopy. Almost all endoscope manufactures now produce fluorescence enabled endoscopes. However the high cost of such systems has limited the technologies use in other tissue sites.
The cost of this technology has changed radically over the last two decades, while initially such systems were on the order of ~$150,000 (laser illumination and image intensifier based detection systems), technical progress has greatly reduced the cost. For example, direct fluorescence visualization devices using battery powered LEDs for illumination and the eye as the detector for the oral cavity can cost as little as ~$2500. Figure 1 shows a comparison of the size and complexity of this technology originally and currently for lung imaging and oral cavity imaging, along with
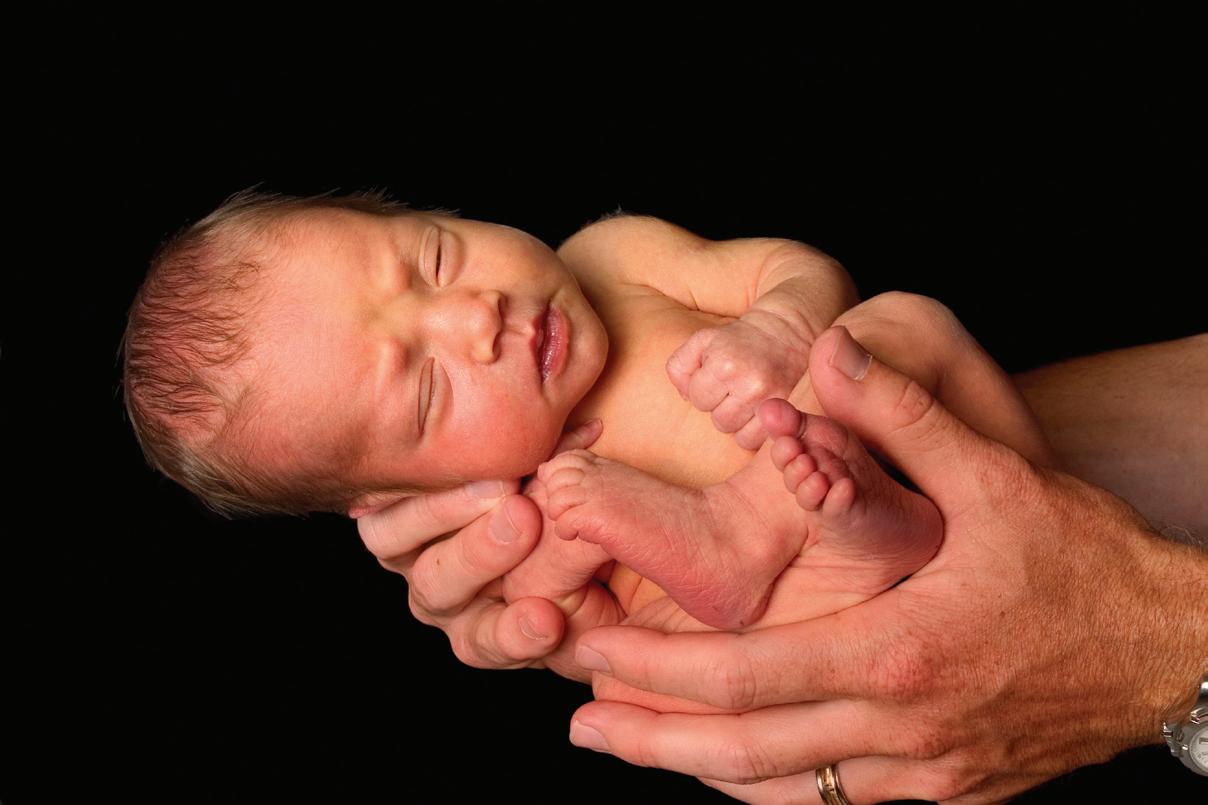
The Most Gentle Touch in Biotechnology
Like a newborn baby, biologically derived products require careful, precise and tender handling. Our centrifuges provide this treatment and more.
Hydrohermetic feed for gentle product treatment HydroStop for highest yields Hygenic design for cleaning-in-place (CIP) and sterilization-in-place (SIP) Scaled for 2 to 25,000 liter reactors GMP-compliant documentation
To learn more about GEA Westfalia Separator and how we can assist you with your separation needs in developing and manufacturing breakthrough biotech products, please contact Dan Van Damme at (905) 319-3900 ext. 216 or by email at: Dan.VanDamme@geagroup.com.
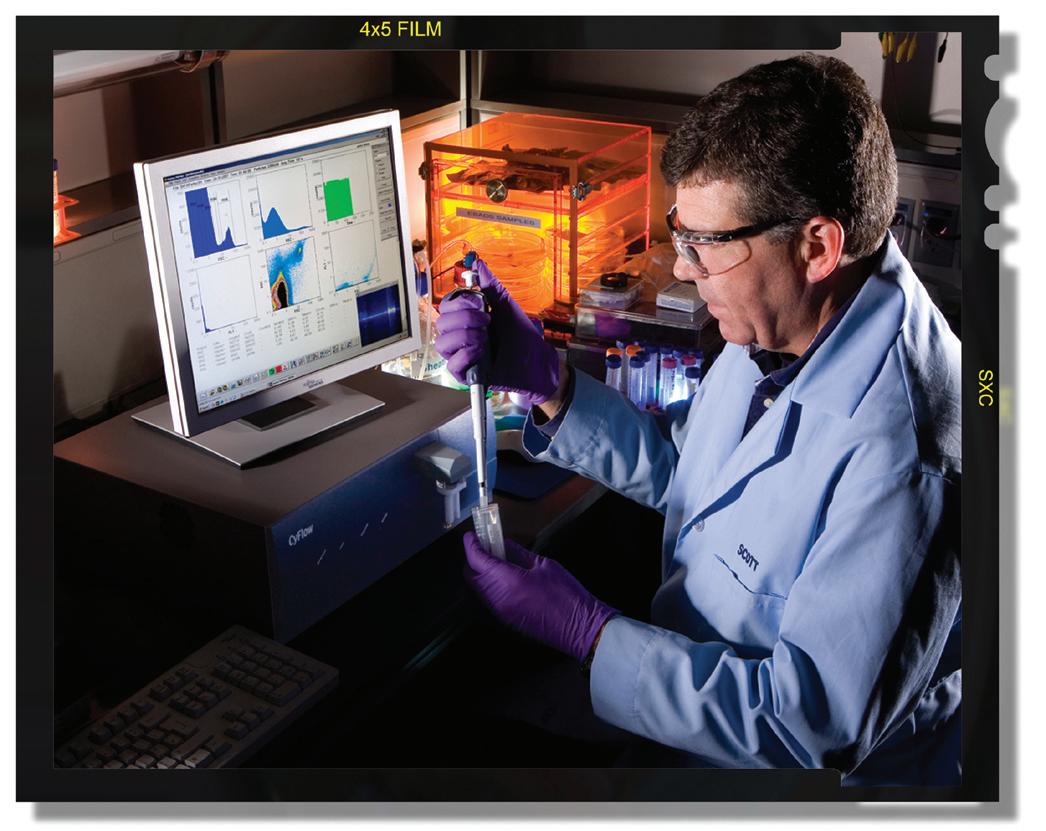
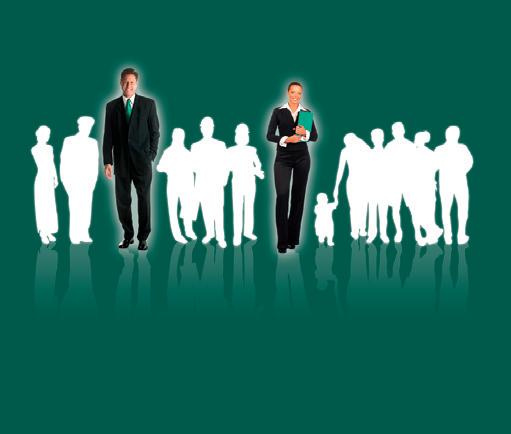
GEA Westfalia Separator Canada, Inc.
LIFe
Lung: Severe Dysplasia
onco LIFe
Lung: CIS
VeLscope
Oral: Cancer
Identafi 3000
Oral: Severe Dysplasia
Figure 1. Pictures of various clinical fluorescence systems used to image the lung and the oral cavity and example images beneath. The far left system is the original LIFE system developed in the late 80s, consisting of a 442nm laser, intensified camera system and computer console and video recorder and printer. The reddish brown areas in the lower autofluorescence images indicate the areas of abnormality, the green areas indicate normal tissue except in the right most fluorescence image in which normal tissue is blue. The near left system is the current Onco LIFE system which uses a filtered arc lamp as its light source and a small sensitive CCD camera, note the large reduction in system size. The reddish brown area in the lower image is the area of carcinoma in situ (CIS). The near right system is the VELscope system used for direct fluorescence visualization of the oral cavity, the illumination is a filtered arc lamp and the detector is the observer’s eye looking through the ocular of the headpiece (replaced in this example by a digital camera to acquire a picture). The reddish area is an example of a cancer not visible under white light imaging. The far right system is the Identafi 3000 system used for direct fluorescence visualization of the oral cavity. Battery powered LEDs are used for illumination and the observers eyes viewing through a coloured visor are the detectors. The reddish area is an example of a severe dysplasia.
examples of the types of images the various devices produce.
The latest commercially available fluorescence visualization (FV) devices for use in the oral cavity have shown the ability to not only enable the detection of clinically occult lesions but have demonstrated improved determination of surgical fields6 which has changed clinical practice in BC. Pilot data from the use of FV to establish the surgical field suggests this approach can greatly reduce local recurrence rates for surgically resected oral cancer (from 25 to 30 per cent to below two per cent)7 .
The cost of current in vivo fluorescence imaging systems is sufficiently low and the systems have very little infrastructure requirements being LED or flash based with digital point and shoot cameras, all battery powered, that they could be used in the developing world. In fact such versions are currently be evaluated for oral cancer and cervical cancer management in the developing world (India, Nigeria) by several research groups.
At a time when the global incidence and mortality of cancer is rapidly increasing8 there is an urgent need for effective and affordable tools to facilitate early detection and effective management of pre-cancer not only in the developed world but also in the developing world.
References
1. Society AC: Cancer Facts & Figures 2008. Atlanta: American Cancer
Society; 2008. 2. Anderson G, Boyes D, Benedet J, Le Riche J, Matisic J, Suen K, Worth A, Millner A, and Bennett O. Organisation and results of the cervical cytology screening programme in British Columbia, 1955-85. Br
Med J (Clin Res Ed). 1988 April 2; 296(6627): 975–978. 3. http://whqlibdoc.who.int/publications/2002/9241545720.pdf 4. Anderson G, MacAulay C, Matisic J, Garner D, and Palcic B: The use of an automated image cytometer for screening and quantitative assessment of cervical lesions in the B.C. cervical smear screening programme. Cytopath 8(5):298-312, 1997. 5. Lam S, Kennedy T, Unger M, Miller Y, Gelmont D, Rusch V, Gipe
B, Howard D, LeRiche JC, Coldman A, Gazdar AF. Localization of bronchial intraepithelial neoplastic lesions by fluorescence bronchoscopy. Chest 113:696-702. (1998). 6. Poh C, Zhang L, Anderson D, Durham S, Williams P, Priddy R, Berean
K, Ng S, Tseng O, MacAulay C and Rosin M: Fluorescence Visualization Detection of Field Alterations in Margins of Oral Cancer
Patients. Clinical Cancer Res. 12(22):6716-6722, 2006. 7. Poh C, MacAulay C, Zhang L, Rosin M. Tracing the “at-risk” oral mucosa field with autofluorescence: steps toward clinical impact.
Cancer prevention research. 2009;2(5):401-4. 8. Boyle P, Levin B: World Cancer Report 2008 IARC; 2008.
For more on cAnceR information visit our DRUG DISCOVERY Web Portal at